cc >> You’re trying to tell me this is Professor Sprott frozen in this block of ice? >> Oh, yeah. >> And you’re going to get him out of this? >> Oh, yeah. >> And you know what you’re doing? >> Oh, sure, I got tons of directions right here. >> And you’re fairly experienced at doing this? >> Oh, sure, I invented this thing. >> You have lots of credentials to prove it? >> Oh, sure. Actually, we’re almost there. All I got to do is give it a good smack. >> That always takes care of it. >> All right, so this’ll be it. >> This is it, huh? >> Safety first.
(loud pops) >> Whoa! >> Oh, no! >> What did you do? >> That was my — It just exploded. I’m never gonna get another one. >> Now what are we going to do? >> Oh, man, we’ve got to think. We definitely, uh, got to thaw him out real slowly, ’cause otherwise all his internal organs are just going to frazzle, and then he’s gonna die. >> No, that’s not any good. >> Uh, okay, um, oh! I’ve got an electric blanket in the back. We’ll plug it in, throw it over him, turn it on real low. A couple hours, no problem. >> Whoa, wait! A couple hours? We don’t have a couple of hours. These people want to see a show now! Right? >> Yeah! >> All right.
I’ve got somebody else who can help us. ET-2, step aboard! >> Yes, sir. >> It seems that Professor Sprott is frozen in this block of ice here. Do you have anything that might help us out? I have just the thing. >> Great. >> A high intensity laser. >> Ha-ha! Lasers! >> You can’t use that! >> No, it’s fine, I’ve got goggles. >> You’re just gonna blow a hole right in the middle of him. And you know what? A frozen Sprott is much better than a gutless Sprott. >> Well, you know, he’s not doing much good to us this way.
So we’re going to try the laser. All right, ET-2, go ahead and fire. You know, folks, in order to fire the laser, we’re going to need a countdown for this one, though. So do it on a three. >> Three, two, one, fire! (electronic sounds) >> Ah-ha! It worked! Ladies and gentlemen, get prepared to irrigate your mind with the man who will open the floodgates of information, present a steady stream of distilled but undiluted amazing feats of physics, quench your thirst for imagination and drench your imagination. May I present to you Professor Clint Sprott! (applause) >> Welcome to the Wonders of Physics. Now you all know I didn’t really come in on a block of ice, right? But I do want to talk to you today about ice, and more generally, about water, because water is a form of ice. It’s ice in the form of a solid. And I have a little bit of ice right here, a block of ice. I’m going to do something a little unusual with it.
I have here a very thin wire, like you might find on a piano. And I’m going to drape this over the block of ice, like so. And I’m going to hang a very heavy weight on the bottom. It’s very heavy. And you may know that when you put pressure on ice, it causes it to melt. That’s what happens when you make a snowball. You take snow, and you compress it really hard, some of the snow melts and then when you release the pressure, it re-freezes, and you have a nice snowball that you can throw. Well, that’s what’s happening here. The weight is going to pull on that wire, and it’s slowly going to melt the ice. And maybe before for the end of the lecture, it will melt its way all the way through.
And the interesting thing is, it’s going to, I hope, re-freeze above where it went through. Now, that of course, is water in the form of a solid. Water, normally, is the form of a liquid. And here I have a little beaker with some ordinary water in it. Do you think I could pull this cloth out from under the beaker without spilling it? You think I could? Well, let’s try. (laughter) That’s not how you do it, is it? What am I doing wrong? What? Tug it? Fast? (audience yelling out suggestions) Okay! (musical flourish) (applause) Well, that’s right. You’ve probably often seen people do that. But you may not know that’s because of a principle of physics called the principle of inertia. Inertia is the property of an object that tends to make it remain at rest. So, the water was initially at rest.
When I went to pull out the cloth, it tended to remain at rest. I can illustrate another property of inertia using water. Here I have a bucket filled with water. Do you think I can turn this bucket upside down over my head without spilling the water? >> Yeah! >> You think? (laughter) That’s not how you do it, is it? How do you do it? Spin it? Fast or slow? >> Fast. >> Fast. You’re sure, now? Well, I hope you’re right. (musical flourish) (applause) That’s right. That’s because of the principle of inertia, also. When something is moving, it tends to continue moving in a straight line with a constant speed. And the water, if I hadn’t been pulling on the handle of the bucket, would’ve gone flying off on a tangent.
But because I was pulling on the handle, I was able to make it go around in a circle. So, the inertia of the water is what kept it from spilling out. There’s another use for water. Did you know you could use water as a propellant for a rocket? Well, I have here a little toy rocket. And it’s got about that much water in it. And up here is air. What I’m going to do is increase the air pressure by just pumping more air into it. And then I’m going to release it and the air is going to come out the back of the rocket and take some of the water along with it. Let’s see what happens. (musical flourish) (applause) It went all the way across the room.
That’s because of a principle of physics called action and reaction. The water going that way was the action. That made the rocket go that way. That was the reaction. That’s a very important principle of physics, because that’s how rockets work. Now, this principle of action and reaction is one that’s familiar to all of you. Do you some of you have at home something that looks like this, that you maybe use out on your lawn on a hot day in the summer? It’s a lawn sprinkler. And if those of you in the front row will duck, I’ll turn on the water. And it goes around. Except there’s no water coming out, because I have it connected, not to water, but to compressed air.
But the idea is the same. When the air comes out these little nozzles around the outside, that’s the action. And the reaction is the lawn sprinkler spinning around like that. And of course, if water had come out, you could water your lawn that way. So that would be a very useful gadget that many of you have at home. Here’s another demonstration of the same idea. I have a flask with a little bit of water in it. And it also has some nozzles here. The nozzles look very much like the nozzles on the lawn sprinkler, except in this case, there’s no way for the water to come out. However, if I heat the water, I could cause it to boil, and I could make steam in here.
