[Title screen, The Wonders of Physics featuring said title over a spinning globe]
[whimsical music]
[slide of an old-time radio set up with the crackling of bad reception on an old radio and the title, 2018, 35th Season Celebration]
[Norman Gilliland, Host, Wisconsin Public Radio, acting as announcer for radio program]
I’m Norman Gilliland of Wisconsin Public Radio.
[Norman Gilliland, in the studios of Wisconsin Public Radio]
Last year in 2017, we celebrated the 100th anniversary of the first regularly scheduled broadcast which took place in the physics building on the U.W.-Madison Campus. This year, in honor of that, and to celebrate the 35th anniversary of The Wonders of Physics, Professor Sprott and his crew will be arranging a broadcast of their own, right here from the basement of the physics building. Let’s find out what exciting and colorful things they have in store for us.
[wide shot of auditorium]
[Peter Weix, Show Coordinator acting as Announcer]
And now, from the basement of the physics building at the University of Wisconsin, we present the 35th anniversary show of The Wonders of Physics. Professor Sprott is just arriving on his 1921 Indian motorcycle.
[sound of motorcycle engine roaring]
And now he’s opening the door –
[Foley artist dressed as Sherlock Holmes drops a large book creating a thud sound, on camera]
– and he’s walking the building.
[Akire Trestrial, a foley artist dressed as Sherlock Ohms uses wood blocks to imitate footsteps, on camera]
[whimsical music]
[applause]
[Sherlock foley artist holing up an Applause sign, on camera]
[Clint Sprott, Professor Emeritus, Department of Physics, University of Wisconsin-Madison]
Welcome to The Wonders of Physics. We’re pleased to bring you this live radio broadcast using a replica of the original 9XM radio transmitter.
[slide featuring a photo of the original 1917 9XM radio transmitter]
We’ll be presenting some of the best physics demonstrations from the past 35 years, as well as a few new ones –
[Clint Sprott]
– and our studio audience will be voting with their applause for their favorite physics demonstrations. And now, a word from our sponsor.
[slide featuring an illustration of the Peanuts character Snoopy the Dog dressed as the Red Baron]
[Peter Weix, off camera]
Hello, folks. Is your old doghouse too slow? Can’t keep up with that pesky Red Baron?
[Peter Weix, on camera]
Well, now the sky’s the limit in the –
[slide featuring an advertisement for The Sopwith Aviation Company with a Sopwith Camel airplane as part of their logo]
– 1917 Sopwith Camel.
[Sherlock foley artist imitating a planes engine buzzing, on camera]
[return to the slide with The Sopwith Aviation Companys logo]
[Peter Weix]
An agile, highly maneuverable biplane, the Sopwith Camel accounted for –
[slide with a color photo of a replica Sopwith Camel airplane]
– more aerial victories than any other Allied aircraft during World War I. And, unlike your doghouse, it can actually fly!
[Sherlock foley artist imitating a plane engine buzzing by, on camera]
[Peter Weix, on camera]
Test drive the Sopwith Camel today!
[Different disclaimer announcer, talking underneath an illustration of Snoopy in a red Sopwith Camel]
Sopwith Camel is noted for its tendency to crash. Side effects include vicious spin characteristics, engine choking, and cutting out during takeoff, crashing on takeoff due to the full fuel tank affecting the center of gravity, tail heaviness in level flight, immediate, uncontrolled spinning in the stall, and sudden right turns due to engine torque. Your results may vary.
[laughter]
[Clint Sprott]
Mr. Weix, air travel has come a long way since 1917 and our first program today is Let’s Fly with Captain R.O. Dynamic.
[applause]
[Mike Randall, dressed as a 1920s airplane pilot with goggles and white scarf, Captain R. O. Dynamic]
Cheerio, everyone. Welcome to Let’s Fly.
[Sherlock foley artist, on camera, imitates a plane engine roaring, firing a machine gun, and then exploding]
[Mike Randall as R.O. Dynamic]
Um, my name is Captain R.O. Dynamic. 100 years ago, the state of the art in motion was the airplane, or aero-plane, as it was called back then.
[shot of child in the audience]
[Mike Randall as R.O. Dynamic]
The most successful plane in World War I was the Sopwith Camel, introduced in 1917.
[slide titled, Rodney D. Williams, Wisconsins First Combat Ace, featuring a bulleted list of Mr. Williams vital statistics – Born on January 29th 1896 in Delafield Wisconsin, died on November 27th, 1972, in World War I assigned to the 17th American Aero Squadron, Royal Air Force, July and August 1918 piloted a Sopwith Camel fighter in combat over France, downed four enemy airplanes and one balloon to qualify as Wisconsins first combat Ace, was wounded on his last mission but landed safely and was hospitalized for the rest of the war]
In fact, Wisconsin’s first flying ace, Rodney D. Williams, flew a Sopwith Camel.
But what makes airplanes work?
[Mike Randall]
Well, we’ve all flown paper airplanes before.
[picks up a paper airplane]
They look simple enough.
[throws paper airplane which flies a short distance and then falls to the floor]
[audience oohs]
[shot of small child in the audience craning for a better look]
[Mike Randall as Capt. R. O. Dynamic]
But what holds them up? To answer that, we need to talk about the ocean that we all live under, our atmosphere.
I need a really strong volunteer from the audience, from the studio audience here. You look pretty strong. Why don’t you come up here?
Hi, what is your name?
[Young girl audience member, Cora]
Cora.
[Mike Randall as Capt. R. O. Dynamic]
Cora, oh yeah, you’ve got a good grip there. Come here. Okay, Cora –
[grabs two Magdeburg plates which are stuck together]
– pull those apart for me, please, will you?
[Cora attempts to pull the Magdeburg plates apart, wincing as she does so]
Any time. You can start. Don’t be shy.
[Cora stops pulling and looks at the Magdeburg plates which are still stuck together]
[Mike Randall as Capt. R. O. Dynamic]
What’s the matter? Oh, you know what? I forgot to tell you.
[Mike Randall takes Magdeburg plates from Cora]
There is something –
[Mike Randall as Capt. R. O. Dynamic pull the release on one of the Magdeburg plate handles and separates the plates]
There is a bit of a trick to this.
We need to understand something.
[Mike Randall places suction cups down]
The ocean of air that we live under is heavy. See the end of my thumb? It’s about an inch wide and an inch high. So, every place I can cover with the end of my thumb –
[Mike Randall pressing his thumbs on the top of Coras head]
– the air’s pushing down with about the weight of a bowling ball. So, you’ve got a lot of bowling balls sitting on your head. So why don’t you notice it? Here’s why.
[Mike Randall places thumbs on the opposite sides of Coras head and pushes]
You’ve got just as many pushing on this side as this side. So, the forces are in balance. But what I did here –
[Mike Randall picks up Magdeburg plates and places them together]
– these are called Magdeburg plates, by the way. When I flip this handle –
[flips handle on one of the Magdeburg plates]
– it pushes most of the air out from between the plates, so it’s not so many bowling balls on the inside pushing out, but the same number on the outside pushing in. You would’ve had to pull with over 200 pounds of force to get these apart.
You did a great job. Thank you so much for coming up here.
[Organ plays Ta-Da and Cora returns to her seat]
[applause]
[Mike Randall as Capt. R. O. Dynamic]
So, we’ve learned that a small imbalance in air pressure can generate a large force.
I need a couple other volunteers from the studio audience. I need a parent and child.
We’ll start with you. What is your name, sir?
[Young male audience member, Isaac]
Isaac.
[Mike Randall]
Isaac, come over here.
[Turns to adult audience member, Mike]
[Mike Randall]
And your name is?
[Adult audience member, Mike]
Mike.
[Mike Randall as Capt. R. O. Dynamic]
Mike, pleasure to meet you. Isaac, come over here with me.
[leads Isaac to a small step stool]
You’re going to sit on this like you’d sit on a horse and that’s the head.
[Isaac straddles the step ladder as if he is on a horse]
And, Mike, you’re going to stay right where you’re at. So, Isaac, right now, all the forces on you are in balance. We know this because you’re not moving. So, let’s see if I can unbalance the forces a bit by pushing –
[Mike Randall pushes on the bottom of the step stool which has no effect]
[grunts]
[Mike Randall pushes on the bottom of the step stool again which again has no effect]
– pushing on the bottom. But you know what? I’m applying a force to the side of this board, but it’s not going anywhere because there’s another force opposing me. It’s called the force of friction. There’s a lot of friction between the bottom of that board and the floor.
Now there’s a lot of things I could do to reduce friction. Me, I like getting rid of it altogether. Hey, Isaac – Isaac, you’re sitting on a hovercraft. There’s an air blower there. When I turn it on, it’s going to lift the board and you up a tiny little bit, but it’ll reach a new balance point, but that new balance point will be off the floor.
[Mike Randall as Cap. R. O. Dynamic turns on a leaf blower that is rigged to push air under a plate that the step stool is on causing plate to lift]
[Mike Randall spins the plate around causing Isaac to spin and then shoves Isaac to his dad, Mike, who catches him and spins Isaac back around and pushes him back to Mike Randall]
[Mike Randall]
Do one more spin.
