– Welcome everyone to Wednesday Nite @ the Lab. I’m Tom Zinnen, I work here at the UW-Madison Biotechnology Center. I also work for the Division of Extension and Wisconsin 4-H, and on behalf of those folks and our other co-organizers, Wisconsin Public Television, the Wisconsin Alumni Association, and the UW-Madison Science Alliance, thanks again for coming to Wednesday Nite @ the Lab. We do this every Wednesday night, 50 times a year. Tonight it’s my pleasure to introduce to you Caitlin Pepperell, she’s a professor here in the departments of medicine and also in the Department of Medical Microbiology and Immunology. She was born in Toronto, Ontario, and went to high school there, and then she went east to go to McGill University in Montreal where she studied English literature. Then she went to Queens University in Kingston, Ontario to get her MD degree. She did a residency at the University of Toronto and then stayed at Toronto at the university to do a fellowship, and then did another fellowship at Stanford. Then in 2011, she came here to be on the faculty. Tonight she’s going to talk with us on the topic of how do microorganisms evolve to become pathogens.
As an old plant pathologist, that’s a pretty interesting question to ask. It’s going to be interesting to hear what you have to say from the medical side. Would you please join me in welcoming Caitlin Pepperell to Wednesday Nite @ the Lab. (audience applauds) – Thank you. Thank you. Thanks Tom for the introduction and the opportunity to give this talk, and thank you all for coming. So yes, I’m gonna talk about bacterial pathogen emergence using a little bit of recherche example. Staphylococcus saprophyticus, which, as I said in the introduction to this lecture, I started working on by accident. So I’ll tell you the story of how that happened. But first, I’d like to start with some definitions for those of you who are in different fields, starting with pathogen, what we mean by pathogen and virulence.
So pathogen is a microorganism that causes disease, infectious disease. It’s fashionable nowadays to get away from thinking about this in a binary sense in terms of pathogens and non-pathogens, and we think of it now, or conceptualize it more along a spectrum, with some organisms having a very high pathogenic potential, like the agent of plague and others being more occasional pathogens, and we might put something for those of you in the plant world, I know there are a few of you here, pseudomonas might be in that category where it’s an occasional pathogen of humans. So pathogen refers to the organism and virulence refers to the trait. So virulence is the capacity to cause disease. I’m going to be talking about bacterial genomics, so just to introduce you to the concept of the genome, which is the total set of genes encoded via bacterium, and bacteria being the nifty little creatures that they are, they have, or can have, one or more main chromosomes and little accessory packages called plasmids. They can have more than one of these, and they can also deliver proteins to their host cell, and this is just a little schematic of the bacterial cell. This is a very simple phylogeny, which is a family tree, so it is a depiction of relationships among organisms, and here we can see that A and B are more closely related to each other than either of them is to C. And I’ll be showing you some phylogenies as well. I’m also gonna be talking about core and accessory genes in bacterial genomes; this is how genes are often drawn or represented abstractly. And the concepts behind core and accessory genes is very simple.
Core genes are always present in the population, and accessory genes may or may not be present. So hopefully I’ve done a good job explaining this and you can keep it in mind as I go through the story. So one of the things, overarching questions that I’m interested in in my research and that we work on as a group in my research group, is this question of where pathogens come from. So microorganisms are everywhere, bacteria, fungi, viruses, parasites, et cetera, but disease in humans and in other host species is attributable to a teeny tiny fraction of those microorganisms. So one of the overarching questions that we’re interested in is the how and why of how microbes adopt this particular lifestyle and cause disease in humans and other hosts. So some of the underlying questions are, what is the function of virulence? So in some cases, virulence or the capacity to cause disease in some way directly benefits the microorganism. In other cases, it’s a byproduct of other things that are important to the bacterium. We’re also interested in mechanisms of adaptation to the pathogenic niche, and particularly in genome, genomic mechanisms, so how genomes change when microorganisms, particularly bacteria in our case, adapt and evolve to invade the pathogenic niche. So I’m gonna start with a story about some mystery nodules, these are pictured here and this picture was taken on my desk shortly after I started my faculty position at UW-Madison. William Aylward is a Professor of classics here at UW and he was the deliverer of these nodules.