Then the steam could come out the nozzles, and it would be very much like the air coming out over here. In order to that, I have to make the water boil. The easiest way to do that is to light a burner underneath the water that will slowly bring the water to a boil. This will take a little while for it to begin to boil, so we’ll just watch that and then we’ll come back to it in a few moments after it’s boiling. >> Hey! >> Oh, my goodness! Who are you? >> Excuse me, I’m just passing through. >> Oh, I see. You seem to have a little bit of a problem here. What is your problem? >> You know, I do have a problem. I just can’t walk straight. Every time I try to walk straight, I veer off to the right.
You know, ever since I moved up here from Argentina. >> Well, I’ve never heard of that. >> Down there, you know, I used to veer off to the left. >> Hmm. >> My doctor says I have coriolisitis. >> I’ve never heard of that. But I have heard of the coriolis effect. Is that related? >> Coriolisitis is a hypersensitivity to the coriolis effect. >> I never knew that could effect people. But I was about to show a demonstration of the coriolis effect.
Would you like to see it? >> Sure. >> It’s right over here. And what we have is a stream of water that comes out. There’s a little pump here that makes a stream of water come out. You can see it on the video there, the stream of water right there. It’s on a rotating table. And you can imagine this being the earth rotating. And when I rotate it around, and you view it from a camera that’s rotating with the water, if it rotates that way, you see the water curves to the right. If I rotate it the other way, the water curves to the left. That’s due to the coriolis effect.
And it’s exactly what happens when the earth rotates. If the wind blows across the earth, in the northern hemisphere, as the earth rotates, it causes the wind to veer to the right. In the southern hemisphere, it veers to the left. That’s why hurricanes go around in the direction they do in the northern and southern hemispheres. >> I knew it! That’s why I’ve been having trouble. That’s why I can’t make my toilet flush in the other direction. (laughter) It must be this coriolis effect you’re talking about. I must just have to move to the southern hemisphere. >> You know, actually that’s a common misconception. The coriolis effect is only strong enough to affect people who have fictitious diseases like mine.
Normal everyday things like toilet and bathtubs, you know, they’re not affected by the coriolis effect. That’s just how your toilet’s designed. So your only option really is to buy a new toilet. Although I do happen to be a plumber, and my specialty is the anti-coriolis commode. (toilet flushes) (musical flourish) (applause) >> Well, you may have heard that the bathtub drains one way in the northern hemisphere and another way in the southern hemisphere. It’s not true. The coriolis effect is very small, unless you’re talking about something very large like a hurricane. But look at our little device going around here just like the lawn sprinkler. It’s doing so because steam is coming out of these nozzles. This is a device that was invented over 2,000 years ago by a Greek scientist named Hero.
It was so long ago that they didn’t even have last names. He was Hero of Alexandria, which is where he lived. This is one of many inventions he came up with. So it illustrates properties of water and also the physics of motion. In particular, action and reaction, which of course is a very important principle of motion. Another scientist that lived a long, long time ago, even before Hero, in fact, was a man named Archimedes. He was one of greatest of all scientists. And he lived about 200 BC. And he thought about properties of water. He wondered what would happen if you had something with water in it, as we have here.
We have an aquarium filled right up to the top. In fact, I want to be sure it’s all the way to the top. One way to do that is to put a little more water in there, till it just starts to spill over, and then we stop. Archimedes thought about the following question. If you lower something into the water, like this block of wood, how much water spills over? Well, let’s try it. You see, indeed, some water spills over the edge. Well, Archimedes reasoned that the amount of water that spilled over is exactly the same as the volume of the wood that’s beneath the water, right? Because that’s where the water was before it spilled over. But you also notice, that when something is floating in the water, it’s as if something is holding it up. Something is pushing upward on it. Because if the water weren’t there, the block would just fall to the floor.
And that, we call a buoyant force. Archimedes was clever enough to realize that that upward force holding up the block is exactly equal to the weight of the water that spilled over. And this is true, whether it’s something floating or something that sinks. So imagine we have something that’s more dense than water, like this cylinder of aluminum. Now, if we lower this into the water, again, some of the water spills over. And in fact, the amount of water that spills over is exactly the same as the volume of the aluminum. In this case, there’s also an upward buoyant force, but it is not large enough to support the weight of the cylinder. So eventually, it would just fall to the bottom if I let go of it. However, the upward force on this is also just equal to the weight of the water that spills over. That’s Archimedes principle.
And Archimedes discovered that, or figured it out in a very odd way. Archimedes lived in Sicily. And the king of the city he lived in, in Syracuse, was presented with a crown. They told the king the crown was made out of gold. The king was a little suspicious that maybe it wasn’t really gold. That maybe the goldsmith had kept some of the gold, and made it out of something else that just looked like gold. So he knew Archimedes was a really smart man. He was 22 years old at the time, by the way. And so, the king gave Archimedes the job of figuring out whether that crown was really made of gold. Well, Archimedes was able to weigh the crown, and so forth.
But he didn’t know how much metal there was in the crown. Then, one day, he was in his bathtub. When he stepped into the bathtub, he realized some water spilled over. And he said, well, the amount of water must be the same as the volume of the crown. So, by lowering the crown into the water, he would therefore be able to find out how big the crown was, and thus from its weight, he would be able to figure out whether it was really gold. Well, the story has it that Archimedes was so excited when he had this idea that he jumped from his bath and ran through the streets without bothering to put his clothes on apparently, yelling “Eureka! Eureka!” which is Greek for “I have found it, I’ve discovered it.” So, that’s how Archimedes Principle was discovered. It turns out that the crown was not, in fact, made of gold. And somebody probably got in big trouble for that. But it does illustrate properties of water. Over here, I have another flask with some water in it.