[Mike Randall spins Isaac and the hoverboard around in place several times]
[Mike Randall turning off the leaf blower]
Alright.
Alright.
[Isaac jumps off the hoverboard and goes to his dad, Mike]
[applause]
So- so, by create – yeah, by creating the imbalance of forces, we were able to get rid of all that friction. You were able to move around. Great job both of you. Thank you so much.
[organ plays Ta-Da!]
[applause]
So, consider that what holds an airplane up is also due to an imbalance of forces. Oh, not from pumps or fans, but by the motion –
[slide featuring a cross-section of an airplanes wing with blue arrows indicating wind, a red arrow indicating the force of the wind against the wing and a green arrow indicating lift]
– of the airplane through the air. Let’s take a look at a cross-section of an airplane wing. Notice how it’s curved on top, flat on the bottom. So as the airplane moves through the air, the air going over the top of the wing –
[Mike Randall as Capt. R. O. Dynamic]
– has to travel farther, which means it has to travel faster to keep up.
The Bernoulli principle says that an increase –
[slide featuring an illustrated portrait of Daniel Bernoulli (1700-17820 above his equation and that also notes that he was a Dutch-Swiss mathematician remembered for his applications of mathematics to mechanics, especially fluid mechanics, and for his pioneering work in probability and statistics. Under the note are three illustrations of the Bernoullis principle]
– in air speed causes a decrease in air pressure. Let’s see what happens when we try that out. I need another volunteer from the studio audience. We’ll – well have a few more volunteers, don’t worry.
[a young girl runs down from the audience]
[Mike Randall as Capt. R. O. Dynamic]
What is your name?
[Young girl audience member, Rachael]
Rachael.
[Mike Randall]
Rachael.
Rachael, I want you to hold this up here.
[Mike Randall holds up a huge beach ball and sets it on a table]
You can just kinda lean it on there. Just hold it there. And when I tell you to, I want you to let go of it.
[Mike Randall, whistling and grabbing a leaf blower]
It’s a little bit loud.
[Mike Randall turns on leaf blower]
Okay, let go.
[Rachael lets go of huge beach ball]
[Mike Randall blows the huge beach ball high above the audience and then turns leaf blower off causing the huge beach ball to land in the front rows of the audience whereupon an audience member pushes the huge beach ball back to Mike who catches it and sets it in front of Rachael]
[organ plays Ta-Da!]
[laughter and applause]
So, what was happening there?
[slide of a cross-section of an airplane wing again this time showing the high air pressure under the wing and low air pressure above the wing and the difference in air pressure causing lift as a red arrow]
So, Rachael, I was blowing air over the top of that. By Bernoulli’s principle, it means that there was lower air pressure here, but there was higher air pressure under the ball and that higher pressure lifted the ball up and sailed it out into the audience.
[Mike Randall as Capt. R. O. Dynamic]
Isn’t that cool?
[Rachael nods her head]
Just like an airplane.
Thank you so much. You did a great job.
[Organ music plays Ta-Da!]
[applause]
[Mike Randall]
What do you think will happen if I blow air over the curved top of this roll of toilet paper?
[laughter]
Let’s find out.
[Mike Randall places a roll of toilet paper on a rig in front of the leaf blower and turns on the leaf blower causing the toilet paper to fly all around the room]
[audience oohing and ah-ing]
[Organ music plays Ta-Da!]
[applause]
[Clint Sprott]
Now let’s vote with your applause for your favorite motion demonstration.
Who liked the paper airplane?
[Applause meter appears on the screen to weak applause]
[Clint Sprott]
Nah.
Who liked the Magdeburg plates?
[audience applauds weakly and registers a boring on the Applause Meter]
[Clint Sprott]
Who liked the hovercraft?
[audience applauds and cheers and registers a pretty good on the Applause Meter]
[Clint Sprott]
Who liked the Bernoulli ball demonstration?
[audience applauds and cheers and registers a Fantastic on the Applause Meter]
[Clint Sprott]
And who liked the toilet paper gun?
[audience applauds and cheers loudly and registers an Amazing on the Applause Meter]
[Mike Randall as Capt. R. O. Dynamic]
Well, that’s all for me today. Next time on Let’s Fly –
[Inset video of the Sherlock foley artist spinning a wheel and touching the spokes with a stick causing a clicking sound]
– we’ll talk about Robert Goddard and his controversial idea about sending rockets to the moon.
[audience oohs]
[Organ music plays Ta-Da!]
[applause]
[Peter Weix as the Announcer]
And now, folks, a word from one of our commercial sponsors. Tired of high energy bills during this long, cold winter? Well, look to a new company for the answer, Solar Fusion Mining Company.
[slide titled, Solar Fusion Mining Company LLC and featuring a photo of the Sun and fake stock market information]
No, we aren’t talking about harnessing the sun’s infrared radiation here on Earth. We’re going to the sun and bringing part of it back. And with your investment, we can’t fail.
[Peter Weix]
Sure, there are a few technical problems, but we will be going at night when the sun is not shining.
[laughter]
So far there has been a massive reaction to our I.P.O. SUMI. Invest now.
[laughter]
[Off-camera Announcer]
Past performance of Solar Fusion Mining Company stock does not guarantee future returns.
[Clint Sprott]
And now, to get our energy going at the atomic scale and to warm us up, we have that famous English physicist, Dr. Calvin Scale, who at times is an absolute zero.
[applause]
[Terry Craney as Dr. Calvin Scale]
Did – did he just call me a zero?
[Young audience member]
Yeah.
[Terry Craney]
Well, I’m going to take that as a compliment, because I’m Calvin Scale, I’m super cool, and at times –
[slide with Zero degrees Kelvin written as 0 K]
I’m O-K.
[laughter and groans]
[Terry Craney as Dr. Calvin Scale]
So, let’s talk a little bit about heat, heat transfer, and the effects of heat and temperature on pressure and pressure on different materials. So, let me start here by warming up a little water. I’m going to turn on this Bunsen burner.
[lights Bunsen burner with butane torch. A circular mesh metal tray sits above the Bunsen burner]
And I’m going to pour some water into this paper cup –
[takes beaker of water and pours it into a paper cup]
– because a little later, I’d like some English tea. So, I’m going to put the paper cup on the burner and let it warm up –
[places paper cup full of water on the circular mesh metal tray above the Bunsen burner]
– like so.
Alright.
[laughter]
[Terry Craney]
We’re going to get those molecules a-vibrating back and forth –
[closes his hands into fists and waves them toward and away from each other]
– and get that water warmed up. By the way, don’t ever trust a molecule or an atom. They make up everything.
[audience groans]
[sound of crickets chirping]
[single snare drum hit]
Moving on.
[Pretending to sneeze] A-a-achoo!
Excuse me, I’m sorry. Oh, I don’t have a handkerchief. Anyone have a handkerchief anywhere? Oh, oh, – I’m –
[adult audience member in the front row hands him a handkerchief]
– thank you, thank you.
[Pretends to sneeze into the handkerchief] A-a-achoo!
Excuse me. Was this a clean handkerchief? Oh, it isn’t now. Okay, I’m sorry, before I give it back here, let me – let me rinse it out for you a little bit here.
[places handkerchief in a bowl of half water and half rubbing alcohol, wetting the entire handkerchief]
Here and see what we got.
[squeezes excess watery mixture out of the handkerchief over the bowl]
Okay, now it’s wet, sorry. So, okay. So let me start it –
[places wet handkerchief on the end of tongs]
– see if I can dry it off for you a little bit, so – okay even though it’s damp.
[takes butane lighter and holds flame from the lighter on the end of the handkerchief]
Oh, oh –
[handkerchief get engulfed in huge red flames]
– oh, oh my goodness! Oh no! Professor Sprott!
[siren wails and Professor Sprott grabs a fire extinguisher and sprays the lighted handkerchief extinguishing the flames]
– Oh no! Oh oh oh!
[siren still wailing]
I’m sorry, I ruined your – Wait a minute.
[unfolds handkerchief to show that it is still completely intact]
[Organ plays “Ta-Da!”]
[applause]
[Terry Craney as Calvin Scale]
It’s not even singed. What’s going on here? What – what happened?
Well, what happened is that this wasn’t pure water. That’s 50% water and 50% rubbing alcohol, very similar to what you might have in your medicine cabinet at home.
And what lit on fire first was the alcohol because it has a lower kindling point or ignition point compared to the cotton in the cloth. But more importantly, there was water in here and water’s a great heat absorber. It has a very high specific heat as physicists call, and if something has a high specific heat, it can absorb a lot of heat into itself without rising much in temperature.
And furthermore, if some of the water did get to the boiling point, and started turning into steam, that takes a lot of energy, too, called heat evaporization. So, the water – raising the water – the temperature – the temperature of the water and turning it into steam absorbed the heat rather than into the handkerchief and the handkerchief was safe. So, thank you.
Your name is?
[Audience member, Cora]
Cora.
[Terry Craney]
Cora? Thank you, Cora.
[Organ plays “Ta-Da!”]
Let’s give Cora a hand.
[applause]
So let me get back to my cup here that I was boiling water with, okay.