He brought them to me to study because I’ve done a lot of work on the evolution of tuberculosis, and he thought that these perhaps represented calcified tubercles, which are, it’s infected lung tissue with microbacterium tuberculosis. And Tom mentioned the field of molecular archeology, Doctor Aylward was really interested in developing this field here in Madison, and he thought that perhaps these nodules harbored some DNA from microbacterium tuberculosis, and that by studying this DNA, we might learn about the evolution of TB. So where did the nodules come from? They came from a cemetery dating to the late Byzantine era in Troy, the ancient city of Troy in Anatolia, in what is currently Turkey. And this is a picture of the cemetery. The nodules were spotted by a very sharp-eyed archeologist, she’s actually an archeologist and a physician to whom I am indebted. And this is Doctor Kiesewetter who’s pictured here. So the nodules, as you can see, they’re a couple of centimeters in size, and she picked them out from the rubble in the remains around this individual. So the person here was dated based on archeological evidence from the cemetery to the early 13th century. Doctor Kiesewetter did an analysis of the people buried in this cemetery, who evidently had a fairly difficult and short life on the whole, so the life expectancy based on her analysis was 32 years for women and 39 for men. 50% of the decedents in the cemetery had degenerative changes in their spine and joints, indicating that they were engaged in difficult, probably agrarian labor.
The person whose remains she studied was sexed as female and aged at approximately age 30. She lost many of her teeth before her death at this young age, indicating again a short and difficult life. And she had a healed arm fracture. Carbon dating of the remains was consistent with archeological analysis of the cemetery and dating to the late 12th and early 13th century A. D. So I was tasked with making a diagnosis of the nodules and the person from whom they derived, and myself and collaborators did this on the basis of analysis of DNA that we extracted from the nodules, as well as the other methods that are listed here. Metagenomics refers to analysis of the mixture of microbial species present in a sample and currently is done, it’s a very active field of research in analyzing, for example, the intestinal microbiome or the bacteria that we harbor in our gastrointestinal tracts. We also did a microscopic analysis of this tissue and an elemental analysis, so analyze the elements present in the nodules. In this type of work, in the ancient DNA field, it’s really like finding a needle in a haystack, so most of what is extracted when we extract DNA from archeological remains is contamination. So when we identity bacteria and other microorganisms, it’s most abundant soil material.
So it’s typical to find less than 1% of your target DNA in your sample. Here we found a rather astonishing amount of target DNA. So there are two numbers listed which refers to the two different nodules, so in one of the nodules, without any enrichment or filtering, 12% of the endogenous DNA was human, 31% was from this bacterium Staphylococcus saprophyticus, 4% from Gardnerella vaginalis. So an absolutely stunning amount of endogenous ancient DNA. And not tuberculosis, which is how I accidentally started working on Staphylococcus saprophyticus. Based on this really abundant endogenous ancient bacterial DNA, we were able to assemble a complete genome of this 800-year-old Staphylococcus saprophyticus, with approximately 300-fold coverage, so the genome was covered– that means the genome was covered 300 times approximately in its entirety. And this was, at the time, and I believe still is, the best preserved example of an ancient bacterial genome, so it was quite a stunning and lucky finding. What you see here are scanning electron micrographs of the nodules, and what you can see here are cocci, so these are small round bacterial shapes in abundance, over here we have extracellular matrix, and what we think is the shape and outline of a white blood cell. I showed this picture at a talk I gave at the University of Illinois, and one of the members of the audience who works on Staphylococcus aureus told me that this looks just like an SEM or a micrograph of a living Staph. aureus biofilm, which is a complex bacterial structure.