This is liquid water. Do you know the temperature water boils at? Who knows? 212 what? Degrees Fahrenheit, or– Oh, my goodness! Let’s stop and talk about this. How many of you have been to Yellowstone Park? You know in Yellowstone Park they have things called geysers. Have you seen those? This is our artificial geyser. And it works by the same principle. I was about to ask what temperature water boils at. And someone said 212 degrees Fahrenheit. Or in Celsius? 100 degrees Celsius. Except, you know, that’s not quite right. That’s right only at atmospheric pressure, only at sea level.
If you live in a place like Denver, water will boil at a lower temperature. And conversely, if you put water under pressure, it boils at a higher temperature. That’s what’s we’re doing here. We have a long column of water. So, down at the bottom, the water is under pressure, just like if you go down in your swimming pool, you feel the pressure of the water, if you’re down deep. And so, the water is being heated by these burners. And it heats up to a temperature higher than it would normally take to boil water, because of the pressure. But eventually, it begins to boil, and then pressure is relieved, and it boils very vigorously. And it shoots the steam and some of the water as well up into the air. It comes in contact with the cool air.
It converts back into liquid water and it runs back down in here, and the heating begins again. This is just what happens in Yellowstone Park. There are crevasses that run deep down into the earth. Rain water runs into the crevasses. The rocks deep under the earth are very hot, hot enough to cause the water to boil, and it shoots the water up. And when it cools, it collects, and the heating begins again. This will go off every few minutes for the next little while, until eventually, I turn it off. But the same principle is actually in effect over here. Because in this case, I have some water in a flask. And what I would like to do, is not increase the pressure, as we did over there, but I would like to decrease the pressure.
Well, I can’t take the flask to Denver very easily. But we can reduce the pressure using a vacuum pump. So, if I turn on the vacuum pump, you will see from the gauge here that the pressure is coming down near zero. And you’ll see the water boiling. It boils very vigorously. Now, normally, when water boils, you expect it to be very hot, right? But I can assure you, I can touch this and it doesn’t feel hot at all. And in fact, it feels quite cold. That’s rather peculiar, that water boils by getting colder. You don’t normally think that would happen. Now if you look, something even more interesting has happened.
If you look carefully, you will notice that ice has formed. And indeed, if I put my hand on it, it feels quite cold, just like a glass of ice water. And indeed, that’s what it is. It’s a mixture of ice and water, and very little gas. In fact, I can let the air back in, and we’re left with a flask of mostly ice in it. Now that’s amazing. We were able to boil water, and then we were able to freeze it without any source of heat or refrigeration. How did we do that? To boil water, does require energy. Normally, when you boil something– like that– you have to apply heat, as we’re doing down below with the burner. Or, you put it on a burner on your stove and provide the heat.
But over here, we weren’t heating it. But the energy had to come from somewhere. The only place it could’ve come from was the water itself. Now, when you add energy to water, it gets hotter. When you take energy out of water, it gets colder. And that’s what happened there. When we boiled it, the heat, the energy required to make it boil came out of the water, lowering the temperature of the water all the way down to the point where it froze. That’s pretty amazing when you think about it. But it does illustrate water in all three forms, solid, liquids and gas. Now, another example of boiling water.
Have you ever had a pan on your stove at home, and you got it really not without anything in it? And then you took a few drops of water, maybe on your finger, and you sprinkled them on the pan? Does it boil? It doesn’t, at least not very much. What you notice is little droplets of water that dance around on the top of the pan. Have you ever seen that? Well, I don’t have a pan here. But I do have a hotplate. It’s been turned on, and it’s nice and warm. I have here a beaker with a little bit of water in it. I have an eye dropper, so I can make little droplets of water. I’m going to take some of these droplets and put them on the hotplate. You’ll be able to see on the television monitor. Those little droplets of water sort of dance around, and eventually roll off the side of the hotplate.
Now, why aren’t they boiling? Well, in fact, they are boiling. But they’re boiling very slowly. And what happens is, the droplet of water begins to boil, and that forms a layer of steam. So, some of the steam is between the water droplet and the hotplate. Now, steam is not a very good conductor of heat. So, as a consequence, it insulates the water droplet from the hotplate, so the water droplet doesn’t boil, at least not very rapidly. It does boil very slowly, but it takes a long time for that water to boil, even though the hotplate is quite hot. Now, next time you see that, you’ll know why that is happening. But you’ll also know the name for it, because this is called the Leidenfrost Effect. It’s named after a man named Leidenfrost.
But anyway, it is a familiar idea that involves water in the form of a liquid and a gas. Now, water can also move from one place to another, like flowing through a pipe. We actually have here a pipe with water flowing through it. The water is flowing in this end of the pipe, and it’s flowing out that end and down into the drain over there. You’ll notice this is a peculiar pipe, because it’s small here, and then it’s large, and then it’s small, and then it’s large again, and so forth. So the pipe keeps changing its size– It always hiccups at the end. I’m going to ask you a question. When the water flows through the pipe, do you think it flows fastest when the pipe is big or when the pipe is small? Small? You think the water flows fast in the small pipe? You sure? Well, in fact that’s right. And an analogy is when you’re driving down the highway, and it’s a nice big four lane highway, and up ahead there’s some construction and it narrows down to one lane, where do the cars move slowly? It’s when they’re coming up, before they arrive at the construction. Everything slows down and says, well, there must be some problem up ahead.