[grabs the cup of water off of the Bunsen burner with a pair of tongs]
And I’m going to dump the water out of here.
[pours steaming water out of the cup]
As you can see, it’s turned into steam, but if you look at the bottom of the cup, it’s basically very – just a little singed on it.
[Organ plays “Ta-Da!”]
[applause]
So, I have a canister here that has some soaked paper towels with lighter fluid – charcoal lighter fluid, and I’m going to start it on fire.
[uses butane torch and lights the paper towels in the canister on fire]
And if you notice that flame goes up several inches now, okay. And how does a flame work? Well, the flame – the hot flame then, they expand – those molecules in the flame expand.
[places his hands into fists and then shakes his fists together]
They take up a bigger volume –
[opens his hands and creates a circle motion with his hands]
– and we say it’s less dense. Less dense materials rise up –
[moves his hands from the bottom of the circle to indicate heat rising in cold air]
– in colder air.
So, the flame goes up.
[moves his hands up]
Cold air from the sides –
[moves his hands in a circular motion to the bottom of a circle]
– come in and take its place, and that air has oxygen, and it feeds the flame.
[moves both hands in two circles]
So, we get a pattern like this. It’s called a convection current and convection currents are all over. A lot of your weather patterns are due to convection currents. So, now what I’m going to do is I’m going to take this cylinder, this screen cylinder, and put it over the top of the flame.
[places large screen cylinder over the top of the flaming canister]
And the cylinder is on a rotating disc, and I’m going to rotate the disc –
[starts rotating the disk that the cylinder is on]
– and watch what happens.
[whimsical piano music]
[the flame in the cylinder grows in height to about 2 feet high – the top of the large cylinder -and starts twisting]
Okay, this is called a fire tornado and fire tornadoes actually do exist in real life.
[slide featuring three photos of real life fire tornadoes]
We have out West in the wildfires, if you have strong, swirling winds, you can get fire tornadoes that go up several hundred feet.
[Organ plays “Ta-Da!”]
[applause]
[snuffs out flaming cylinder]
Now let’s talk about the effects of pressure. Remember Captain Dynamic earlier talked about pressure and this sea of air we have above us and the effects of that pressure on your boiling point and freezing point of water. Does anyone know the boiling point of water? Anybody?
[Young audience member]
212 degrees.
[Terry Craney]
212 degrees, or 100 degrees Celsius. By the way, in my scale, it’s 373 degrees, in the Kelvin scale.
But that’s only if you’re at one atmosphere of pressure. So, that’s the boiling point in New York City or in San Francisco. Here in Madison, Wisconsin, we’re about 700 feet above sea level. The boiling point is a little bit less –
[slide titled Boiling Points of Water at Various Altitudes, showing the boiling point of water at Sea Level – 212 degrees Fahrenheit or 100 degrees Celsius, Madison Wisconsin (740 feet above sea level) – 210 degrees Fahrenheit or 99 degrees Celsius, Denver Colorado (5400 above sea level) – 203 degrees Fahrenheit or 95 degrees Celsius, and Mount Everest, Nepal (29029 above sea level) – 160 degrees Fahrenheit and 71 degrees Celsius]
– as you can see. And it’s even less in Denver, Colorado, and the top of Mt. Everest, it’s even lower. So, what I have over here is a flask that has just distilled water in it.
[Terry Craney]
No trickery in this, just plain distilled water. It is exposed to atmospheric pressure, right now. So, the boiling point’s around 100 degrees Celsius, or 212 Fahrenheit. I’m going to lower the pressure over the top of that flask. I’m not going to do it by raising up in altitude. I’m going to flick a switch on a vacuum pump which is going to suck the air out and watch what happens.
[turns on vacuum pump attached to a container of distilled water]
It’ll take just a few seconds –
[the water in the container starts to boil rapidly]
– but it starts vigorously boiling. I lowered the pressure to the point where room temperature was warm enough to boil it away. Now, if I let this go, this is turning very cold.
[places his hand on the bottom of the container]
I can feel it being very cold and if I let that go a few more seconds, you will start seeing an icy skim on the top. Why is that?
Well, remember I said to boil something, to go from liquids to gas, you need heat evaporization. There’s no outside heat here. So, it took the heat internally from the water and from the flask to do that. So, wow, you can boil and freeze water at 32 degrees, at the same temperature if you lower the pressure.
[Organ plays “Ta-Da!’]
[applause]
So, I’m going to take a match here, put it on the end of this stick, and come over here.
Light the match, and we’ll see what we get.
[places lit match at the end of a long stick and then places the flame of the match next to a balloon which pops]
[audience gasps]
[Terry Craney]
What do you think?
[Audience members]
Helium.
[Terry Craney]
Helium, yeah, it blew up, but it would’ve been no different if I’d taken a pin and kind of just popped it up there, okay? So, it was pretty obvious it was – it was helium.
Now one of the things in physics is you should never take anything for granted, and another principle in physics is that you should always repeat your experiment to see if you get the same results or different results. So, we’re going to blow this one up then.
We’ll put my match on here.
[relights match on the end of the long stick]
[Peter Weix as the announcer]
I’m out of here. You’re on your own.
[Peter exits stage right]
[Terry Craney as Calvin Scale]
He is such a chicken.
[laughter]
[Terry Craney]
All right, you may want to cover your ears on this one.
[places lighted match next to the second balloon that explodes in a bright red flame]
[Organ plays “Ta-Da!”]
[cheers and applause]
[Terry Craney]
Pretty obvious we had hydrogen in there. Hydrogen, very explosive gas, lot of energy. Very light gas, that’s why it’s used in spaceships and rockets to get outside of our atmosphere.
[Clint Sprott]
And now, let’s vote with your applause for your favorite heat demonstration.
[An applause meter is superimposed on the screen]
Who liked the boiling in the paper cup?
[audience applauds with applause meter registering at Not Bad]
Who liked the non-burning handkerchief?
[audience applauds with applause meter registering at Not Bad]
Who liked the fire tornado?
[audience cheers and applauds with the applause meter registering at Amazing]
Who liked the freezing by evaporation?
[audience applauds with applause meter registering at Fantastic]
And who liked the exploding balloons?
[audience applauds and cheers with the applause meter registering at Amazing]
[Terry Craney as Calvin Scale]
Thank you.
[Peter Weix as the Announcer]
And now, folks, another word from a commercial sponsor.
Do you want to take your music wherever you go?
Well, now with the Pauli Portable Player, you can.
[slide of the Pauli Portable Player which is an old Gramophone being held over the head of a man named Pauli who strains under the weight of the Gramophone]
Weighing in at only 30 pounds, our record player lets you be the life of any party. Pauli, a proud sponsor of Sound Off with Rileigh Rayleigh.
[Clint Sprott]
And now to break up the talk radio with some sound waves of her own, it’s Sound Off with Rileigh Rayleigh.
[applause]
[Emily Ehlerding as Rileigh Rayleigh]
Well, hello, everyone. I’m Rileigh Rayleigh and this is Sound Off. Today we’re going to be bringing you some of the top songs of the last 50 years and some science along with them.
Now since we’re on the radio, it makes sense to talk about sound, right? Well, sound travels through the air, or other things, as a wave. Basically, the air molecules are squished together –
[Sherlock foley artist plays a slide whistle from low to high]
– and pulled apart –
[Sherlock foley artist plays a slide whistle from high to low]
[slide showing a speaker with sound vibrations traveling to an ear on the top and the sound vibrations shown as a wave below]
– like you in our studio audience can see in this diagram. When those squished –
[foley artist playing slide whistle from low to high]
– and pulled apart
[foley artist playing slide whistle from high to low]
– bits of air hit our eardrums. Our nerves in our brains take that mechanical pressure, you know, the squishing and pulling –
[Emily Ehlerding]
– and make it so that we hear a sound, or a song, like this one from the 1950s by The King.
[Elvis Presley’s “Hound Dog” recording playing]
You ain’t nothing but a hound dog/ Crying all the time
[Physics T.A. dressed in 1950s clothing comes out from behind the stage and starts dancing and then stops, looks at the audience and runs backstage]
[Emily Ehlerding, laughing]
Now, the speed at which sound travels is going to depend on the thing that it’s traveling through.
Sounds will travel faster through lighter gases, like we just learned, like helium. So –
[Emily holding a helium filled balloon exhales deeply and then inhales helium from the balloon]
[Emily speaking in a high-pitched helium induced voice]
A molecule of helium weighs about seven times less than your average air molecule, meaning the sound of my voice travels about three times faster.
[laughter]
And in this case, faster means higher pitched.
[Organ plays “Ta-Da!”]
[applause]
Kind of makes me sound like all the fan girls who saw our next group – The Beatles.
[Beatles recording of the Isley Brothers Twist And Shout plays while a Physics T.A. dances to it]
So, shake it up, baby, now/Shake it up, baby
Twist and shout/Twist and shout/
Cmon, Cmon [music fades out]
[applause]
[Emily Ehlerding]
Now sounds travel fast through lighter things, right? So, it makes sense that they’re going to travel slower through heavier things. There are also gases that are heavier than air, like sulfur hexafluoride.