So this is in fact the 800-year-old tissue, and what’s been preserved here are the shapes of the bacterial cells, the shapes of the inflammatory cells, and the shapes of the extruded material, which we call extracellular matrix, that binds these cells together. So the individual cells and their entire architecture of the tissue was preserved in this sample. The diagnosis that I settled on in the end, based on the microscopy, the ancient DNA analysis, elemental analysis, metagenomics, et cetera, was the diagnosis of chorioamnionitis. This is a severe pregnancy complication, so it’s an ascending infection in which typically several species of bacteria from the vaginal microbiome ascend and infect the amniotic membrane surrounding the fetus, the placenta, the amniotic fluid, and the fetus. As you can imagine, this is associated with pregnancy complications with poor outcomes for the fetus and can be associated with major morbidity and mortality in the mother as well. And that was almost certainly the case here. We found by looking at different tissues from different bones, that she was likely bacteremic at the time of her death, meaning that the Staph. saprophyticus had invaded her blood stream and caused a septicemia. We think that the nodular tissues represent calcified placental abscesses and that the process of calcification that occurred during this infection is what preserved the bacterial cells, the tissue architecture, and ultimately, the DNA. So one of the things that happens during placental development is there is flux of calcium through the placenta to allow mineralization of the fetal bones, and so placentas are prone to calcify, they calcify spontaneously, and also as a result of inflammatory and infectious conditions.
So we think that what happened here is that there was an abrupt inflammatory calcification that basically froze this infection in time and preserved the bacterial cells, inflammatory cells, et cetera. The placenta is an organ that’s jointly made by mother and fetus, and in this case, we were actually able to recover DNA from a male fetus from this tissue, which was quite amazing. So Staph. saprophyticus, this 800-year-old infection is actually quite unusual in our understanding of this bacterium today. Staph. saprophyticus is familiar to clinicians and microbiologists more as a cause of urinary tract infections and much milder forms of infection than this woman had. It is a member of the gastrointestinal and genitourinary microbiomes, so these complex microbial communities, from which invaders can emerge and cause things like urinary tract infections. It is second only to E. coli as a cause of UTI in reproductive aged women, so it’s quite a common cause of UTI, and urinary tract infections are associated with a very significant global health burden, so hundreds of millions of cases per year, and a recent estimate of cost associated with UTIs in the U. S.
in the billions of dollars. So perhaps in most cases, you know, we think of it as a minor nuisance, but it’s a very major public health problem with a lot of costs related to both the care and treatment of this infection, and also loss of productivity, et cetera. But there’s a bigger picture to this bacterium. So it’s pictured here streaked on a plate, and the images here show multiple niches from which we can obtain this bacterium. So it’s found in meats and cheeses. I read a paper recently about isolating Staph. saprophyticus and other coagulase-negative Staph. from a range of different meats and cheeses in France, and I’m convinced I must go there to further pursue this lead. [laughter] This bug is in yummy foods. It’s also isolated from– can be isolated from the environment, so it can live freely in the environment, and it’s typically been found on farms and agricultural settings.
It’s also isolated as a pathogen and a commensal or innocent resident of animals, and most of the information that we have is from agriculturally important animals, which may just be an issue of those are the animals that we sample most frequently. It’s found in dairy environments, so on belt environments, in milking equipment, and it does cause disease, it causes mastitis, which is an infection affecting dairy cattle, so it’s also a pathogen of animals, including cattle. Some work has been done to try to understand why Staph. saprophyticus is a pathogen and what helps it particularly adapt to, most of these studies have been focused on the human urinary tract, and this is a list of some known virulence genes, so genes that are thought to enable this organism to invade the human urinary tract. For urinary tract infections, one of the most important traits they need to have is they need to be able to bind to human cells and to the matrix that connects human cells. So one of my colleagues who works on E. coli that causes UTIs gave me an analogy of the bacteria having to hold on to slippery rocks as a waterfall cascades over them. So there’s major physical sheer force applied to bacteria that try to ascend the urinary tract, and the ones that stick around and win have to be able to really stick well. So many of these virulence genes are adhesions or they encode adhesions, which are proteins that allow the bacteria to really rock-climb up the urinary tract. As part of the analysis of this ancient bacterial genome, we did a phylogenetic analysis, or looked at relationships among different types of Staph.
