– Welcome everyone to Wednesday Nite @ the Lab. I’m Tom Zinnen. I work here at UW-Madison Biotechnology Center. I also work for the Division of Extension and Wisconsin 4-H, and on behalf of those folks and our other co-organizers; PBS Wisconsin, the Wisconsin Alumni Association, and the UW-Madison Science Alliance, thanks again for coming to Wednesday Nite @ the Lab. We do this every Wednesday night, 50 times a year. Tonight it’s my pleasure to introduce to you, Daniel Ludois. Daniel was born in Beloit, Wisconsin on the bonny banks of the Rock River, as was I. He went to high school at Hononegah High School in Rockton, Illinois. So he went over to the dark side for high school. Then he went to Bradley University in Peoria, Illinois, where he studied physics.
Came back to Wisconsin to get his PhD at UW-Madison here in electrical engineering. In 2013, he joined the faculty here in electrical and computing engineering. In 2015, he won the Career Award from the National Science Foundation. And in 2017, he was awarded a Moore Inventor, and that’s as in the Moore Law person, so that’s a pretty great accomplishment. Tonight he gets to talk to us about something near and dear to all our hearts, Electric Machines: Then, Now and the Future. He specifically is gonna talk about electrostatic machines, and I have never heard of those, and I’m looking forward to the yin of electrostatic to the yang of electromagnetic, so I think it’ll be great stuff. So please join me in welcoming Daniel Ludois to Wednesday Nite @ the Lab. [audience applauding]
– Thank you very much. [audience applauding] All right, good evening everyone. So I’m Dan, and for the most part, this talk is broken into a few different segments.
So I’m gonna try and cram 250 years into the first segment. And then for the middle segment, we’ll talk about what we do now. And for the third segment, we’ll look to the future a little bit, and what my lab’s doing specifically here at UW-Madison. So, how much energy do we use as a society? It’s a lot. In fact, it’s so much that if you stand up on a satellite somewhere and look back down at the night side of the Earth, you see that. The surface of our planet glows at night. And so this image is courtesy of NASA. And so around 7% of the electricity on the electric grid goes to lighting, and so you can see that reflected in the night sky above. But that’s just a tiny amount. It’s only 7% of what we use on the grid.
And so when we talk about energy on these scales, we don’t talk about watts anymore, we talk about something called quads. And a quad is a quadrillion BTUs, okay. So the total energy used by the United States in 2016 was 97. 3 quads, quadrillion BTUs. That is, 1 quadrillion is 293 million megawatt hours, and 1. 05 quintillion joules. All right, so that’s a lot. And if you think about that, we have around 97, up to a 100, and then the 7% of that 12%, I’m sorry, of the 12 quads is lighting. And so we’re looking more on the verge of oh, in the ballpark of less than 1% of the energy we use makes the planet’s surface glow at night. Think about that.
So if you wanna get a little bit more specific, this is a diagram that shows where our energy comes from and where it goes. So over here on this side, this is where our energy comes from. So yellow is solar and red is nuclear and so on, and then down here the fossil fuels. And the amount that we use is proportional to the width of these lines, and this is where it goes. And so over here we have residential, we have commercial, we have industrial, we have transportation, and then we have electricity generation. So notice that at the end, the amount of energy that actually went out and did the work that we wanted it to do is here. And on this side, we have what’s called rejected energy. This is the energy that never made it to do anything, but we used it. And so that comes out to be about two-thirds, so about two-thirds of all of the energy we produce is wasted in total. So what are the biggest culprits? So if we break down the losses, we see transportation, we see electricity generation, and then here’s our homes and residential, here’s commercial, so shops and stores and things, and here’s industrial.
And so in all these sectors, these are our biggest culprits of waste, so it’s mainly electricity generation and transportation. What do we learn from this? So if 70% over that, 71, of all losses come from generation and transportation, well, we should put some focus on that, give it some attention. And so we have a few options. The main reason that we have all this loss is because we burn fuel, and it’s hard to get around the second law of thermodynamics. So we have two options: Option A is we can stop burning fuel and then we go to a generation solutions for electricity or solar, wind, ocean, geothermal, et cetera, processes where we don’t burn anything. Second one is transportation: Same thing, electric vehicles, electric aircraft, or option B is to be better, smarter about how we burn things. So this would be for generation, this is combined cycle plants, this is using the waste heat over again to produce more electricity. The other option is, in transportation, is things we’re already doing, things like hybrid vehicles, hybrid aircraft and the like. And so my research, what I work on, is something called electric machines. And electric machines have to do with things that move.