[Emily holding a balloon filled with sulfur hexafluoride exhales deeply and then inhales the sulfur hexafluoride from the balloon]
[Emily Ehlerding speaking in a very low-pitched voice]
A molecule of sulfur hexafluoride weighs about five times more than your average air molecule. So, the sound of my voice travels about three times slower and sounds much deeper.
[Organ plays Ta-Da!]
[applause]
It’s kind of the opposite of our next group, The Bee Gees.
[recording of the Bee Gees Night Fever plays while a different Physic T.A. dressed in a red construction hat, silver gloves, and a suitcoat dances]
Livin’ on the music so fine /Borne on the wind
Makin’ it mine/
It might be the Night Fever [music fades out]
[Emily laughing]
[applause]
[Emily Ehlerding]
Now there are some ways to see what a sound wave looks like, you know, to see those squished –
[sound of slide whistle from low to high]
– and pulled apart –
[sound of slide whistle from high to low]
– bits of air. The most science-y way is using an oscilloscope. However, it’s just not really exciting. You guys know what is exciting?
[Young audience member]
Fire.
[Emily Ehlerding]
Lighting things on fire, yeah.
[laughter]
So, since sound travels by squishing –
[inset shot of Sherlock foley artist playing a slide whistle from low to high]
– and pulling apart –
[inset shot of Sherlock foley artist playing a slide whistle from high to low]
– bits of air, the pressure and density will change at different points on this tube if I send a sound wave into it.
[Emily Ehlerding standing behind a very long copper tube that has flames over the first half of the tube]
And when the pressure and density changes, you’ll see that the fire changes, too. So, let’s turn on our sound.
[Emily plays a high-pitched sound and the flames along the pipe break up into smaller areas of flame about two inches apart]
Can you guys hear that?
So, you can see the different parts of the wave.
So, let’s see if we find another frequency here.
[Emily turns the sound up to a higher-pitched frequency and the flame groupings become shorter and the flames grow higher]
We can get different looking waves because of the different sounds. So, you can physically see what the sound waves look like.
[Organ plays Ta-Da!]
[applause]
And speaking of physical, this is Olivia Newton John.
[Recording of Olivia Newton Johns song Physical plays while a Terry Craney dressed in a headband comes out from behind the back curtains and dances]
Let’s get physical, physical/
I want to get physical/
Let’s get into physical/ [music fades out]
[Emily laughs]
[applause]
[Emily Ehlerding]
Every time that somebody plays an instrument, you know, a guitar, a violin, flute, trumpet, anything, they’re going to be creating something called a standing wave. The idea behind this is that sounds like to travel –
[slide titled Harmonics of a Guitar showing an illustration of an electric guitar with the 12th, 7th and 5th frets shown and underneath the guitar are the sound waves produced the 2nd, 3rd, and 4th harmonics showing how the sound waves get shorter with each increase in harmonic]
– in the way that’s easiest for them. They’re going to create a pattern that kind of looks like that, that it can just keep going and going.
[Emily Ehlerding]
Now I have something here that will create a standing wave. It’s not really an instrument, but of course it involves fire. So, let’s light this flame.
[Emily Ehlerding, lighting a Bunsen burner in front of a long section of PVC pipe]
Now since sound travels once again by squishing –
[sound of slide whistle from low to high pitch]
– and pulling apart –
[sound of slide whistle from high to low pitch]
– bits of air, the density changes, right? And another thing that we learned that can change the density of air is heating it up, like we can do with this flame. And what happens to less dense air? It rises, right?
So, if I were to put this tube over the flame, it’s kind of like blowing air through a really big straw. It’ll create a standing wave that sounds something like this.
[Emily places a short PVC pipe over the Bunsen burner which creates a mid-range humming sound]
[Ooohs from the audience]
[Emily Ehlerding]
Yeah, so I also have a longer tube here. Do you guys think that this long tube will sound higher or lower pitched than the short one?
[audience]
Higher.
[Emily Ehlerding]
Higher? Lower? Why don’t we find out, okay? Ready, three, two, one.
[Emily places longer PVC pipe over the Bunsen burner and the result is a lower pitched hum]
[Organ plays Ta-Da!]
[Emily Ehlerding]
So, it was lower.
[applause]
So, my longer tube had a longer standing wavelength, which meant it sounded deeper. Shorter tube, shorter wavelength, higher pitched sound. Those sounds are kind of obnoxious, though, weren’t they? Yeah, speaking of obnoxious, here’s the Macarena.
[Recording of “Macarena” by Los Del Rio plays while a Physics faculty comes out from behind the curtains and does the Macarena dance]
So, since sound travels as a wave, it has some power to it, like water waves or things like that. Also turns out that strings and tubes aren’t the only things that can have standing waves and resonant frequencies. Everyday objects like, say, this glass can also have resonant frequencies. However, some things really don’t like having standing waves in them.
[Emily stands in front of a cabinet that has three sections behind a sheet of plexiglass. In the first section is a frequency emitter, in the second section is a glass beaker]
For example, I have a sound wave here that we have perfectly tuned to the resonant frequency of our glass, and you can see as we turn up the power –
[Emily turns up the power to the frequency emitter]
– our glass will start to shake because of all that power from the sound wave.
[A piece of tubing in the glass beaker in the middle section of the crate starts to vibrate back and forth from the sound waves]
What do you guys think would happen if I turn up the sound even more?
[Young audience member]
It’ll break.
[Emily Ehlerding]
It’ll break. Help me count down. Ready? Three, two, one.
[Emily turns up the power to the frequency emitter and the glass breaks]
[Organ plays Ta-Da!]
[slow motion replay of the glass beaker breaking]
[applause]
[Clint Sprott]
And now let’s vote with your applause for your favorite sound demonstration. Who liked breathing helium and sulfur hexafluoride?
[An Applause meter is superimposed on the screen]
[audience applauds and cheers sending the applause meter to Fantastic]
Who liked the flame pipe?
[audience applauds and cheers sending the applause meter to Amazing]
Who liked the hoot tubes?
[audience applauds and cheers sending the applause meter to Fantastic]
And who liked breaking the beaker with sound?
[audience applauds and cheers sending the applause meter to Amazing]
[Clint Sprott]
And now to get some sparks out of your radio, we have our own electrifying duo of electrifying hijinks, Sparky and his sidekick Mr. E.
[Michael Winokur appears as Mr. E. dressed in a silver bodysuit with his face also painted silver, holding a stuffed bunny and stuffed eel]
[electrical buzzing sounds as he approaches the microphone]
Hello there, partner. Yep, it’s time for another hair-raising adventure of Sparky the Wonder Eel, he’s electric, and me, I’m a real mystery. That is short for Mr. E-lectricity, your low resistance conductor of today’s episode. But first a word from our sponsor, Faraday Cages, shielding you from all of life’s unwanted e-fields.
[Peter Weix, appearing as the Announcer]
Yes, listeners, it’s true. Electric fields are nearly everywhere, cell phones, microwaves, power outlets. It can affect your potential and not always in a positive way. Well, the kind folks at Faraday’s have the perfect refuge. Just reserve a vacation in one of Faraday’s equipotential cages and you can be field free for as long as it takes to get your charge balance back.
[slide titled Faradays Equipotential Cages, featuring two photos, one of an old time Faraday cage and one of a modern Faraday cage]
Soothing, peaceful, relaxing, and highly recommended by all of Sparky’s closest neighbors. Now back to the show.
[Michael Winokur as Mr. E.]
Sparky wants – hopes everyone knows that there are two kinds of electrical charges, positive and negative. And objects with opposite electrical charges attract one another. But things with like or the same electrical charge repel. There is a simple but classic demonstration to show this. All it takes is two identical black plastic rods –
[holds up two plastic rods]
– some of Sparky’s fur. I guess he’s a male. And some vigorous rubbing. But first, I have to convince you that these rods right now are electrically neutral. So, there are no long-range electrical forces and so I’ll put one of these rods on the hanger like this –
[places one of the rods on a hanger which is attached to an arm on a tripod]
– and I’ll bring the other one –
[moves the other plastic rod towards the hanging plastic rod and nothing happens]
– and you’ll see that there’s no real force until they actually touch. But now I’ll take it back here.
[sound of sneezing]
Oh, gesundheit, Sparky.
[Mr. E. rubs one of the plastic rods causing static electricity]
Now we’ve transferred electrical charges and since these rods are identical, they should have the same charge –
[Michael Winokur places one of the plastic rods back on the hanger]
– and so there should be a repulsive force. Now let’s just see.
[Michael Winokur as Mr. E. tries to touch the two rods together, but the electrical force causes them to repel and the rod on the hanger to spin around as he tries to touch it with the other rod]
I can’t even get close, see?
[audience oohs]
[Organ plays Ta-Da!]
[applause]
[sound of electrical buzzing]
[Michael Winokur as Mr. E.]
Oh, I didn’t forget, I didn’t forget. Sparky also wants – hopes everyone knows that everyone knows that electricity or electrical currents or charges can flow through conductive objects similar to water flowing through a pipe, except the electrical charges, usually electrons, they flow right through the metal itself rather than in the pipe.