Once you get into that one lane, then you move nice and fast. And the reason is the same for water or for cars. If you have a lot of water moving through a big pipe, it can move rather slowly, but once it gets to the small pipe, to get that large amount of water through, it has to move fast. So, that’s exactly right. We can actually demonstrate that with this apparatus, because it has a bunch of little tubes sticking up vertically above the different sections of the pipe. I’m going to put a little red dye in each of these tubes, just so you can see how high the column of water is going. And you’ll notice a peculiar thing. That all of these are not going to the same height. What that means is, where these things are high, that means the water pressure is large. And where they’re low, that means the water pressure is small.
What this tells you, then, is that the pressure is high when the water is moving slowly, but the pressure is low when the water is moving fast. And that may not be quite so obvious, but the faster something moves, the less pressure it exerts on the walls of the tube that it’s moving through. This was something that was discovered or thought through by a Swiss scientist named Daniel Bernoulli, in 1738, he explained that. And in his honor, this is called the Bernoulli Effect. And it’s a very important phenomenon, because this is one of the principles in action when an airplane flies. When the air moves over the top of the wings, going very fast, it reduces the pressure there, and that gives some lift to the airplane, much like the buoyant force– (fire siren blaring) >> All right, everyone, just remain calm. We’re going to get through this safely and efficiently, okay? We need to evacuate this room. So, we’ll start right here with you folks in front. Go ahead, stand on up. >> Wait a minute! What are you doing? >> I’m rescuing these people, of course, from the unstable fire house.
>> The what? >> The unstable fire house. I heard on the radio, they said there was a fire house instability here. >> No, I’ve never heard of such a thing. But I was about to talk about something called the fire hose instability. >> Fire hose instability? Why didn’t they say so? >> Well, would you like to see it? >> Sure. >> If somebody had a garden hose, and you connected it up to water, you turn on the water really fast without holding onto it, what does it do? That’s right, it goes all over, doesn’t it? And that’s what I’m going to show you. Here I have a garden hose, well, it’s really just a rubber tube– >> I knew this place wasn’t safe. >> Don’t pay any attention to that. We’ll clean that up later. I’m going to turn the water on in this rubber tube.
And you notice the tube moves back and forth. That’s not really instability you’re seeing there. In fact, eventually, it’s just oscillating back and forth, sort of like a mass on the end of a string swinging back and forth like a pendulum. But if I turn up the air pressure, in this case, a little bit, you can see a much more complicated kind of motion. And that’s called the fire hose instability. >> Ah-ha! I know all about these things. We have to deal with them every day. And I know just what to do to stabilize it. If you want to keep this fire hose down, all you’ve got to do is hold on real tight. There we go, try that.
>> Okay. (turns up air pressure) >> He’s right, that did stabilize the instability. >> But that’s a pretty wimpy fire hose. Do you guys want to see the real thing? All right! Ready? Oh, no! I think it’s broken. I better go get this fixed, or I might get– fired. (musical flourish) (applause) >> It’s true, the fire hose instability, as you could imagine, if you ever did that with one of those big fire hoses, with no one holding onto it, it would flail around, and it could be very dangerous. It could injure someone. But that’s an example of a special kind of motion that’s called chaotic motion. It’s moving around in a very complicated way that never repeats. I can show you another example of chaos here that also uses water.
This water has a little blue coloring in it, just so you can see it a little better. This is one of my favorite demonstrations, because you can all do it at home. Maybe you have a faucet or something in your bathroom or your kitchen. Open it so it drips very slowly. It’s going to drip into a little pie tin down here that has a microphone next to it, so you can hear the drip. (water dripping) Can you hear it? Now you notice this is dripping in a very regular, repeatable manner, kind of like a clock ticking. But I’m going to turn the drip rate up a little bit. Listen to what happens. Now it’s not regular at all. It’s very irregular.
Let me turn it down again. It’s regular again. That’s another example of chaotic motion. If you do this at home, I would encourage you to do the following. Explore the transition, where it stops being periodic and becomes chaotic. What you should observe is something called a period doubling bifurcation. A big, long word, but what it means is you should hear something that sounds like drip-drip, drip-drip, drip-drip, drip-drip. It should repeat every second drip. Instead of every drip being just like the one before it, it’s like the one two before it. Then if you turn it up a little more, and it takes a lot of patience, and it’s very hard to do this, but you can try, you will hear it repeat every fourth drip.
It’ll sound something like drip-drip-drip-drip, drip-drip-drip-drip, drip-drip-drip-drip, drip-drip-drip-drip. Then it will repeat every eighth drip, every 16, every 32nd, and so forth, until very soon, it is never repeating. It takes an infinite time before it repeats. That’s what we mean by chaos. Have you ever been to the jeweler, where they have something called an ultrasonic cleaner? You put your rings and your bracelets and things in it. It’s a little bath of water, and usually some kind of detergent. But here we just have ordinary water in an ultrasonic cleaner. When I turn it on, you’ll be able to see on the television monitor little waves on the surface of the water. That illustrates that something’s happening, but it’s not obvious that anything much is going on. However, I have here a bottle with water in it.
And it’s a special kind of water called carbonated water. You’re familiar with this. You’ve all drunk things like this, a carbonated beverage. And it has carbon dioxide dissolved in the water. You probably know that if you shake it and then take the top off, it fizzes. Well, I’m going to take the top off. I don’t know whether this one is shaken, but you can see that some of the carbon dioxide is coming out of solution. I want to do it slowly enough that when I take it off, it doesn’t bubble over. So, we are reducing the pressure, and some of the carbon dioxide that was previously dissolved in the water is now coming out of solution. But there’s still some carbon dioxide in there, and you see some of it is still coming off.