And so in my case, I’m striving towards enabling technologies that will make the things that move, like wind and ocean and transportation et cetera, possible. So you’ll hear me calling out electric machines. So what is an electric machine? So we don’t call motors and generators, motors and generators in the trade as it were. There’s a reason for that. So an electric motor converts electricity into motion, and that we call a motor, but if you put motion into one of these things, you get electricity, and so it’s the same object, and so we have a generic term for that, and that’s called an electric machine. And so we don’t call it a motor or generator, because any motor or generator, with very few exceptions, can be either. Electric machines enable our future, so some of those topics that I was telling you about are framing from a perspective of the impact that we have on the world that we owe to ourselves going forward. These are some of the fun ones. So everyone’s seen one of these. I actually have, I think a neighbor of mine has a teenager that’s flying one of these over my house all the time. [audience laughing]
And then for the Star Wars fans in the room, you can cruise over the moons of Endor in one of these. And this is a hover bike. This has got propellers built into it, and so this is a concept that’s coming out. Electric cars, we’re already more familiar with, and everyone, I’m sure someone in here owns a Tesla or a Chevy Bolt or Volt or any of the like. But the next generation of them may have motors built into the wheels, making them even more attractive. The race is on for electric aircraft, and that’s got numerous hurdles for it. But also, we have the robots that look like they’re going to come for us one day. These are made, this one in particular is from Boston Dynamics. And then sources of generation, so this is the basic wind turbine and the cell structure; it’s the box at the top of the tower. And right now, we have this big gearbox that matches this low-speed blades to this small generator, and this is a source of reliability for them.
So future wind turbines, and they’re experimenting with this, and somewhere out in the field or with what are called direct drive, there’s no gearbox of any kind. And then likewise down here, we’ve got ocean and tidal energy, where we try to extract energy from the slow movement of the rise and fall of wave and water. And so all of these things at some point have to take motion and turn it into electricity, or take electricity and turn it into motion. Okay, so there’s a whole bunch of challenges associated with all those applications; we’re gonna get to that. But before we can jump into the wind turbines and the oceans and the robots and the hover bikes, I need to explain how an electric machine works. And for an audience to appreciate what we’re gonna try to do here, okay. So I’m a firm believer that you can learn a lot from a little history. So in this case, we’re gonna start off towards the beginning and we’ll spend a little bit, and then remember, I mentioned we’re gonna do 250 years in the first segment, all right. So in 1820, there was a gentleman named Orsted, and he discovered that an electric current moves a compass needle. He was doing an experiment; there was a current flowing through a wire on his bench, and he had a compass sitting on the bench, not for any purpose of the experiment; it was just there.
And then he noticed when he turned his experiment on, the compass needle moved. And so that was how we discovered that electric currents make magnetic fields. We all knew, scientists at the time knew, that if you had two magnets, they would attract or repel and all that basic stuff, but no one knew until that point that an electric current can make a magnetic field. And so that’s what Orsted did in 1820. That same year his colleague, Ampere, you might recognize his last name, the amp, he refined Orsted’s observations and figured out the relationship between angles and magnitudes of currents and things like this. And so, and this is something that is the, you know, the bane of physics students in high school, and this is something called the right hand rule. And what the rule means is that an electric current will have a force upon it that is orthogonal to both the current and the direction of the magnetic field. And so in this case, if I take my right hand and I point my fingers in the direction of the current, and then I curl my fingers in the direction of the field, my thumb points in the direction of the force. So if I stand this way and do it, it should work. Current, field, force.
And so it’s always kind of fun during test time, everyone’s like writing and doing this, but so anyway, this is the relationship between a current and a magnetic field and the force that happens. All right, the next thing we need to know in order to understand how a motor works, is we need to know about Ohm’s law. So I’m gonna keep this presentation very math-light, so I know that, I mean some people love math, other people don’t, but for those in the room that don’t, this is one of my favorite cartoons. So how Ohm’s law works, or rather what it states, is that if you have some voltage, voltage pushes current through a wire, and the resistance of the wire tries to pinch it off; it stops it. And so the old analogy of the water hose, the voltage is the pressure pushing the water, the volume of water is the current, and the diameter of the hose is the resistance, okay. And so the relationship between the three is that voltage is the current times the resistance, and that’s what we’re having to work with. So, and then electric power is simply current times voltage. How much current is flowing, or how much water is flowing, and how much pressure is behind it. That’s power, okay. So good conductors are things like silver, copper, gold, aluminum.
Poorer ones, insulators, are glass, mica, plastic, rubber. And why all this matters will become apparent. Next thing you need to know in order to understand how a motor works is: What is torque and what is mechanical power? So torque is rotational force. And so you can see here you have a certain amount of torque. It can be applied to a shaft if you have a force that is tangential to some circle acting on a radius. And so in this case, there’s a torque about the shaft, because you’re applying a force tangential to that disk, and so that’s rotary force. And so you might hear a car engine has so many foot-pounds and things like that. It’s the amount of force acting at a distance. And so that’s in Newton meters or foot-pounds. The product or the power of something is the torque that is being exerted and the speed at which the shaft is rotating, okay.