In fact, if we give those electrons enough electric potential, they’ll even flow through the air. This device, a Jacob’s Ladder, does just that.
[Mr. E. walks toward a Jacobs Ladder]
Watch what happens when I turn on the switch.
[Michael Winokur turns on the switch at the Jacobs Ladder, causing an electric current to jump between the two rods of the ladder that moves from bottom to top and back again]
Do you see that?
[audience oohs]
[Michael Winokur as Mr. E.]
So, what’s happening? Well, if you look underneath, there is a blue box and it’s called a step-up transformer and that raises the electric potential to thousands of volts and where the two metal electrodes come close together, the electrons go through the air and complete a circuit. But you don’t really see the electrons. What happens is those energetic electrons excite the atoms inside the air molecules and excited atoms emit characteristic colors of light that you can see. Poor Sparky, he’s just green with envy.
[sound of crickets chirping]
[audience groans]
[Michael Winokur]
Well, that marching motion of electrons in charge per second is called a current.
And speaking of currents, did you know Sparky’s favorite subject in school?
Current events.
[Inset of Peter Weix as he plays a rimshot on a drum and cymbal]
[Michael Winokur as Mr. E.]
You know, he really is well grounded.
[sound of crickets chirping]
I just couldn’t resist.
[Inset of Peter Weix as he plays a rimshot on a drum and cymbal]
[Michael Winokur as Mr. E.]
So, electrical currents can also cause objects to move. A device which converts electrical energy into mechanical energy is simply called an electric motor. In 1822, a scientist, Michael Faraday –
[slide titled Faradays Law featuring a photo of Michael Faraday and an illustration of his Law with a magnet with a changing magnetic field inducing a current and that current is pushed by the electromotive force (EMF) and noting that Faradays law is contained in Maxwells Equations]
– invented the first electric motor, but what Sparky and I really like about this motor is you can make this electric motor at home. All it takes is a AA battery –
[Michael Winokur as Mr. E. pointing out a AA battery taped to the side of a piece of cardboard]
– which we’ve taped up so you can see, some super strong conductive magnets –
[slide titled A D.Y.I. Electric Train showing all the pieces necessary to do the experiment at home including a AA battery, small round magnets, and a metal coil. Additionally, a photo of the metal coil wrapped around the AA battery with the magnets on both ends of the battery and an illustration of the physics involved noting that the upper magnet is pushed while the lower magnet is pulled causing the bar magnet in the middle to spin]
– on either side, you see them here, one, two, and then just a long coil of bare wire. And all we have to do is insert the assembly into the coil completing the circuit and –
[inset of Sherlock foley artist honking a horn]
[the D.I.Y train battery contraption flows through a circular tube]
[audience oohs]
[Organ plays Ta-Da!]
[Michael Winokur as Mr. E.]
Now for this demonstration, I will need an energetic volunteer from the audience.
[Clint Sprott]
Mr. E.! Mr. E.! I just noticed that we have with us today a very special guest, and I think we should use him in this demonstration.
[Michael Winokur as Mr. E.]
That sounds like a plan.
[Clint Sprott]
Ladies and gentlemen, please give a welcome to Norman Gilliland from Wisconsin Public Radio.
[audience applauds and cheers]
[Clint Sprott]
Now, Norman, I know you used to come to these shows years ago, is that correct?
[Norman Gilliland]
Yeah, when I could get in.
[Clint Sprott, laughing]
It’s been popular for many years.
[Norman Gilliland]
Yes.
[Clint Sprott]
And how long have you worked for Wisconsin Public Radio?
[Norman Gilliland]
34 years.
[applause]
[Clint Sprott]
Thirty – just exactly as long as we’ve been doing these shows.
Now 34 years, that’s not very long in the life of Wisconsin Public Radio.
[Norman Gilliland]
That’s only about a third of it.
[Clint Sprott]
Right. Did you know that this very apparatus that we’re about to show you was one of the first radio transmitters used by WHA?
[Norman Gilliland]
I’ve heard rumors.
[Clint Sprott]
Well, here it is, and we’re going to give you a close-up look at it.
[Norman Gilliland, laughing]
Okay.
[Michael Winokur as Mr. E.]
Okay, so this is Norman and Sparky’s new electrical adventure.
[Mr. E. hands the stuffed eel to Norman]
Is your insurance all paid for?
[Norman Gilliland]
Uh, no, but I noticed that Clint was awfully good with that fire extinguisher. And I would imagine a lot of practice or what are we up against?
[Michael Winokur as Mr. E.]
We’ll find out. Well, come with me, and we’ll take you over to our Faraday cage.
[organ plays the Imperial March from Star Wars as Mr. E. leads Norman to a Faraday cage]
[laughter]
[Michael Winokur as Mr. E.]
Everyone take notice. It looks a little like a, or a lot like, an electric chair.
[ominous organ music]
[Michael Winokur, opening the door of the Faraday Cage]
[Norman enters the Faraday Cage and sits on a wooden chair within the cage]
Make yourself at home. All the conveniences. Are you comfortable?
[Norman Gilliland]
Oh, yes.
[Michael Winokur as Mr. E., closing the door of the Faraday Cage]
All right, we’ll just latch you in here. Now the cage is metal so all of the electrical sparks and electricity will go through the cage rather than through Norman, which is a good thing. I’ll just run the control panel.
You may not want to touch the wires. Even if you do, it’s probably okay.
You ready?
[Norman Gilliland]
Ready.
[Michael Winokur as Mr. E.]
All right –
[House lights go dark]
– I’ll engage the spark app, the solenoid, and turn up the potential.
[Michael flips and electric switch which causes a Tesla coil to spark and send a lightning-like electrical current jumping from the Tesla coil to the Faraday cage]
[House lights come on revealing an unharmed Norman]
[Organ plays Ta-Da!]
[applause]
[Michael Winokur as Mr. E, to Norman]
How are you feeling?
[Norman Gilliland]
Still here.
[Michael Winokur, opening the Faraday cage]
That’s good, glad to hear it. [retrieving the stuffed eel from Norman] And Sparky looks pretty good, too. Let’s give Norman a great hand.
[applause, as Norman makes his exit]
[Organ plays Ta-Da!]
[Michael Winokur as Mr. E.]
Well, so far, we’ve talked mainly about electrical circuits. In fact, electric fields can move through space if they’re joined by – with a magnetic field. In fact, one of the devices that does that, that has electric fields that’s in your house is called a microwave.
Did you see Sparky’s tail move?
You probably didn’t. That’s what Sparky calls a microwave.
[sound of a rimshot]
[Michael Winokur]
In this case, it’s hard to see those electric fields, but we can do an experiment to show that they are there.
We’re going to do something you’re not supposed to do at home and never, ever do this.
[slide titled Microwave Electric Field Strength showing an infrared image of a microwave oven at work and noting that the stronger the microwave electric field the hotter the food. Additionally, there are two graphs on the slide showing that as the electric field distribution increases the magnetic field distribution decreases in an inverse relationship]
[Michael Winokur]
It’s put fine metal wire inside the microwave because electric fields condense or become very strong at – at fine metal wires. So, inside this ordinary, old-fashioned light bulb is a filament that goes round and round and rather than sticking in a light socket, in the name of science, we’re going to do this experiment –
[Michael Winokur places a lightbulb in a glass beaker into a small microwave oven and closes the door]
– and see what happens when the electric field collects at the filament.
Are you ready? Let’s go.
[the lightbulb in the beaker lights up in the microwave oven]
Look carefully. You’ll see.
[audience oohs]
[Michael Winokur as Mr. E.]
So, it’s similar to the Jacob’s Ladder in that it produces all sorts of characteristic colors of light due to the excited atoms near the filament.
[Organ plays Ta-Da! while Michael removes the beaker from the microwave]
[applause]
[sound of electrical buzzing]
[Michael Winokur]
Oh, oh, wow, wow, Sparky is getting all charged up in our electrical adventure. Another thing that can get charged up is inside this mysterious box over here that’s orange. It’s called a capacitor, and a capacitor is a very simple device.
[Michael points out two pieces of aluminum foil that are attached between the positive and negative poles of a large battery]
It’s just alternating metal plates, but when I push this button here on this little box [pushing the button on the box] we’re going to use this high voltage battery and charge those plates positive, negative, and positive and negative.
Now those charges want to attract one another, but they’re not allowed to sort of collect together or form a current until I release the button. We will take all of that charge we’re storing and pass it right through that aluminum foil in a fraction of a second when it hits about 5,000 volts or 50 on the galvanometer.
Is it there? It’s a little hard for me to see. Tell me, are we up over 50?
[Clint Sprott, off camera]
Yup.
[Michael Winokur, releasing the button]
All right, this could be a bit loud. Let’s go.
[the piece of aluminum foil explodes with a small bang]
[Organ plays Ta-Da!]
[audience oohs]
[Michael Winokur]
Well, we finally managed to get rid of that stationary electrical charge we’ve been storing. Everybody should be positively ecstatic.
[audience laughs and groans]
[Clint Sprott]
And now let’s vote for your favorite electricity demonstration.