What I want to do now is put a little nozzle on here, just like you would find on a shampoo bottle, or something. It just lets the water come out rather slowly. I’ll put it in the ultrasonic cleaner, and then turn it on. Let’s see what happens. (musical flourish) (scattered applause) That’s evidence that sound is a form of wave. It’s just as if I was shaking the bottle really vigorously, except I wasn’t shaking it, the sound waves in the ultrasonic cleaner was shaking it. That was causing some of the carbon dioxide to come out of solution. So, that’s an illustration of some uses of water to do things with sound. Another thing that involves water is electricity. Is water a good electrical conductor? You think so? Who thinks not? Well, you know, we don’t have to just argue about it, we can actually test it.
Here, I have some ordinary water, ordinary tap water, right out of the faucet. And in order to see whether that’s a good electrical conductor, I’m going to use something that’s quite familiar. It’s a light bulb, a rather big one. It’s a 200-watt light bulb. Usually, when you have a light bulb, you plug it into the wall, as this one is, and there’s a switch here. When you turn on the switch, the light bulb comes on. We don’t have a switch. We just have two wires. I’m going to use the water as the switch. I’m going to lower this down into the water.
And if the light bulb comes on, that means the water is an electrical conductor. Let’s try it. Did the light bulb come on? Well, look carefully. It comes on, but not very bright at all, right? The water is conducting some electricity, but not very much. I can make it conduct more electricity if I take some ordinary table salt, sodium chloride for you chemists. If I put a little bit of that in the water, just a few sprinkles, and I’ll stir it, now you see the light bulb getting brighter. The more salt I put, the brighter it becomes. So, saltwater actually is quite a good electrical conductor. But ordinary tap water is not a very good electrical conductor. It does conduct, but not all that well.
By comparison, I have another beaker over here with the tiniest amount of sulfuric acid, so little that I could in fact safely drink it, but I’m not going to do that. I do want to lower this down into the sulfuric acid and show you. So that makes a very good electrical conductor indeed. (electronic sounds) Excuse me, who are you? >> My name’s George. I come from a planet that’s about 37 light years away. >> What brings you to Earth? >> I heard all about Earth from Buck Rogers, the 21st century radio show. >> He hasn’t been on the air for 75 years. >> Yeah, it took me a long time to get here. But the trip wasn’t boring at all, because I had my iPod. We’ve had iPod technology for millennia.
>> I see, what brings you here to Earth, George? >> My planet ran out of clean water to drink, so I came here looking for some water. >> Hey, is this safe to drink? >> Well, you know, that water looks awfully dirty. I think that has a physical problem. I wouldn’t drink that. >> Oh, yeah, okay. Here, can I drink this? >> You know, that has kind of a funny color to it. That could have some strange chemical in it that might not be safe. >> Hmm, I see that. Oh, okay, okay. This doesn’t have any particles or color.
I can drink this, right? >> Well, you know, that water does look rather clean, but that could have a biological problem. That could have microorganisms in it that could be very dangerous to you. >> Huh, well, then how do I know that the water is safe to drink? >> You know, there’s no way to know by just looking at the water. You actually have to go into the laboratory. You have to test for physical contaminants, chemical contaminants, and biological contaminants. It’s not easy to test water. >> All right. I’ve got to get back to my planet though, because I’ve got to tell them to stop drinking the water out of the– (toilet flushing) coriolis commodes. (applause) >> Okay, well, it is true that a lot of water is not very clean and pure to drink. But some water is, and fortunately, we have good water that we can drink here.
But water does conduct electricity. And do you know what water is made out of? Who knows? Yeah? H2O, and what is “H”? Hydrogen. And what is “O”? Oxygen, that’s right. We can separate water into hydrogen and oxygen. And we do it using electricity, the same kind of electricity we have over here. Here we have a little U-shaped tube. It has in it some water. It’s colored red, so you can see it a little bit better. I’m going to connect it back here to a source of electricity so that one side of it is positive and the other side is negative. And you can see some bubbles rising, in fact, on both sides.
And what we’re doing is making hydrogen and oxygen right from the water. We’re separating the water into the hydrogen and oxygen. The hydrogen and oxygen, of course, are gases. So, they are forming up in this region up here. You think this is the hydrogen or the oxygen? Oxygen? Hydrogen? Well, let’s think about it. Water is H2O. That means H-2, means there are two atoms of hydrogen for every one atom of oxygen. So the one that is making the most gas here must be the hydrogen. And in fact, if you look carefully, it’s making exactly twice as much as it’s making oxygen. Of course, I could just be kidding you, maybe that’s not hydrogen at all.
How could we show that it’s really hydrogen? Light it on fire? Oh, my goodness! (laughter) Well, you’re right, hydrogen does burn. I have a little candle here. I’ll light the candle. And we need to now take some of the hydrogen and put it over the candle and see if it burns. How are we going to do that? Well, I have a little, tiny beaker here. And what if I pour some of the hydrogen in it, and then walk over and pour it into the flame. Would that work? That wouldn’t work? That’s right, because hydrogen is less dense than air. You can fill a balloon with it and it goes up to the ceiling, so I couldn’t really carry it around in a thing like that. But here’s an idea. What if I turn this upside down and put the hydrogen in it.
Then it would try to rise, it would have no where to go. Then maybe I could carry it over to the flame. That would be evidence it’s hydrogen for two reasons. First of all, because it’s less dense than air. And secondly, if it burns, that would be pretty strong evidence, wouldn’t it? So, as we always do, when we work with chemicals, I put on my safety glasses. I’m going to let some of this gas out into this little beaker, upside down, trying not to get any water in it. So now we claim I have hydrogen in here. Let’s see. (small pop) Did you see that little puff? The hydrogen actually ignited. It was a tiny explosion in there.