saprophyticus, and at the time that we had this amazing ancient bacterial genomes, there were almost no other fully sequenced genomes, so as part of that project, I went knocking on doors with collaborators around the world to try to find some more isolates of this bacterium. And the goal of this work was to place the ancient genome into a context and also to understand the evolution of this bacterium and its genome structure. And what is pictured here is a collection of genomes that we had at that time, and then these are the areas that we obtained them from, and then we’ve got some cute little pictures to show you where they came from. So you can see from fish, from meat, this was from a river in Malaysia, and then we had a doggy, and a cow, and our guy from Troy here. So, consistent with this organism being able to live in multiple niches and environments. What’s shown over here is a different kind of family tree called a network, and this shows the relationship between the plasmids, so these are those little autonomous pieces of DNA that we got from different bacterial genomes, and we looked at how they were related to each other. And one sort of interesting thing that came out of this analysis of the plasmids particularly, is we’ve got this major clade structure in the core genome that is recapitulated on the plasmids, suggesting that the bacteria can’t exchange, from these two clades, can’t exchange plasmids with each other, which is, at this point, an unsolved mystery. So, in follow-up work, we looked at a larger sample of Staph. saprophyticus genomes, focused on the question of whether there’s any specific adaptation, any signature of adaptation in the genomes to the human urinary tract. And this work was led by Tatum Mortimer, who’s pictured here as a former graduate student in my lab and is now a postdoc at Harvard.
So one of the really interesting things about Staph. saprophyticus UTI that’s emerged from the epidemiological literature is that this infection appears to be acquired from the environment, so as opposed to spreading from person to person, it seems that people acquire it from the environment and then it causes UTI, and then they shed the bacteria back into the environment. So our question was whether this represented, human UTIs represented a spillover or whether this could be an adaptation. So whether we happened to acquire this bug from the environment, it’s really adapted to do something else, versus it really has a specific adaptation to humans. So one clue that supports a specific adaptation is we found the UTI associated isolates were clustered together on the phylogeny, so the bugs that cause UTI are more closely related to each other than they are to bugs that were obtained from different environments and niches. Tatum did some further work looking at patterns of variation to look for signatures of adaptation to human urinary tract infections. So one of the things that we did is a genome scan, which is pictured here. And what we’re looking for here is a dip in levels of diversity as we move across the genome. And the reasoning behind this is fairly simple. So when you have an advantageous mutation, it spreads through a population quickly and it tends to pull linked DNA next to it as it rises in frequency, and so the population at that particular locus where the advantageous mutation is will have low diversity relative to other parts of the genome.
So Tatum found this dip in diversity, this location, and within this dip, found a mutation in a gene called Aas, that was strongly associated with human urinary tract infection. And this is a protein-coding mutation, so it changed the code of the protein, as opposed to what we call a silent mutation. The Aas gene is one of the virulence genes that I listed at the beginning of the talk. It is an adhesion, so it binds to a human material called fibronectin, and the mutation was in the fibronectin binding region of the protein. We looked at patterns of gene exchange between organisms in this sample, and we found several examples of transfer events where the bugs swapped out one version for another, and each of these transfer events reinforce the association between the one variant and human UTI, supporting its role in mediating adaptation to causing UTI. Tatum also did some demographic reconstructions from the genomic data. So this is figuring out whether populations have stayed the same size, have grown, have shrunk, and she found evidence of a bottleneck, which is a sudden decrease in the size of the population followed by a 15-fold expansion. So this is consistent with what we call a selective sweep, where an advantageous mutation sweeps through the population, decreasing diversity, and then the population expands into a new niche. So we’ve followed up this work with Madison, who is in the audience now, and a graduate student in my lab, and ongoing studies to follow-up these observations are on the larger sample of 165 genomes. These are from several different types of sample and host, and from, you know, it’s not a perfectly representative global sample, but a quite diverse one.