[An applause meter is superimposed on the screen]
Who liked the electric forces on the plastic rods?
[audience applauds sending the applause meter to Boring]
Who liked the Jacob’s Ladder?
[audience applauds sending the applause meter to Not Bad]
Who liked the Faraday train?
[audience applauds and cheers sending the applause meter to Pretty Good]
[Sherlock foley artist toots an old bicycle horn]
[Clint Sprott]
And who like the Tesla coil and Faraday cage?
[audience applauds and cheers sending the applause meter to Amazing]
Who liked the light – light bulb in the microwave oven?
[audience applauds and cheers sending the applause meter to Amazing]
And who liked the exploding foil?
[audience applauds and cheers sending the applause meter to Amazing]
[Michael Winokur as Mr. E.]
Well, until next time, radio friends. Hi ho, Silver, away!
[Organ plays Ta-Da! as Michael makes his exit]
[applause]
[Clint Sprott]
Our next radio program will be a reading of Sherlock Ohms –
[slide with two cartoon characters, Sherlock Ohms – an Ohm symbol with a smiley face, a deerstalker hat and holding a magnifying glass, and Dr. Watts-On, a light bulb with a hat, mustache, and a briefcase and the title – A Brilliant Induction!]
– by his assistant Dr. Watts-On.
[laughter]
[Ryan Norval as Mr. Watts-On]
Good afternoon, everybody. Welcome back to another exciting reading of Sherlock Ohms’ Magnetic Mysteries with me, Dr. Watts-On. Will Sherlock Ohms solve the mystery?
[sound of a slide whistle]
Or has he met his polar opposite? Find out today on Sherlock Ohms, A Brilliant Induction. But first, a word from our sponsors.
[Peter Weix, as the Announcer]
Hey, amateur detectives, ever go looking for clues only to have your batteries run out? No way to turn that flashlight on, power up the camera, or call for help? Well, try Farad’s new ultra-high-capacity capacitor, guaranteed to store more charge than those pesky batteries.
[applause]
[Ryan Norval as Mr. Watts-On]
It was a dreary London morning. Sherlock Ohms and I were having our morning tea. I was reading the newspaper and noticed a crime had been committed in the London Underground. A thief, after stealing wallets and purses, jumped in front of a subway train, vanishing mysteriously.
[Akire Trestrial as Sherlock Ohms]
Why, Watts-On, people do not just vanish mysteriously. The game is afoot!
[Ryan Norval]
Sherlock and I wandered down to the subway station and began looking for clues. I went over to the subway tracks and took a look and said, Sherlock, there is no way a person could survive if they jumped in front of a train.
[Akire Trestrial]
Ah, quite to the contrary, Watts-On. One only needs to be propelled upwards very quickly to get out of the way of a moving train. Ah, look here, Watts-On, an electromagnet on the railway’s electrified center rail. The thief must’ve used this to vault over the train.
[Ryan Norval as Dr. Watts-On standing in front of a large electromagnet]
Now, listeners, at the time, I didn’t know what an electromagnet was, and I’m sure some of you are interested to learn yourselves. So, I will explain as Sherlock explained to me that day. An electromagnet is simply a hunk of iron surrounded by copper coil. When that copper coil has a current pass through it, if we do this very quickly, it’ll produce a magnetic field. This magnetic field can then be used to induce a current in any metal object. I have here a ring of aluminum such as the thief may have had on his shoes, and that current in the ring will feel a magnetic force.
[Ryan places a metal ring on the top of the electromagnet]
Let us find out. Three, two, one.
[Ryan turns on the electromagnet and the metal ring is propelled about 6 inches from the top of the electromagnet and then falls back down]
[audience oohs]
[Ryan Norval as Dr. Watts-On]
Sherlock, that ring didn’t jump nearly high enough to get over a train.
[inset of Akire Trestrial as Sherlock Ohms]
A great observation, Watts-On. But there are things we can do to make the ring jump higher. Say, the thief had a bottle of liquid nitrogen on him.
[Ryan Norval holds up a container filled with liquid nitrogen]
If we cool that ring down with that really cold liquid nitrogen –
[Ryan Norval places the metal ring into a small dish and pours some liquid nitrogen on it]
– we can change the electrical properties of the metal. It becomes less resistive, allowing a large, induced current to flow on the ring, which in turn allows for a larger magnetic force to exist on the ring. Show the nice audience, would you, Watts-On?
[Ryan Norval as Dr. Watts-On grabs the metal disk out of the liquid nitrogen with metal tongs]
So, liquid nitrogen is at a very warm temperature of negative 321 degrees Fahrenheit. Not something that you’d want to be handling just out of – out of the blue.
[Ryan Norval places the super-cooled metal ring on top of the electromagnet with the tongs]
So, now with liquid nitrogen, let’s see how much higher we get.
Three, two, one.
[Ryan Norval turns on the electromagnet and the supercooled metal ring jumps about twice as high as it jumped when it wasnt supercooled, and Ryan then catches it with the tongs]
[audience oohs]
[Ryan Norval as Dr. Watts-On]
So, we, like, doubled the height of the ring jump there. But that’s still not quite enough to get over a train. Let’s say, on the other hand, the thief, in addition to that metal ring in his boot, happen to have a stilt of iron or something.
[Ryan Norval places a large hollowed out iron tube on top of the electromagnet]
[laughter]
[Ryan Norval]
Now you’re probably wondering why a thief would have a stilt of iron on his boot. Well, with an electromagnet, the magnetic field tends to taper off right where the iron ends. So, by adding an additional piece of iron, we’ll be able to extend the magnetic field up and put the ring in that magnetic field longer. Longer time in the magnetic field means hopefully we’ll get it to accelerate faster.
[Ryan Norval places the supercooled iron ring around the outside of the iron tube but on top of the electromagnet]
So now the question is, who thinks this setup will get someone to jump over a train? Let’s find out, then.
Three, two, one.
[Ryan turns on the electromagnet and the supercooled metal ring jumps almost as high as the ceiling of the lecture hall and then Ryan catches it with the metal tongs]
[audience oohs]
[Organ plays Ta-Da!]
[applause]
[Ryan Norval as Dr. Watts-On]
Okay, Sherlock, I’m with you now. The thief, he could use the electromagnet, jump up onto the train. But once he gets there, how’s he stay on? The London subway sure does move fast.
[Akire Trestrial as Sherlock Ohms]
Ah, elementary, Watts-On. If the thief already had metal rings on his shoes and if he had pre-planned the robbery, as one would do if they were about to jump in front of a train, he must’ve had some way to stay on or above the train.
He likely levitated himself.
[Ryan Norval standing in front of two large circular electromagnets that are stacked one on top of another with several inches between the bottom of the upper one and the top of the lower one]
Now, listeners, levitation, that might sound like madness, but Sherlock Ohms is a brilliant detective and later that day, we happened to find a set of train cars with a pair of magnets on the roof. Now how does one use a pair of magnets to levitate themselves? Let’s find out.
This setup is similar to the ring launcher, except instead of running a single pulse of current through the ring to project something upwards, we will rapidly alternate the current between positive or negative in the electromagnets. Similarly, instead of an aluminum ring, we have an aluminum cylinder. In the cylinder, we’ll induce a current from the changing magnetic fields. That current will feel a magnetic force from these two and levitate. Let’s find out.
[Ryan drops the aluminum cylinder in the donut holes of the electromagnets and it falls harmlessly to the top of the lab table]
[Akire Trestrial as Sherlock Ohms]
Uh, Watts-On? The magnets need to be turned on.
[Ryan Norval as Dr. Watts-On]
Right you are, Sherlock.
[Ryan turns on the electromagnets and turns up the power to the electromagnets]
This time let’s see what happens now with more power.
[Ryan spins the aluminum cylinder between the donut holes of the two electromagnets and it levitates spinning between the gap in the two electromagnets]
[audience oohs]
[Ryan Norval]
So, you see here, I’m spinning the cylinder in-between there. The magnets force it to the center. The blue one’s lifting it up. The orange one is pushing it down. I can spin it side to side, push it up and down a little bit, and as long as it stays somewhere relatively close to the center, the magnetic forces keep it held in place. And when we turn off the electricity –
[Ryan turns the power to the magnets off and the cylinder drops down to the table]
– right back to the Earth we fall.
[Organ plays Ta-Da!]
[applause]
[inset of Akire Trestrial as Sherlock Ohms]
Okay, Watts-On, back to the story.
[Ryan Norval]
Right. Sherlock Ohms and I continued to look for clues down a subway tunnel. We wandered along until we found a service door where the lock had been cut.
But the lock was not just cut, it was melted right to slag.
[Akire Trestrial]
As you might well have guessed, the thief used magnets to melt the lock.
[Ryan Norval places a large metal girdle with a small stainless-steel rod in it on the top of a large, tall electromagnet which he turns on]
Now with the levitator, we were using energy, magnetic energy to hold that up. If instead your thief with your pocket electromagnet hooked up a pair of wires to this stainless-steel rod representing a lock, we can see that instead of using the energy to levitate something, we can use that energy to heat up the stainless-steel rod.