So mechanical power, so if you have a car that’s got a really powerful engine, that engine will have a lot of torque, and it’ll have a really high RPM range, okay, so if you’re thinking of your car. All right, so those are some basic concepts that we’re gonna need for motors for the moment. So I made a little demonstration in my workshop at home, and I tried to make it look period-appropriate, so it’s got a cherry base and a few other nice things, but mostly some brass here. My students tease me that I have an affinity for brass and walnut. So in this case, this is a pendulum. At the end of this pendulum, there is a coil of a thousand turns of wire. And this big black piece of cast iron here, this is a magnet, a permanent magnet. And so what I’m able to do, and there’s wires that run up the through the pendulum and then up through the top, and I can connect those down to either a battery or a meter or whatever I like. So in this case, what I’ve set up is I’ve got a voltmeter that’s across my coil, which is inside this magnet here at the ends of the pendulum rod. And then this meter here, this is measuring current that’s going through the coil.
So this is the voltage across the coil and the current going through the coil. All right, so I applied a voltage to the coil, and if you look down here, a current is flowing through it, and when the current’s flowing, the coil swings out of the magnetic field or away from it. I’ve offset the coil a little bit in one direction, so it prefers to go one direction than the other. But this is the basic thing that Ampere and Orsted saw. And then Ohm described the voltage in the current. If we look a little bit more closely at this, you can all practice our right hand rule. We have our current flowing here, we have our B field crossing the current, and our force pushing that current segment out of the magnet. Also note that we have a certain amount of volts, a certain amount of amps, and so there is power developed or not developed, but being dissipated in this coil. And so this is the resistance of the coil. To push current through it requires a certain amount of electrical power, but the mechanical power is zero.
We’re putting electricity into this thing, but there’s no mechanical output power. The coil is deflected, but then it just stays there. It’s not moving continuously, and if it’s not moving, then there’s no mechanical power, because power is the product of speed and torque. So this brings us to Michael Faraday. And so how do we get continuous mechanical motion? The conductor needs the right trajectory. So in this case, how do we design something where the conductors can always move to develop power? So Michael Faraday was at the Royal Society, and that was where he was employed. At the time, he was the assistant to Sir Humphry Davy. His boss at the time was one of the most, if not the most famous scientist in the world, and everyone was trying to figure out: Well, how do we go from this force to a continuous motion? And actually it was Faraday who did it. And he didn’t do it with any kind of rigorous theory. He did it through tinkering and intuition.
And then later on, 10 years after getting the motor to spin in 1831, he did the law of induction, which was what is needed for the motor to work, but he didn’t know why. So what is this Faraday’s law? And so Faraday’s law says that if you have, in this case I have a loop of wire, and this loop of wire is going, moving through a magnetic field. This magnetic field then, its lines of force, as Faraday called it, would cut through this, and so there’d be a flux that builds up as the coil enters, and then the flux builds down through the coil as it leaves. And so you can see as the flux is increasing, there is a voltage that’s proportional to the rate of change of flux, that’s called the derivative for the folks who know calc, and then as it leaves, there’s a voltage in the opposite direction. This negative sign states that, that’s credited to Lenz, that the back EMF, the voltage that develops is opposed to the change in flux. It’s trying to keep its flux constant, but it can’t. So a change in flux makes a voltage. So what does that look like? So I’ve set up my same pendulum that I’ve had before, and in this case, I’ve put in a voltmeter just measuring the voltage on this coil. Now this good-looking hand is gonna come in and displace that coil. And as it swings through the field, notice that it’s mimicking what that image was in the last slide.
Also note that the voltage is changing at twice the frequency that the pendulum is swinging at, and that’s because the flux enters and leaves in a single swing, and so you get that double frequency. But that change in flux creating voltage is what Michael Faraday is credited for, and that’s why he has Faraday’s law. So what he came up with was the following device, and this is considered the first magnetic motor. So here we have a wire that goes down into a cup of mercury. And over here, we have a wire that also goes down into a cup, and this large black bar is actually a bar magnet. And so what he figured was, he could get continuous motion if the wire orbited around the magnet. It would always be trapped in its field, always continuous motion. I did not replicate giant cups of mercury [all laughing] for a variety of reasons, whether it’s safety, preservation of my marriage, other things. But in this case, I decided to do it in a way that’s a little bit more acceptable. And so what I’ve done here is something that anyone can do at home, and I figured I’d just do a quick tutorial about it.