So we start with the phylogeny, and it’s a much larger sample, it’s a bigger phylogeny. We’ve got the same clade structure, so we’ve got, this is one clade, and then this whole thing is another, so as we’ve added to the sample, one of the clades has expanded to a much greater extent than the other, suggesting that the places that we sample are dominated by this clade. I have a note here about no geographic structure, so what this means is that we have isolates from Madison, Wisconsin, that are interspersed with isolates from Brazil and Japan, so they’re more closely related to isolates from Brazil and Japan than they are to other isolates from Madison, Wisconsin, which is pretty remarkable. As I mentioned, clade one is more common, so this is the one that grew really dramatically, and then clade one has a really interesting sub-clade structure. So this sub-clade up here is almost exclusively human UTI isolates. There’s one exception of an isolate from a dog, and most of this kind of infection in dogs represents spillover events from humans to their pets, then we have an environmental isolate here, but aside from that, we have all human UTI. This sub-clade, on the other hand, contains human UTI, bovine mastitis isolates, and food isolates, so it’s consistent with some perhaps different ecologies in these two sub-clades, one of which is more strictly associated with humans and the other with food and agricultural animals. Madison did another genome scan with this larger sample to see if the patterns that Tatum had identified with a smaller sample would be replicated, and very nicely, they were, so this dip here in diversity corresponds to the dip that Tatum had identified in the smaller sample, and supports the idea that this region, and particularly the gene Aas, is under selection in this sample. So one of the possibilities to explain, you know, why a particular strain causes UTI versus ending up, you know, as a commensal of cattle is that, perhaps the ones that caused UTI have virulence genes and the other ones don’t. And there was, if you recall, I mentioned that there was that list of virulence genes that had been identified previously.
There is no support for that hypothesis, so what we found, what Madison found, is that, so these are a list of virulence genes here and here, and these genes tend to be either present in the entire sample from all different niches and environments, or they’re present in a really small fraction of samples, so we can’t map the presence of these known virulence genes onto human UTI and use that as an explanation of UTI. One interesting example is this gene dsdA, which is D-serine deaminase gene, and what this does is to detoxify D-serine, which is found in mammalian urine, and in studies of this gene, the idea is that this is a really important protein for bugs that want to live in the urinary tract. Well, this gene is found in the entire sample of bugs from all different niches, and Madison has looked at other bacterial species to see if they encode this gene, bacteria that don’t have anything to do with the urinary tract, as far as we know, and it’s encoded by all kinds of different bacteria. So what this suggests to us is that the gene has multiple functions, and this is very typical for bacterial genes, so I like to think of them as Swiss army knives, they typically do many different things. And so the concept that this is specific to adaptation to urinary tract just reflects our own biases in how we look at these genes and proteins, and think about them. So Madison has done some further work to find genes that are associated with human urinary tract, and also with bovine mastitis, and in addition, to look for variants of genes, so mutations that are associated with these different niches. She has found some accessory gene contents of some genes, and some variants that are associated with UTI and that are good candidates for adaptation to the pathogenic niche. That’s the good news. The bad news, or slightly more difficult news, is that the genes that are associated are poorly characterized, most of them are hypothetical proteins, and they don’t have close cousins in other bacteria, so they’re. . . difficult to know exactly what they do, and it’ll be a lot of work to figure that out.