[the stainless-steel rod in the girdle starts to glow orange hot]
[audience oohs]
[Akire Trestrial as Sherlock Ohms]
If you heat metal up enough, it will melt.
[Organ plays Ta-Da!]
[applause]
[Ryan Norval as Dr. Watts-On]
Now, Sherlock, the real question is what kind of thief has the kind of knowledge to commit this crime?
[inset of Akire Trestrial as Sherlock Ohms]
Only the vile Professor Magnetson could possible do this, using physics to rob people.
[Ryan Norval]
Professor Magnetson, didn’t you catch him last week? Wasn’t he scheduled for execution?
[inset of Akire Trestrial as Sherlock Ohms]
He was. He must’ve faked his death in the guillotine last week.
Here, let’s see how that’s possible. We just happen to have a guillotine right here.
[Ryan Norval, standing in front of a guillotine]
The guillotine is a rather simple device, medieval, one might say. It works by lifting up a heavy metal blade –
[Ryan pulls the metal blade of the guillotine to the top of the contraption and when it hits the top, the blade releases and comes down cutting a wood block in half]
– and letting gravity do the work.
[audience oohs]
[Ryan Norval]
The question then becomes how does one with a knowledge of magnets use that knowledge to save oneself from such a grisly fate?
Well, let’s say that Professor Magnetson, instead of letting the guillotine just fall –
[Ryan places a carrot on the bottom of the guillotine and set of four magnets on a steel rod at the base of the guillotine just above the carrot and then pulls the blade back to the top]
– had placed a set of magnets at the base. Let’s see what happens, then, when the blade falls this time.
[The blade of the guillotine hits the top and is released and then falls and stops right before it hits the carrot]
[Organ plays Ta-Da!]
[applause]
[Clint Sprott]
And now let’s vote with your applause for your favorite magnetism demonstration.
[Applause meter is superimposed on the screen]
Who liked the ring launcher?
[audience applauds and cheers sending the applause meter to Fantastic]
Who liked the levitated ball?
[audience applauds and cheers sending the applause meter to Amazing]
Who liked the induction heater?
[audience applauds and cheers sending the applause meter to Fantastic]
And who liked the magnetic guillotine?
[audience applauds and cheers sending the applause meter to Amazing]
[Peter Weix as the Announcer]
And now, folks, a word from a commercial sponsor.
Hi, folks, is that gravitational force getting you down? Well, then, make a motion over to Newton’s for some of the best rings in the universe. Don’t confuse them with someone else’s dark and tasteless corpuscular theories. These rings are light –
[slide titled Newtons with a photo of Saturns rings with an X through them and another photo of Newtons rings with a check mark next to it and a third photo of several superhero rings with an X through it]
– light as can be. Remember it well, listeners, Newton’s rings run circles around the competition.
[Kimberly Palladino as Dr. Sunshine Maxwell]
Hi, Professor Sprott. I’m in early, a few minutes before my show. Can I tell you about my new passion for painting? Too bad I can’t share it over the airwaves.
[Clint Sprott]
Well, actually, we’re already on the air.
[Kimberly Palladino gasps]
[Clint Sprott]
And now, it’s Late-Night Lights with Dr. Sunshine Maxwell. For those of you who are still awake, she’ll help you see in the dark.
[laughter and applause]
[Kimberly Palladino as Dr. Sunshine Maxwell]
Well, hello, my late-night nightlights. We’ve got a lot about light to learn tonight. First, can I get an energetic volunteer to come and help me?
Right here on the end in gray, can you come on down?
So, light is vibrating patterns of electricity and magnetism, and there are different wavelengths that correspond to different energies.
And what is your name?
[Young girl audience member, Teagan]
Teagan.
[Kimberly Palladino as Dr. Sunshine Maxwell]
Hi, Teagan, you’re going to help me demonstrate how energy and wavelengths are related.
[slide showing the light spectrum from Gamma rays to X-rays to Ultraviolet to visible light to Infrared light to Radio waves (Radar, TV, FM and AM). Additionally, at the top of the slide light is shown as a wave showing shorter waves with increasing energy at the Gamma end and longer waves with decreasing energy at the Radio wave end. Moreover, at the visible light spot all the colors of visible light from ultraviolet to infrared are shown with their appropriate color and wavelengths in nanometers]
First, we’re going to demonstrate short wavelengths.
Can you jump up and down really fast?
[Teagan jumps up and down fast]
Excellent.
Now we’re going to look at the other end of the spectrum and demonstrate long wavelengths. Can you jump just as high, but take a lot of time in between?
[Teagan jumps up and down more slowly]
Excellent. Now which of those took more energy?
[Teagan]
The first one.
[Kimberly Palladino]
The first one. Did it tire you out?
[Teagan nods her head yes]
Yep, that’s why I had you do it instead of me.
[laughter]
[Kimberly Palladino]
Can I get a round of applause for my volunteer?
[Organ plays Ta-Da!]
[applause]
[return to the light wavelength slide]
[Kimberly Palladino as Dr. Sunshine Maxwell, off-camera]
So, just as we saw the short wavelengths have more energy than the longer wavelengths –
[Kimberly Palladino, on-camera]
– and only some of these wavelengths are the visible light –
[return to the light wavelength slide]
– but invisible light, that means that blue light has more energy and red light has less.
Now just to the more energy side, and shorter wavelengths than blue light, is ultraviolet light, or U.V. And on the other side of red light in the infrared, or I.R., that has even less energy than red. And today –
[Kimberly Palladino]
– we’re going to see all of these different wavelengths in practice.
So, even the white light that we see from lights in the room like this, or from the sun outside, can be broken up into the different wavelength colors, and we can do that by sending it through a prism.
[Kimberly turns on an overhead projector that sends light through a prism that projects on the wall with a rainbow effect]
[audience oohs]
So, we get a nice rainbow effect.
[Organ plays Ta-Da!]
[applause]
[Kimberly Palladino]
Now I’m going to turn off this blacklight [turns off overhead projector] and bring down this fluorescent screen [pulls down screen] and we’re going to see if we can get it to light up if we use a laser with enough energy.
[House lights go down]
So first, lets – do you think my red light will get it to light up?
[Young audience Members]
No.
[Kimberly shines her red pointer light on the screen, and nothing happens]
[Kimberly Palladino as Dr. Sunshine Maxwell]
Nope, it didn’t. What about my green one?
No. What about my purple one?
[Young audience members]
Yes.
Yeah.
[Kimberly points her green pointer light at the screen, and nothing happens]
[Kimberly Palladino]
No. What about my purple one?
[Young audience members]
Yeah!
[Kimberly shines her purple pointer light on the screen and draws a smiley face with hair]
[audience oohs]
Maybe I shouldn’t quit my day job, though.
[Organ plays Ta-Da!]
[House lights come on]
[applause]
[Kimberly Palladino as Dr. Maxwell Sunshine]
Now, transparent materials don’t absorb visible light. That’s why we can see through them, and we’ve got an example of that with this laser here.
[Kimberly points to a box with a window in the front and a glowing lamp inside. To the left of the box is a laser beam creator that shoots its laser beam through a tube into the box]
So, I’ve got a blue laser and I’ve got smoke in a can –
[Kimberly opens the top of the box and sprays the smoke-in-a-can into the box where the blue of the laser light is revealed]
– that I can put in here so you can actually see the laser beam. And you’ll be able to see it in the tube here –
[Kimberly turns on the blue laser and the tube lights blue from the beam for a second]
– but it’s harder to see in the glass here ’cause this is laser protective glass. It’ll also mean that the colors of anything I put in there will look a little different, and I’m going to put this purple-colored balloon inside.
[Kimberly places a purple balloon inside the box]
And that balloon can absorb the laser light and then pop. So, let’s see what happens when I turn on the laser.
[Kimberly turns on the laser and the balloon pops]
[Organ plays Ta-Da!]
[applause]
[Kimberly Palladino]
Well, now I can use that trick to change the color of this red balloon. Let’s put it in and see what happens.
[Kimberly places a red colored balloon into the box]
I’ll turn on the laser.
[Kimberly turns on the laser and a popping sound occurs, but the balloon remains intact]
And what started as a red balloon –
[Kimberly opens the top of the box and pulls out an orange balloon surrounded by a clear balloon]
– now looks like an orange balloon.
[audience oohs and applauds]
[Kimberly Palladino as Dr. Sunshine Maxwell]
Let’s put it in one more time, though, and see what else can happen.
[Kimberly places the orange and clear balloon combo into the box and turns on the laser. The clear balloon stays intact, but the orange balloon pops inside the clear balloon]
[Kimberly Palladino]
And what we’ve got left –
[Kimberly opens the top of the box and pulls out the clear balloon]
– is a clear balloon.
[organ plays Ta-Da!]
[Kimberly Palladino]
It didn’t absorb the laser light and so it stayed whole.
[Kimberly places the clear balloon back into the box and moves to the front of the stage with an infrared camera in her right hand]
Now I’m going to need two more volunteers. Hello. So, we’re going to come down and you guys can hide behind this piece of plastic here.