So get yourself a bar magnet, a cylindrical one, and then stick it to the bottom of a double A or a C cell, in this case, battery. And then get yourself a piece of copper wire. And this copper wire you bend into a square, but this end of it is open. You set it on top of the battery, and then the current will flow out and it makes contact with the magnet at the bottom. Current will flow out of the battery, split and go either way and go down, contact with the battery, and flow back up in. The flux, the lines of force from this magnet, come out and around and circle, and they cut through these little wires as they go down the side of the battery. If you do your right hand rule; current down, flux in, you can notice that there is a force developed that’s pushing the wire into the screen here, and then since the flux is going the opposite way on this side, out of the screen. That’s gonna create a torque that’s gonna twist it, and that is at a radius, so that’s torque. And then, indeed, it works. And so what took people lifetimes and many things to do, you too can do on a piece of paper in five minutes.
But that’s one thing I always love to tell students, is that we try to cram into their heads in a half hour what took other people that we think are legendary a whole lifetime to do. So don’t feel bad. But in this case, we’ve got, I’d also like to talk a little bit, just very briefly, about some of the math. So in this case, we have a battery. That battery is pushing current through the resistance of the coil, and then the rotary motion of the coil that gives out voltage from that Faraday’s law, and so we can describe that the output power of this motor is equal to the current flowing through the coil, and the voltage induced by Faraday’s law, and that’s equal to the torque and the speed out. Notice that we can never get out more than what we got in. There’s conservation of energy in play here, so you’ll always get out less. You have to subtract off the losses due to your copper windings for having resistance. But that doesn’t really look like a motor that we use, does it? So we’re gonna get in a little closer. So what we do is, instead of having copper wire orbit around batteries, instead what we do is we make loops and we put them in a field in this configuration.
And then the same principle though of the current flows through the wire, there’s a force on it in this direction up, this direction down, causes it to rotate. However, when it starts rotating from one side to the next, it will experience a torque in the opposite direction, and it wants to flip. There’s something called a commutator, you always have to keep the current in this side of the motor going this way, and the current on this side of the motor going the other way. ‘Cause if it rotates and it flips over, the current directions will flip too, unless you have something that’s changing it. And this is what we have here. So if this went all the way over and rotated around, this current, with those little arrows pointed this way, would now be going the opposite direction. So we have these bars, and it’s much like a old telephone operator, when something needs to get changed, you gotta switch it, you plug it in somewhere else and so notice, as it flips over, the contacts flip as well. Similarly, we can add more turns to it and get better torque. More turns is better. And so, if you were to make something that looks pretty realistic, we’d wrap or put our windings inside our magnet like this.
And then this is what I was calling the commutator, and as it spins, these currents, as you can see here, always get maintained, so the current goes into the page or the screen on this side and out of the page on this side. And these contacts that are over here always ensure that that happens, so you get continuous torque and motion. All right, so the traditional DC motor. So this is a starter motor or a similar kind of motor that you might find on a lawnmower, okay. And you can see if I take it apart, you see the magnets, you see the coils, you can see the coils embedded here. Here’s the commutator bars. Here’s the brushes. And so that cartoon I just showed you is pretty accurate reflection of real life, and then I just crammed a whole semester into you pretty quickly. So someone might say, “Well, what about AC motors? “You showed me a DC motor. ” Well, all I’m gonna say about AC motors is that it’s a DC motor flipped inside out.
We put the AC winding, we put these windings on the stationary part, the part that doesn’t spin. And then we take these magnets and we put them on the rotor, the part that does spin. And so we don’t have this commutator thing anymore, because the commutator part wears out. That’s the part that breaks all the time, ’cause the brushes wear out, it makes dust, it’s friction, it’s sliding contacts, it’s no good. And so modern motors are all AC. And just to give them a shout-out: Tesla, Ferraris, Steinmetz, these are names of people that created this instead of, so the windings, although they’re not rotating anymore, the magnetic field that they generate is rotating, and that’s through the use of what’s called three-phase power. Three-phase power allows us to have a series of coils, one, two, and three, that are all were shifted or skewed from each other. And if you put a three-phase current in them, the magnetic field that they generate rotates in time if you apply that, or in this case, where I’m showing us in space. But so as the these phases go in sequence, you get this rotating field, and so. And we owe that to the folks in the 19th century, mainly Tesla, Ferraris, and Steinmetz.
All right, now if I show you an actual electric car motor, you’re gonna know what all the parts are, okay. So this is one that’s made by GM, and I got this off their media page. This is the stator, here’s all the windings, and then that’s a three-phase winding set, so they put three-phase currents like I just showed you in it. And then here’s the rotor, and it’s full of very fancy magnets that are very powerful, and then iron course. And so everything that we just talked about is buried in this, okay. So some summary, so if you wanna get torque out of an electric motor, you need current and you need flux. If you want speed, then it’s tied to voltage. So torque and loss are at odds with each other. So if you wanna get torque out of your motor, you gotta put current in it, that means you’re going to create heat, because you’re pushing current through windings, that’s I squared R. And so if we wanna make motors more efficient, what do we have to do? We have to add more copper to get the resistance down.