Over here, I claim we have oxygen. How can we test that? Burn it? Well, oxygen doesn’t burn. You need oxygen to make a fire. In fact, that’s what burning is, you combine things with oxygen and they burn. So, oxygen makes things burn better. So, if we set something on fire, like this little popsicle stick, get it burning, and then if we put it under the oxygen, the oxygen should make it burn even better, right? Here we’ve got this burning. And to make it even more dramatic, I’m going to blow this out and then let the oxygen flow onto it. (musical flourish) (loud noise) Oh, goodness! Look what happened over here! This piece of wire went all the way through the ice. And look at the ice. It re-froze behind it.
And that’s just like as I said with a snowball, where you make it melt by putting pressure on it. And then you relieve the pressure and it refreezes. Okay, now there are other ways to make energy from water. Did you know that not all water is H2O? One of about every 6,000 molecules of water, instead of hydrogen, there’s something called deuterium. That’s an isotope of hydrogen. If you had pure deuterium oxide, you would call it heavy water, and people can make that. But there’s an interesting thing you can do with that little bit of deuterium. If you start with a gallon of water, right out of the lake, or the river, or the faucet in your kitchen, and you extract all of the deuterium, it’s about an eighth of a gram of deuterium. If it were in the form of a little ice crystal, it would be like a grain of sand. If you put it on the tip of your finger, you can barely see it.
And yet, there’s as much energy in that eighth of a gram of deuterium as there is in 300 gallons of gasoline. And it came from one gallon of water. The only problem is you have to burn it at a really high temperature in something called a nuclear fusion reactor. You have to burn it at a temperature of 100 million degrees. That’s hard to do. But some day it will be done, because we know the stars work on that principle, and they work. So some day, we’ll be able to harness that energy, in a few decades, perhaps in the lives of many of you. There’s yet another way we can make energy from water. In fact, we do it on a large scale today. That’s called hydro-electric power.
If you dam up a river, and then you let the water flow over the dam, and the falling water turns a turbine that is then connected to an electric generator, you can make electricity. Well, I can’t dam up any rivers in here, but we do have some water in that tank way up near the ceiling. And we can let it flow downhill, over this water wheel, make the water wheel go around. That’s connected to an electric generator, which will light some lights up here. Let’s turn on the water. You see the water wheel go around. And you will see the electricity that we’re producing. If I turn off the water, of course, the water wheel continues to turn for a little while, but eventually it stops turning, and then the light goes out. (scraping sound) >> I’ve been at this a long time. I’ve never seen any place that looked so good for finding neutrinos.
I just know there’s a neutrino here somewhere. Eh? Ah! Who are you? >> Excuse me, who are you? >> It’s a giant, pedantic penguin. (laughter) I’m pleased to meet you. I’m Swingwell Slugwell, explorer extraordinaire. >> What are you doing in my physics show? >> I’m doing science. >> Really? >> You’ve heard about neutrinos, right? >> Oh, yes, absolutely. >> They’re shy as sub-atomic particles go. >> That, they are. >> They never stop to say hello. If you ran into one, you wouldn’t know Oh, where, oh, where are you, little neu-trin-o? Well, all the best neutrino hunters are in Antarctica.
Neutrinos are so shy that the best place to find them is in Antarctica. >> I hear that. >> So somewhere, amidst the vast frozen wastes, I will find the legendary neutrino. Hold these. >> Okay. >> And when I find it, I’ll be famous. And then, I’ll be rich! I know it’s here somewhere. Is it this way? You know, I can feel a signal. It’s not. It’s over here.
You’re not a neutrino. >> Unh-uh. (laughter) >> I can just feel it. Yes, it’s a very strong signal. It’s right here! Yes! I have found it! Excuse me, sir, are you a neutrino? >> No! (laughter) >> Stop being ridiculous. That little boy is not the legendary neutrino. I ought to know. I am a neutrino hunter. >> A neutrino hunter? Oh? >> That’s right. >> Well, how many neutrinos have you seen? >> Today, we have seen about 646 of them.
>> 646?! Oh, it must be no ordinary neutrino hunter school you went to! >> In fact, one of the top neutrino hunter trainer programs is right here in Wisconsin. >> In Wisconsin? Well, tell me, how do you find a neutrino? >> Well, you have a neutrino hunter, such as myself, adventures deep into the frozen wastelands of Antarctica, and we dig a mile below the surface of the Antarctic ice, where we search for flashes of light, not with dousing rods, but with photo sensors, such as these. And this provides evidence of the legendary neutrino. >> I suppose you have to have a mile-long shovel to dig that mile-deep hole? >> It’s very much a challenge to dig that deep. But we use very hot water, which is environmentally safe, to dig that deep below the surface. >> You dig holes with a mile of hot water. I’d like to see the backpack that you carry your hot water in. I think I’ll stick to dousing, thank you very much. May I have my ski poles? >> Suit yourself. >> Thank you.
Cancel neutrino alert! I just know it’s got to be here somewhere. (musical flourish) (applause) >> Well, you know, they do look for neutrinos under the ice in Antarctica. They’re very tiny, very elusive particles, very hard to observe. I’ve heard of people using dousing rods to look for water. But I’ve never heard of them trying to look for neutrinos with a dousing rod. It doesn’t work for water and it doesn’t work for neutrinos, either. I can guarantee you that. But how would you get to the south pole? If you had to get there, you’d need something like a compass, wouldn’t you? It turns out, we have a compass right here. Here is a compass needle and it’s floating in the water. Now, you wouldn’t normally think a compass needle would float because it’s made out of iron, and iron is more dense than water.