[Two young volunteers come down from the audience and set up behind a sheet of plastic that has been draped over a long metal pole on a stand]
[Kimberly Palladino]
And we can’t see them in the visible light, ’cause the plastic blocks it, but in the infrared, we can see through that plastic with no problem. Can you guys wave to us?
[the image from the infrared camera is displayed showing the two volunteers behind the plastic sheet]
[Kimberly Palladino]
Excellent. You can look up and see what you look like also.
[two volunteers look up at the video screens and the audience laughs]
[Kimberly Palladino]
Fantastic. Now stick around –
[Organ plays Ta-Da!]
– while my colleagues change the screen for a second.
Now one of the other things I can do with my I.R. camera is check myself out in the mirror, and to make a mirror, all I need is this dull piece of stainless steel.
[Kimberly shines her I.R. camera at a large piece of stainless steel that is at the back of the room and the image of her and the audience behind her appear in the video monitors in the room]
All the scratches that mean it’s not a very good visible mirror don’t affect the longer wavelength in infrared, and so, ah, I look pretty good.
[Organ plays Ta-Da!]
[applause]
[Kimberly Palladino as Dr. Sunshine Maxwell]
Okay, now I’m going to give my volunteers two squirt bottles of water, one warm and one cold, and now they get to be the artists.
[Kimberly picks up two squirt bottles of water and walks behind the sheet of plastic on the metal pole and hands them to the volunteers behind the plastic sheet]
So, you guys can each take half of the screen and draw whatever you’d like on it. The bigger the better. Remember, if you want to write something, we see it backwards so it might just be easier to draw something. Ah, and they’ve already started.
So, in bright yellow, you’ll see the warm water –
[view of the sheet of plastic from the infrared camera on the screen and on the left side is an image of a circle in dark purple and on the right side is the image of a yellow heart]
– and in the dark purple, you see the cold, and you’ll also notice in the warm water, as it evaporates, what’s left behind is cooler –
[the yellow heart outline slowly changes from yellow to purple as the hot water cools while inside the heart is yellow as the volunteer fills in the heart]
– and so, it switches from bright yellow to dark purple.
[Organ plays Ta-Da!]
[applause]
[Kimberly Palladino]
Fantastic. Thank you to my wonderful volunteers. You guys can leave the water back there and come take a bow.
[two volunteers come out from behind the curtain and take a bow]
[audience applause]
[organ plays Ta-Da!]
[Kimberly Palladino]
Well, looks like the sun is coming up and that’s all for our Late Night Lights. Join us next time for another light adventure.
[applause]
[organ plays Ta-Da!]
[Clint Sprott comes to the microphone as an Applause Meter is superimposed on the screen]
And now let’s vote with your applause for your favorite light demonstration.
Who liked the fluorescence demonstrations?
[audience applauds and the Applause Meter moves to Not Bad]
Who liked popping the balloon with a laser?
[audience applauds and cheers with the Applause Meter moving to Pretty Good]
And who liked the infrared camera?
[audience applauds and cheers with the Applause Meter moving to Amazing]
And now, Peter, which demonstration got the most applause for the entire show?
[Peter Weix as the Announcer]
Well, listeners, I’ve been judging closely and monitoring the applause meter, and the winner is the paper toilet gun. Toilet paper gun!
[audience laughs and applauds]
[Peter Weix]
It’s wiped out the competition.
[audience groans]
[Clint Sprott]
Oh. Now some of you may be thinking, we’re just pretending to broadcast this, but in fact, if you happen to have a portable radio, as I do here –
[Clint grabs a portable radio from a lab table]
– if you take it and tune it to 550 kilohertz, down at the bottom of the A.M. band, you should be able to hear us broadcasting.
[Clint hands the portable radio to Peter who attempts to tune it]
And to prove that I’m going to turn off my wireless microphone –
[Clint turns off and removes his wireless microphone and sets it down on the table while the radio in Peters hand starts buzzing]
– and take this old-fashioned microphone from 100 years ago –
[Clint takes the old-fashioned radio microphone and speaks into it]
[Clint Sprott speaking into the microphone, and it is amplified through Peters radio]
– and show you that we are indeed broadcasting. This is 9XM broadcasting from the basement of the physics building at the University of Wisconsin, just as was done for the first time 100 years ago.
[organ music plays Ta-Da!]
[applause]
[Clint Sprott sets down the old-fashioned microphone and returns to the front of the stage]
Now when I – when I started doing these shows 35 years ago, I had no idea I’d be doing it 35 years later. But our audiences have been extraordinary, and we thank you for listening over the years. But we need your help in order to continue the programs for many years to come. Please go to wonders.physics.wisc.edu –
[slide titled Donate appears on-screen with the website to donate and a Q.R. code to scan to donate]
– click on donate for your tax-deductible contributions.
[Clint Sprott]
And now I’d like to end the show the way we have ended every one of the shows over the past 35 years, by making for you a cloud.
For this, we use 25 liters of liquid nitrogen.
[Clint rolls out a container of liquid nitrogen that has a pipe on top of it]
It’s very cold stuff and we force nitrogen gas into the container, that forces the liquid up into this pipe on the top. This pipe has several dozen small holes. The liquid nitrogen will come out very, very cold, come in contact with the air, and the water vapor in the air will condense into tiny droplets of liquid water, and that’s what we call a cloud. And so, with that, I will put on my hat –
[Clint puts on his top hat]
– and thank you all for coming.
[Clint turns on the liquid nitrogen contraption and the stage is filled with cloud-like water droplets while the entire cast comes to the front of the stage to take bows]
[whimsical music]
[applause]
CREDITS
Executive Producer and Host: Clint Sprott
Coordinator: Peter Weix
Captain R.O. Dynamic: Mike Randall
Dr. Calvin Scale: Terry Craney
Rileigh Rayleigh: Emily Ehlerding
Mr. E.: Michael Winokur
Dr. Watts-On: Ryan Norval
Sherlock Ohms: Akire Trestrial
Dr. Sunshine Maxwell: Kimberly Palladino
Technical Support:
Tara Keenan
Jim Moran
Steve Narf
Paul Nonn
Steve Oliva
Jim Reardon
Bill Zimmerman
Theme Music: Jim Latimer and Frank Ferriano
Cameras:
Greg Cichy
Leigh Drogemuller
Brandon Duerst
Steve Narf
Audio:
Chad Raby
Steve Narf
Akire Trestrial
Editing: Leigh Drogemuller
Copyright 2018 University of Wisconsin System Board of Regents, Department of Physics – University of Wisconsin-Madison.
All Rights Reserved
ICS: Instructional Communications Systems
Search University Place Episodes
Related Stories from PBS Wisconsin's Blog

Donate to sign up. Activate and sign in to Passport. It's that easy to help PBS Wisconsin serve your community through media that educates, inspires, and entertains.
Make your membership gift today
Only for new users: Activate Passport using your code or email address
Already a member?
Look up my account
Need some help? Go to FAQ or visit PBS Passport Help
Need help accessing PBS Wisconsin anywhere?
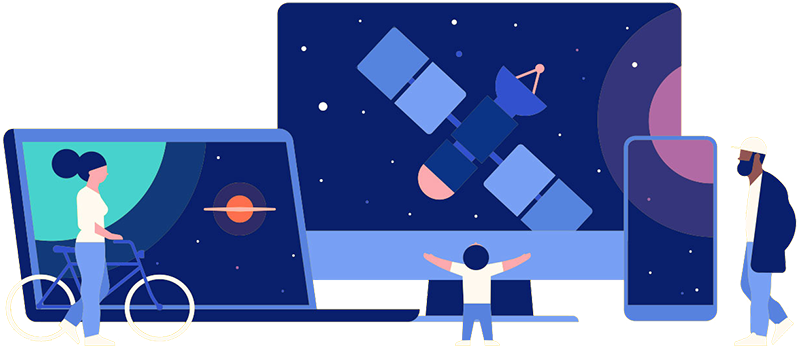
Online Access | Platform & Device Access | Cable or Satellite Access | Over-The-Air Access
Visit Access Guide
Need help accessing PBS Wisconsin anywhere?
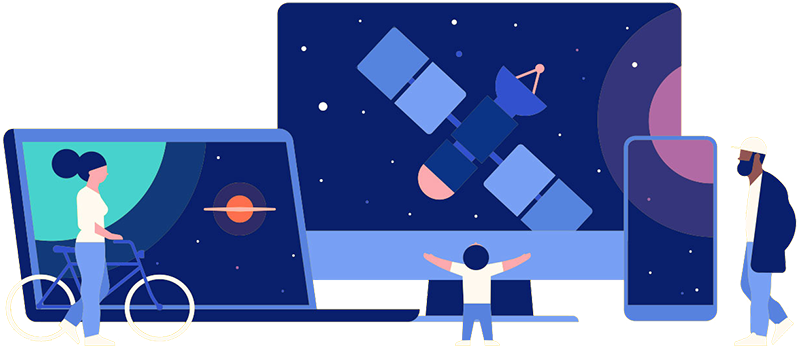
Visit Our
Live TV Access Guide
Online AccessPlatform & Device Access
Cable or Satellite Access
Over-The-Air Access
Visit Access Guide
Follow Us