We have to add more magnets or more steel to make the flux go up. But that adds size, weight, and money, and we make things to be cheap, at least now. But the bandage that we apply to fix all this is we use higher speeds and lower torques with gearboxes, so wherever, usually in a car there’s a gear, they put a gearbox on it. You have a hand drill, there’s a gearbox on it. So electric motors are typically higher speed, lower torque; you put gears on it to keep the thing from melting. They also use cooling systems. All right, so optimization, materials, and power they’re trying to control, are the main thrust people that are working on in electric motors today. So we’re throwing computers at, going through thousands of designs to pick out the best ones. We’re working on trying to get superconductors in the role here, so we don’t have that I squared R loss. Carbon nanotubes for the same reason, for making some lower resistance wire, magnets, insulation cooling.
We’re using loss minimization with advanced controls and semiconductors. So this is what we’re doing now to enable the near-term future. But in the end, and if you look at a motor in 1890 and you look at a motor in 2010, are these two pictures. Do you see a similarity? They’re still the same thing. I mean one is– I don’t wanna cheat out millions of person lifetimes’ worth of work over the last hundred and some years, but it’s still the same concept. And so this is actually an Edison machine, and I grabbed this machine out of the storeroom. These two machines are about a 100 years apart, but they’re the same power rating. So we’ve come a long way, but what does this mean? So right now, we are struggling to make some of these applications work, particularly things like direct drive wind, direct drive ocean, robotics, electric aircraft, electric vehicles are doing all right, but we’d like to see a hub motor that’s more realistic. And a lot of this is held back by the electric motor as well as batteries, but if we start looking at all of these things, we have things that we have to figure out. And there’s a common thread amongst them all, and that’s weight.
We gotta get the weight out and also the heat out, and we can’t have it cost anything. [audience chuckling] So this is the life of an engineer. [chuckling] If we look at the sustainability materials, this periodic table is color-coded to reflect the sustainability and availability of the elements projected within the next 100 years. Green is good, red is bad. So that is the best way to describe it. So in this case, that dashed line, everything below that line, so namely copper, iron, nickel, cobalt, samarium, neodymium, and dysprosium are what we use to make electric machines, and they’re all not color-coded that well except for iron, but the other thing about where they are in the periodic table is they’re all really heavy. We don’t make anything out of all this nice lightweight stuff. That’d be nice. So what can we do? Is there a way to make an electric motor out of the things above that line? And so I propose, and my group has been actively working on making electric motors that use electrostatic forces, rather than magnetic. So instead of the force that holds your child’s artwork to the fridge, I’m using the force that holds your clothes together out of the dryer.
[audience chuckling] All right. So if we do that, we can then enable the materials that don’t necessarily have to be magnetic. And in this case, that allows us to be more sustainable and also cost-effective. If we go back here, we can see, “Hey, I can use these “to make composites and/or plastics, “aluminum’s above the line, that’s in play. ” It’s looking good. Has anyone attempted this before? And the answer’s yes. [chuckling] The very first person to turn electricity into rotary motion was Benjamin Franklin in 1748. And what you see here is the motor that he constructed. Everyone says Michael Faraday made the first motor, but that’s actually not entirely true. Benjamin Franklin made an electrostatic one that ran off of him rubbing rabbit’s fur on an ebonite rod as fast as he could, standing next to this thing to get it to go.
As you can see, it is a novelty, and it was a curiosity at the time. He’d pull this thing out for diplomats and start making electricity turn things and needless to say, it was a great party trick back in the 1700s. So, but can we bring this into the 21st century? And so to do that, we have to examine why we failed at it at the past. And so I’ve already discussed these with you. This is what makes our motor go. This is what, if you wanna pick up a car with an electromagnet and drop it off in a junkyard, and this is what we got here. This, these guys down here are the electrostatic forces sort of in a discreet fashion. If you put an electric charge in an electric field, it’ll move in the direction of the field lines. So the field lines point to the point over here. This charge is gonna move over there if it’s a positive charge.
So we’re gonna use that. Is there a way to compare these things? And it turns out that there is. So we can, instead of talking about fluxes and currents and charges and fields, those currents and charges have their own fields associated with them, so we can talk about the energy that they have in space. And so bear with me for a moment here, but if you were to just take a motor and say, “I’m gonna have a part that spins,” that’s a rotor, and “I’m gonna have a part that’s a stator, “that’s stationary, don’t care about anything else. “I got two concentric cylinders. “This part moves, this part doesn’t. ” What you can do, via a lot of math that we’re not gonna talk about, that, if you have electric fields and magnetic fields in that gap, you can use those fields to determine how good of a motor it would be. And so in this case, it turns out that if we use copper, iron, and magnets, permanent magnets, to make a motor, that allows me to have an energy in that gap of 900 kilojoules per cubic meter. If I use electrostatic forces, say with aluminum, it’s about 0. 04, so that’s around 10,000 times less.