Archimedes would’ve said it should just sink. But because water has a special property called surface tension, things that are sufficiently small can in fact float on the surface of the water, even if they’re more dense than the water. That’s how you sometimes see little bugs dancing around on the surface of the water. It doesn’t work for you, but it does for things that are sufficiently small. And so, you can see it’s really a compass needle. I have here a magnet. If I bring this magnet up near the compass needle, I can make it move around like that. So, it’s a way to suspend the compass needle so it is free to move with very little friction. And it will eventually swing around to point to the north, as it’s doing now. So, there’s not a lot of application of water with magnetism, but this shows you at least one way that you could use water to make a compass.
Most compasses aren’t made with water, they’re made in other ways. But you could do it that way. I want to move on and talk about the physics of light. And one of the things that relates to water– (thunder crashing) >> Professor Sprott! Professor, I’m glad I found you. Did you see the rainbow outside? >> No, I’ve been in here doing the physics show all day. >> I tried to stop her from interrupting, but she just insisted on seeing you. >> There’s no time to give a physics show! We need you to help us find the end of the rainbow! >> I tried explaining to her that there is no end of the rainbow, but she just wouldn’t listen. >> That’s because everyone says there’s an end to the rainbow. You know, with the Leprechauns and the pots of gold. >> Maybe I need to explain this.
Maybe I have something that can help. Let’s see. >> That doesn’t look like a rainbow. >> No, I don’t think that will work. Is that a projector I see up there. >> Yes, it makes a very bright light. >> Let’s pretend this projector is like the sun making a very bright light. We have a ball of water here that’s kind of like a raindrop. Let’s see if we can make a rainbow. Do you see my rainbow up there? The rainbow goes below the curtains there, just like the rainbow in the sky would go below the horizon.
So we can’t see the same thing, but it’s the same shape as the raindrop. If I turn the raindrop here, you can see the rainbow just keeps going on. They’re the same shape. >> That’s not the most impressive rainbow I’ve ever seen. Here’s a better rainbow. This is made out of a prism, a little triangular piece of glass, with light going through it. It separates the lights and all of the colors of the rainbow. >> That’s much brighter, you’re right. Are you convinced now? >> I guess I am. But I’m going to go back outside and look at the rainbow again.
>> Okay. (musical flourish) (applause) Thank you. Whoo! Thanks! If you like the rainbow, and some of these other physics demonstrations, you might want to ask your teacher to invite me to your school, since I travel all around the state of Wisconsin and do physics shows like this. You might want to hang onto this, in case it starts to rain in here. >> Okay. (musical flourish) (applause) That was Rachel Lancor. And indeed, she does travel around doing a version of this show in the schools. And if she hasn’t been to your school, you should ask your teacher to invite her some time. I don’t think it’s going to rain in here, Peter, maybe you can take this. Oh, my goodness! >> Ah-ha! That’s the pot of gold! (laughter) I’ll take that.
(musical flourish) (applause) >> Well, there goes the budget for our show next year. Anyway, we’re talking about light and water. And another thing we can talk about involves– We can use this aquarium over here to demonstrate something that you’ve probably all seen before. Have you ever taken a stick like this, which is nice and straight, and put it down under the water? And have you noticed that it looks like the stick bends? You’ve seen that, right? Let’s try it with this aquarium. You have to look down from above, or just look on the television monitor there. And you see it looks like it bends, doesn’t it? And why does it do that? Well, that’s because of a special property of water. It’s called refraction. Do you know that light travels only about three-quarters as fast in water as it does in air? It travels a little bit slower. And so, the light coming from underneath the water took longer to get here, because it was traveling more slowly. That made it look like this was bent.
And that’s an important effect that you’re all familiar with called refraction. There are other things I could show you that are related to this. Have you ever seen water go around a bend? Sorry, light go around a bend? Well, I can actually do that using a device over here. This is a very powerful laser. And you’ll see that the laser beam. Oops, get the fireman out of the way here. The laser beam is going across the room and is striking that piece of paper that Mr. Weix is holding up over there. This laser is going through a tank of water here. It’s going through a window on one side, and it’s coming out a window on the other side. What I’m going to do is open the little trap door here, and let the water stream out.
And the laser beam will be trapped inside the stream of water, and it will reflect off of the interface between the water and the air. And the light will stay trapped inside the stream of water. So let’s try that. And we have a trough down there to catch the water. (musical flourish) (applause) That’s a very important effect. It’s called total internal reflection. It’s something called a light guide, because it’s guiding the light. And you can do the same thing with fine threads of glass, with something called fiber optics. That’s a very important technology some day. I want to show you something else you can do with a laser that may surprise some of you.
I have here two Styrofoam cups. They’re empty, nothing in them. But what I’m going to do is put just a little bit of water in the two cups. First, a little bit of water in this, about a tablespoonful, and then the same amount of water in this one, about that much. And then, I’m going to take this one and I’m going to put it in the laser beam, if I can find it, right here. You see the laser beam hitting it. And I’ll just let the laser shine on it for a moment. Then I’m going to take the two cups. And this one, of course, is not in the laser beam. It still has water in it.
This one, on the other hand– (musical flourish) No water at all. Now, how many of you knew a laser would do that? A few people, but not very many. That’s good, because a laser can’t do that. This one had a sponge in the bottom. (laughter) Well, it’s a trick. But there is a point to this. There’s a point to this. When you do an experiment, it is important to have a control. One was in the laser beam, one was not. But it’s important when you have a control, that there is only one difference, the laser beam.
If one had a sponge, and one didn’t, maybe it had nothing to do with the laser beam. And that was the case here. So, it’s a lesson of how you do proper experiments, and how you really test to be sure that you don’t fool yourself into thinking that a laser will do something that in fact it will not do at all. Let me show you one other demonstration here that involves light. Here I have a beaker of water back here. And up here in front, there’s a candle. I’m going to light the candle. Then we’ll turn some of the lights down. I’m going to rotate this around. Does it look to you as if that candle is burning underneath the water? Well, in fact, it’s not.