We’ve also had a look at the plasmids found in the sample, so those little autonomous bits of DNA, and these, what’s pictured here, are assemblies of plasmid genomes based on a different kind of sequencing technology, so a type of sequencing that allows us to look at very long stretches of DNA and resolve these structures. Madison has found a lot of diversity in terms of plasmids, from bacterium to bacterium, so between one and five plasmids harbored by the bacteria, some of them are quite large, up to 100,000 base pairs, some of them have apparently completely novel gene content on them, and she’s also found that virulence genes have been mobilized on these plasmids. So the plasmids are giving the bacteria an enormous amount of flexibility, and there’s reflected in a lot of diversity in terms of what the bacteria can do. So, to come back to my original question of where do pathogens come from, this bug, Staph. saprophyticus, is found as I said in humans, animals, indoor, outdoor environments, and food, and when we look at human infections, some of them do appear to be spillover events, so those are the organisms that are on the parts of the tree that are not pure human UTI, but many of them appear to reflect a specific adaptation to the human urinary tract. And this is on the basis of genome reorganization, so it’s on the basis of new mutations that have arisen, mutations that are swapped from one bacterium to another, and entire genes that are swapped between bacteria. So I’ll end there with my acknowledgments. This is the Pepperell lab, where we are completely serious at all times. [laughter]
And I’d like to thank the members of the Pepperell lab, particularly Tracy, Lindsey, and Nathan, who are directly involved in generating the data that Madison analyzed. And my funders at the NIH and NSF, and collaborators at UW-Madison and off-campus, and thank you for your attention, and happy to take questions. [applause]
Search University Place Episodes
Related Stories from PBS Wisconsin's Blog

Donate to sign up. Activate and sign in to Passport. It's that easy to help PBS Wisconsin serve your community through media that educates, inspires, and entertains.
Make your membership gift today
Only for new users: Activate Passport using your code or email address
Already a member?
Look up my account
Need some help? Go to FAQ or visit PBS Passport Help
Need help accessing PBS Wisconsin anywhere?
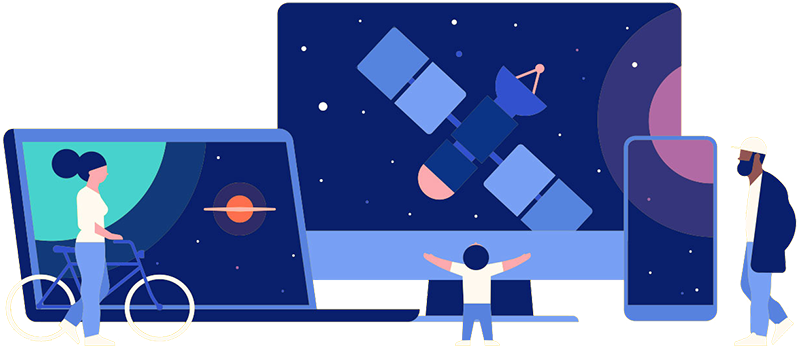
Online Access | Platform & Device Access | Cable or Satellite Access | Over-The-Air Access
Visit Access Guide
Need help accessing PBS Wisconsin anywhere?
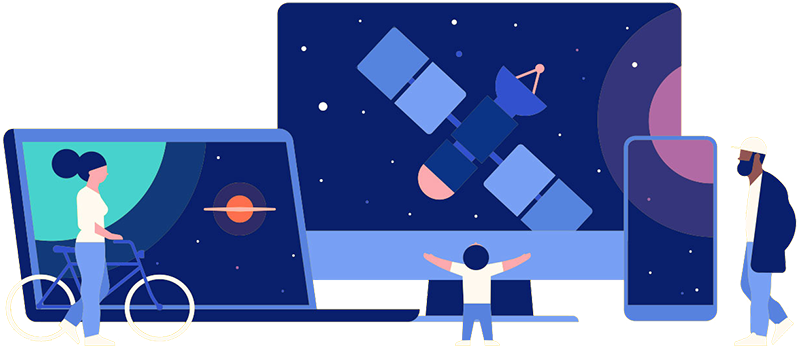
Visit Our
Live TV Access Guide
Online AccessPlatform & Device Access
Cable or Satellite Access
Over-The-Air Access
Visit Access Guide
Follow Us