This is why we don’t have electrostatic motors. Then illustrate this a little bit more, if I have a magnetic field and I wrap it in iron, which is gray here, and copper coils, which are orange, this little equation here says that for a given field strength, which is measured in teslas, we get that energy density. Similarly, for the electrostatic field, same volume, which you would put between two surfaces, you get 40, so that sucks. All right, so what do we do? So this is where we have to be engineers, rather than scientists. I can have degrees in both, so I get to play. [chuckling] But so the first step is, the thing that’s holding us back is air, so get rid of it. So the reason that you can’t develop much energy density in air with electrostatic fields is, if you build up a charge and you keep building it up, eventually what happens when you touch a doorknob or anything, you get a spark. Or if it’s outside, you get a lightning bolt. There’s only so far you can push air until it breaks down and you get that spark. So we have to eliminate that problem.
And so what do we do? I’m going to displace the air. I’m going to fill my motor with a very fancy liquid. This liquid has the same properties that iron does for magnetism, but for electricity. So if I do that, that buys me a factor of 300. I’m trying to get to 10,000, here’s 300. [audience chuckling] All right, so that’s step one. And so if we look at that, if I fill my motor, other people have suggested a gas, other people have suggested vacuum, but it doesn’t have the same energy storage capability as a liquid, and so magnetic motors will see suffer at low speeds. So we’re not gonna try and compete with them at high speed; we’re gonna stick with low. And so we’re gonna go with this liquid. We’re gonna displace the air with it.
It’s not a fair comparison to say, “Oh, this field strength “in this volume is way more than this one,” because this one, this volume comes with baggage. If you have this volume, you have to invite in the iron that surrounds it. You have to invite in all the coils, the cooling system for it. This is just two pieces of aluminum looking at each other. And so in the same volumes, what if I put a bunch more in? If you wanna do apples to apples I can pack in, there’s another factor of 10. And then step three is advanced manufacturing. So compared to the prior attempts by folks in the past, we now have 3D printing. I can print any shape I want. We have injection molding. I don’t have to make this out of steel and copper, I can injection mold this out of plastic if I wanted to, and just spray some metal on it.
Materials: I have composites, I have voided material, so I can make with my 3D printer, I can make hollow shapes you couldn’t otherwise do. Power electronics, the semiconductor industry has advanced to the point where I can manipulate high voltages at high frequency. And then, the power of the computer that we have now is that I can run through thousands of simulations and get the optimal shape I want. And so what happens is, is that you have this technical roadmap, and so the chessboard that I’m manipulating, against nature if you will, is that we have geometry, we have liquids, we have materials in manufacturing, then we have the power electronics, and if you sum all of these as multiplicative gains, you can get your 10,000 and beat it too. And so this is why I get excited about engineering, because it’s a different perspective. This sounds great, but it’s a lot of work. That is a ton of work. So we’re going up against a 200-year-old industry, a 200-year-old concept. It’s really well-established. And so universities are great at this new idea.
This is where we publish it and this is where it dies. [all laughing] Over here, we have established industry, and this is, well we’re a giant cruise ship, we’ve got all these people on board, and we just gotta keep it going straight, otherwise we’re gonna lose a bunch of money. And then we have here, this is, I call this the innovation impedance mismatch. Some people call this, financially, the Valley of Death, but there’s a variety of terms for it, but this is usually– this is actually most people, when they talk about inventing something new, they always talk about the technical part. But the technical part, as much as we love it, isn’t the only part; there is a social part that is equally challenging. Another way I like to think about this is if you look at the old USDA food pyramid, so I subscribe no nutritional whatever to this, but it illustrates my point. Is that if you had a technology food pyramid, I would argue that energy conversion, from the creation of fire to the food we eat, is at the base, and then we, no particular order, those topics, and then apps up at the top. This is your sweets and the candy. Some computer scientist is gonna get mad at me. [all laughing] But it is a foundational thing, to mess with how we manipulate and use energy, and so therefore it will meet resistance, both socially and technically, just about in every way you can think of.
And so it’s hard to change rapidly. And so between that technical roadmap of all those multiplicative gains and the social part of it, and trying to get it out of the university. That is more than one person could ever hope to do. And so like, if I’m like the composer or conductor of an orchestra, there’s no way music comes to life unless I have world-class musicians, and these are mine. So obviously, that’s me. But I also have a start-up that’s helping to work on this S-curve to transition things out. Because the thing that doesn’t necessarily terrify me, but makes me very conscious, is the idea of spending all this time on something and not being able to get it out into the world. And so to do that, you have to be able to pass the torch, and so this problem is being designed in such a way that I have a team that can handle that. And so over here, this is my UW lab and my students and postdoc, and over here is my startup. And so for me, the Wisconsin Idea means that we have not only the duty, but the ability to transition our knowledge out into the world, and for me, entrepreneurship is one of those paths.