You can’t burn a candle underneath the water. But what you’re seeing is the reflection of the candle off of this plate of glass right here. You know, you can see your reflection in a plate of glass. It doesn’t need to be a mirror. Some of the light is reflected off of the surface. And when you look at yourself in a mirror, it looks like there’s someone behind the mirror that looks just like you, that’s looking at you. But there’s really not, of course. And so, what you think you see as the candle back here, when in fact, the candle is there, in front of the mirror. And of course, you’re seeing the water through the glass right here. This is the kind of thing that magicians like to often do in order to trick you into thinking you’re seeing something that in fact you’re not.
You’ve heard the expression, “smoke and mirrors”? Well, this is a kind of a mirror. The last thing I want to show you that involves water, involves a special kind of water that you’re all familiar with. Snow. Snow is a form of water, in the form of a solid. It’s not quite like ice. It’s little tiny crystals that we call snowflakes. I want to show you some photographs of snowflakes that I found on the web. These are actual photographs taken by Professor Kenneth Libbrecht, who’s at the California Institute of Technology. He’s very famous for taking pictures like this. Have you heard that no two snowflakes are alike? Well, you know, nobody knows for sure, because no one has looked at all of them.
But Professor Libbrecht points out that probably no two grains of sand are alike, either, but nobody ever talks about that. But it certainly is true that there are lots and lots of different shapes that snowflakes can take. I wanted to show you a few of the snowflakes that Professor Libbrecht has photographed. You may notice that many of these snowflakes have a particular property of having six sides. They are so-called hexagons. And that is one of the forms that ice crystals prefer to take. So, even within the six-sidedness, though, you see an enormous variety of shapes from these snowflakes. So, the last thing I want to do for you involves another example of water. Traditionally, we always completed these shows by making a cloud. Nothing could be more relevant, because a cloud is water.
Did you know that? A cloud is very, very tiny droplets of liquid water. And how do you make a cloud? Well, how nature does it, you cool the air. And air, normally, has some water vapor in it, water in the form of a gas. And when you cool air with water vapor in it, some of the water condenses into tiny droplets of liquid water. If the droplets are too big, they fall as rain. But if they’re very tiny, they stay suspended in the air in the form of a cloud. So how do we cool the air? Well, we use liquid nitrogen. Liquid nitrogen is very cold stuff. It’s at a temperature of about 321 degrees below zero Fahrenheit, or 196 below zero Celsius. Or a mere 77 degrees above the absolute coldest anything can be.
And we have a large tank of liquid nitrogen here. What we do is force nitrogen gas into the tank. That forces some of the liquid up into this pipe, where the liquid vaporizes and forms nitrogen gas. It’s still very cold, however. The nitrogen gas, and some liquid as well, fill the room here, cooling the air, and making the moisture in the air condense, the vapor in the air condense into the tiny droplets of liquid that we call a cloud. So, with that, I will put on my hat, and I will thank you all for coming. (applause)
Search University Place Episodes
Related Stories from PBS Wisconsin's Blog

Donate to sign up. Activate and sign in to Passport. It's that easy to help PBS Wisconsin serve your community through media that educates, inspires, and entertains.
Make your membership gift today
Only for new users: Activate Passport using your code or email address
Already a member?
Look up my account
Need some help? Go to FAQ or visit PBS Passport Help
Need help accessing PBS Wisconsin anywhere?
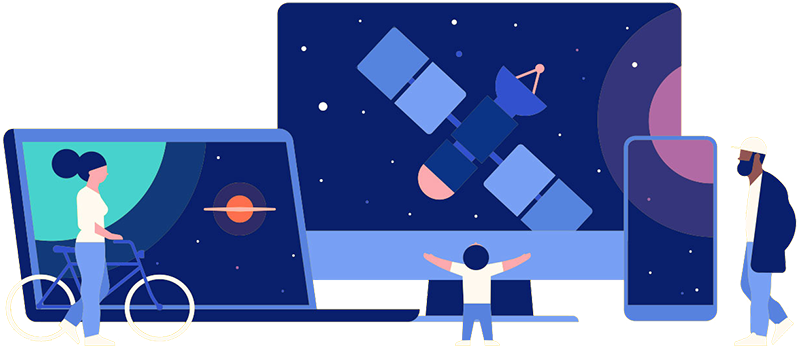
Online Access | Platform & Device Access | Cable or Satellite Access | Over-The-Air Access
Visit Access Guide
Need help accessing PBS Wisconsin anywhere?
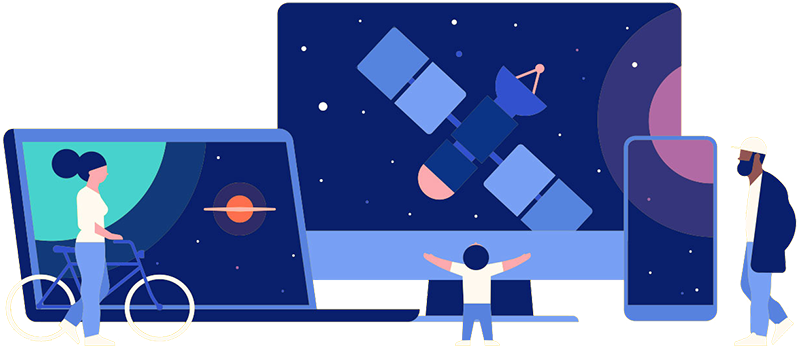
Visit Our
Live TV Access Guide
Online AccessPlatform & Device Access
Cable or Satellite Access
Over-The-Air Access
Visit Access Guide
Follow Us