Also, it’s a great way to keep our best and brightest students here in the state of Wisconsin and help build it. And so since this picture, these pictures, were taken in 2017, and since that time, three of my students have joined the startup to continue to carry on the fight. My work is sponsored by NSF and the Gordon and Betty Moore Foundation, Moore’s and Moore’s law, Intel, those folk. And my collaboration with my startup is sponsored by the federal government’s SBIR-STTR program, which is for transferring tech out of universities and into small business. And then our IP is managed and facilitated through WARF. All right, so I’m not gonna go into the details of how I pulled off that roadmap, to all those multiplicative gains with math or anything, but if you’re interested and want to see the gory details, they’re right there, so feel free to dig in on your own. But for the time being, I figured we’d all like pictures better. So a first attempt, and as you can see, here’s some of the characteristics, all the cascaded plates, all inside to get all the area. And then this is inside of a tank filled with a liquid to displace the air, and it didn’t work, but we learned a lot. Attempt number two.
Same thing, our cascaded surfaces. This time, we did it in a different direction and we used different materials and different things, and it worked. This one actually did work, but not as well as we hoped. Onward. Three more attempts. So all of these work, and each one got a little bit better. So this one came first, and then we had this one. This one was 3D printed out of plastic, and then we metallized it. And then this one, this one’s made out of Delrin. This one’s made out of composite materials, and it all gets exploded into a case.
And then from these, and all the knowledge that we generated, we created models, both mathematical and computational, that allowed us to optimize every shape and corner. And these are various curves, where we can follow trajectories of all the various dimensions to always ensure that we get the most force out of it that we can. Next, we upgraded it to be more in line with the conventional technology of today that uses what’s called a polyphase system. Remember when I taught you about how to commutate a motor with AC, and how those rotary fields have come about from those space vector currents? Anyway, we extended that into the electrostatic realm, and so we have our electrostatic charge of plus and minus that orbits now inside these surfaces. And then, if we turn that into a disk, it looks like this. And so we can cascade these disks. This is a rotor. This is a stator. And so the charge that’s trapped on these surfaces on this disk surfs on a wave of potential on this disk. And that’s what these disks look like.
The test that we’d get our rotating electric field out of this, we made a disk and we put it in the liquid, and then you can see we were so excited, we were all laying on the floor watching it. This is me and Baoyun and Graham and Aditya and Justin, but anyway, so we’re laying on the floor, watching this thing. And that orange thing right there that’s actually, you know those kids’ toys where they stack all the little doughnuts up? I stole that from my two-year-old, [all laughing] and then deposited it in here, and so the electric field is dragging it around in this dish. So here’s what the latest model is. And this model closed officially all of the four orders of magnitude, and now we’re trying to go beyond. So this motor here is the world’s first aluminum and fiberglass electric motor that has the equivalent performance of a magnetic one. But it doesn’t use any of the materials that it has. You can see here, so this is all retained inside of a tank. We have the cascaded disks. All those disks have the poles and the polyphase system.
It’s been optimized. And this is what it looks like. So the neat thing about this motor is that to look at it, if you don’t know any better, it just looks normal. It fits in with the crowd, and that’s surprisingly a good thing. But if we were to look at it, gone is the circuit where you have motion creating voltage. Now we have motion makes current, and voltage makes torque. We’ve made the opposite of the magnetic motor. So now voltage makes force and current makes speed. We’ve turned the equation around. And so by doing this, the current that flows into this motor no longer flows through this resistance, and so therefore we don’t have, well I should say most of it for my being completely academic.
Most of this current goes over here to where it’s needed in this section, but this resistance here is no longer in series with that current. And so the conduction loss that crippled magnetic motors under certain circumstances has been eliminated. So this is just showing that when we spin it like it were a generator, right now the terminals in this machine, we’re measuring the current that flows through it. And so this machine when we spin it, it makes current, it doesn’t make voltage. It’s what’s called a current source. And because of that mechanism, now I’m not trying to shamelessly plug my startup or anything here, but of course when we made a lever arm, they put the name on it. In this case, again, a beautiful hand, this is what’s called a torque arm. And I’ve applied voltage to the motor. This meter here is measuring the current going into the motor, it’s in microamps. And so we can now hold torques with microamps of current instead of amps of current.
And why does that matter? Say you were a robot arm and you were wanting to hold something, now we have the ability to hold something and not ever heat up. So this is a FLIR camera that was taking a thermal image of the motor, and here you can see, well, we put some black tape here, and it’s 14 Celsius, we left it there sitting all day and it never heated up at all. And it can just hold indefinitely. And so for industrial automation, robotics, things like this where you have to position something and then hold it there, we’ve drastically, over 20X reduced the power. So the equivalent magnetic motor that we specked out required a 100 watts to hold a torque, and ours is less than five. Now going forward, we had to develop an entire new set of electronics to control a motor like this, ’cause you can’t go out and buy it. So we had to develop it ourselves, and we did. So it has to take low voltage from the wall, turn it into high voltage, and do it all efficiently and well. And this is something we just published this year. And then ultimately we installed it, the motor on a fan, and pay homage to Ben Franklin, we found a Ben Franklin action figure. [all laughing]
But anyway, and so this is just documenting. Here you can see the electronics generating the three phases that are required to spin this motor. And so this is the world’s first electric motor that could compete on a macroscopic scale with a magnetic one. So there’s lots of people that have worked on this over time, and this is my group down here. One of my students, Baoyun Ge. And these are the the torques that other people achieve. Torques per volt, and we’re orders of magnitude beyond, which is something we’re really proud about. And then here, finally, this has been our progress over the last few years. And so this is all the various prototypes and achievements and tweaks and changes. And so these are some standard industrial motors you can buy off-the-shelf, not car motors, but like industrial motors, general purpose ones, and so we finally surpassed some of the best ones you can buy off the shelf, and now we’re gunning for the electric vehicles.
So we’re hoping, and so you judge a motor by its, what’s called its torque density, its Newton meters per kilogram, how much torque can you get out of it for how much mass. And we’re hoping that since our motor’s so efficient from conduction not really being an issue, we want to hit this. This is in the range that we’ll wanna have, but we don’t require any cooling of any kind, so no radiators, no heat exchangers, no pumps, no nothing, which is typically associated with electric cars, motor drive platforms. So a leap towards the future. I’m hoping that we continue to innovate and things like this, and this is part of the Wisconsin Idea to do things like that. And we need to be bold. I mean, we got the transistor because someone told their boss, “Well, we think that we can, like attach wires to rocks– [audience member laughing] “–and have it replace a vacuum tube. ” I mean it was stated more elegantly than that, but the story is the same. And a closing thought, so Tesla, someone asked him about this and he said, “But the seemingly inseparable difficulties “which are now in the way, may be overcome “in the end, and then iron will be done away with, “and all electric machinery will be manufactured of aluminum “in all probability, “at prices ridiculously low. ” So with that, I thank you, and On Wisconsin.
[audience applauding]
Search University Place Episodes
Related Stories from PBS Wisconsin's Blog

Donate to sign up. Activate and sign in to Passport. It's that easy to help PBS Wisconsin serve your community through media that educates, inspires, and entertains.
Make your membership gift today
Only for new users: Activate Passport using your code or email address
Already a member?
Look up my account
Need some help? Go to FAQ or visit PBS Passport Help
Need help accessing PBS Wisconsin anywhere?
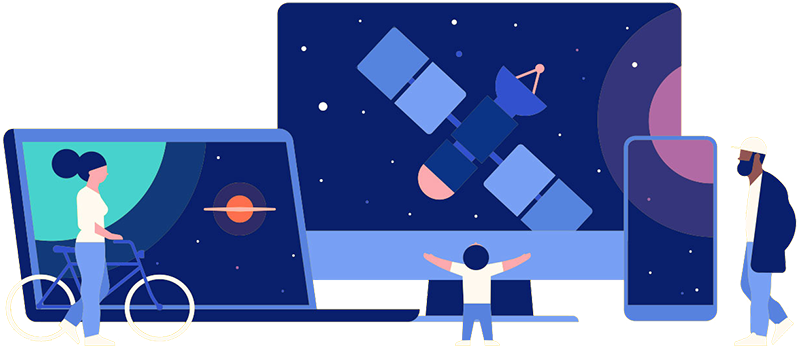
Online Access | Platform & Device Access | Cable or Satellite Access | Over-The-Air Access
Visit Access Guide
Need help accessing PBS Wisconsin anywhere?
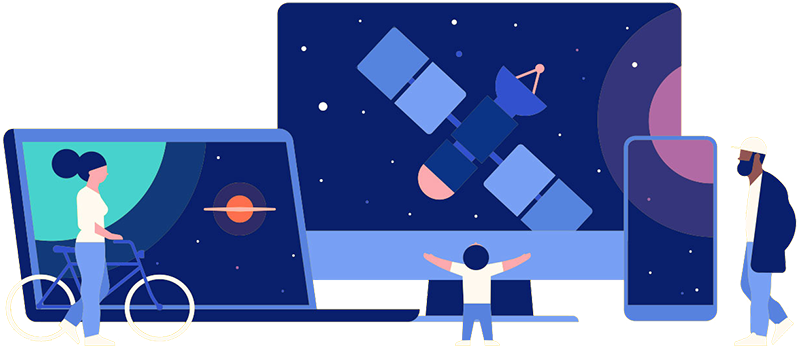
Visit Our
Live TV Access Guide
Online AccessPlatform & Device Access
Cable or Satellite Access
Over-The-Air Access
Visit Access Guide
Follow Us