[Fiona McNamara, Undergrad, University of Wisconsin-Madison]
Welcome, everyone, to Wednesday Nite @ the Lab. My name is Fiona McNamara. I’m a second-year undergrad student here at the U.W.-Madison. I work for Tom Zinnen, who couldn’t make it this evening. We do this every Wednesday night, 50 times a year here at the U.W. Biotech – Biotechnology Center.
Tonight, it is my pleasure to introduce to you Nathan Sherer from the Department of Oncology. He is originally from Bismarck, North Dakota. He went to Bismarck High School there, and then he went and got his undergrad in Grinnell College, which is in Iowa. Then he went to Yale to get his PhD, and after that he post-docked in London, England, for five years until he came to U.W.-Madison in 2011. Tonight, he will be talking about imaging live cells under viral attack. Please join me in welcoming Nathan Sherer to Wednesday Nite @ the Lab.
[applause]
[Nathan Sherer, Assistant Professor, McArdle Laboratory for Cancer Research, University of Wisconsin-Madison]
Thank you.
Thanks for being here tonight. So yeah, my name is Nate, and I’m an Assistant Professor affiliated with the Department of Oncology, which is also known for the McArdle Laboratory for Cancer Research, many of you will know.
[slide titled – Viral Videos – which is the title of Nathans talk along with his U.W.-Madison titles and associations]
And I have a joint appointment in the Institute for Molecular Virology, which is on the other side of Henry – Henry Mall in Robert Bock Laboratories on Linden Drive. And tonight –
[new slide titled – Visualizing Viruses – featuring three microscopic videos of viruses as well as a list of the objectives for the evening – Crash course on virus-host interactions and why we care; Seeing is believing – how to build fluorescent viruses and cells; New imaging-based platforms for modeling key aspects of viral replications]
– I’m going to tell you a little bit about – it’s mostly an excuse to try to – you know, as the research goes – goes on, you start to lose – lose touch with old things, and I really wanted to, you know, sort of go back through my old favorite movies and – and – and share them. And [laughs] because I – I – I miss them, and I don’t get to talk about them all the time. And – and, you know, hopefully, you know, through this I’ll be able to, you know, tell you a little bit about virology on this campus, which is extraordinary. I know Dave O’Connor here – was a couple weeks – was here a couple weeks ago talking about Zika. We have a – we have an almost second-to-none community of virologists on this campus. I’ll tell you a little bit about why we care about viruses and virus-host interactions and what we’re trying to learn. I’ll tell you about how we’ve gone about trying to build fluorescent viruses in cells that we can image using live cell imaging. So, that’s where the – the viral cellular attack and interplay comes in. And I’ll sort of finish with, you know, what I – what – what my vision for the future in terms of what we can now do in terms of sort of developing a multi-variant, high content, multi-color imaging system for trying to learn –
[Nathan Sherer, on-camera]
– very comprehensive things about how viruses act in cells, and – and how they spread from one cell to another.
Okay, so viruses, why do we care?
[slide titled – Viruses: Why Do We Care? – with the answer of – Viruses Impact the Health of Humans, Animals, and Plants]
Of course, viruses impact the health of humans, animals, and plants.
[the slide animates on eight photos – each of a different viral outbreak – one of a hospital of Chikungunya patients, one of a small child with Pox on his back, one of Japanese people wearing masks because of SARS, one of a patient suffering from West Nile, one of Middle Eastern people wearing masks around camels labelled MERS, one of a military man holding a premature baby labelled Zika, one of a man in Africa in a hazmat suit holding a child labelled, Ebola, one of two people in hazmat suits watching a dump truck discard dead chickens labelled Influenza, and one of an emaciated African man labelled AIDS]
So, this is just kind of the last five years. Things that have – many – many of these things have popped up. Some of these things you know that afflict us on a regular basis. So, we have seasonal flu, common colds, all sort of – all sorts of respiratory infections, stomach bugs, chickenpox, herpes virus-type afflictions that are latent and stick with us for – for lifetime, come back and cause shingles. Things like this. I’m going to mostly talk about HIV/AIDS cause that’s the focus of my laboratory. HIV con-continues to be a massive problem and is – is still one of the, I think, number six in terms of the top killers on the planet despite all the progress we’ve made. And then all these other guys which have been popping up in recent years as – as our – as the world population has grown, as we’ve started to commingle with new environments that – that we – that we hadn’t before, as viruses continue to change and feel out –
[Nathan Sherer, on-camera]
– new evolutionary space. It turns out that some of them start to cause human diseases, and that’s, of course, what we saw with Ebola last year, and then this year’s hot topic of Zika.
Okay, so this is going to be kind of the only dense –
[return to the – Viruses: Why Do We Care? – slide now with these global statistics – HIV/AIDS – 34 million infected, over 1 million deaths per year; Hepatitis B and C viruses – Liver disease, cancer, 200 million infected, approximately 1 million deaths per year; Herpesviruses – Ubiquitous, cause skin lesions, birth defects, cancers; Influenza A – Respiratory illness, over 250,000 deaths each year; Rabiesvirus – Almost always fatal, over 60,000 deaths each year]
– text slide. But just to run through, you know, some global perspectives on what are some of the big viruses that we worry about at present. So, HIV currently infects more than 34 million people on the planet. There have been about 7 – 70 million infected since it showed up in the late ’70s. And it still causes more than one million deaths per year even though we – we now have pretty good drugs that can control this infection. Hepatitis B and C viruses are crazy. More than 2 million – 200 million people are – are infected at any one time. And roughly one million deaths per year. They both establish or can establish lifelong chronic infections that can lead to liver disease and cancers. We’re all full of different herpes viruses. I had infection mononucleosis when I was in college and a raging case of chickenpox when I was nine caused by varicella-zoster. A variety of herpes virus circulate in humans and cause different diseases. Birth defects, cancers, all sorts of issues. Every year we’re afflicted by new versions of influenza. Roughly 250,000 deaths per year with pandemic potential. So, we’re always very concerned about flu. And rabies is always something that’s just sort of terrified me because it’s almost universally fatal.
[Nathan Sherer, on-camera]
It’s very rare in the United States to now get a rabies infection but it does happen, and it still, worldwide, leads to about 60,000 deaths per year.
And then we have these emerging viruses. So –
[return to the – Viruses: Why Do We Care? – slide now with a text box underneath the global statistics for the aforementioned viruses labelled – Emerging Viruses (a few examples) – and the following list – Ebola and Marburg (filoviruses); SARS & MERS (coronaviruses); Zika (flavivirus); Chikungunya (alphavirus). The slide also notes that with the exception of Ebola in 2016, relatively few deaths, but great concern for the future]
– each year there seems to be a hot new virus which is causing problems. You know, fortunately, with the exception of Ebola in 2016, these viruses, such as Ebola/Marburg –
–
[the slide animates on a graph titled – What will the future bring? Discovery of new viruses that infect humans – with the year from 1900 to 2020 on the x-axis and the cumulative number of virus species on the y-axis and showing a steep increase year-over-year of the number of virus species]
– which are these Filoviruses; SARS and MERS, which are members of the Coronavirus family; Zika, which is all the rage at the moment and is a Flavivirus. And Chikungunya is one that we haven’t talked about too much, but this is now prevalent in the Bahamas, and it’s also mosquito-borne like Zika. And there’s thought with climate change that this will be one that will be quite prevalent, especially in the south, over the next few years. It causes a debilitating, long-lasting disease that sort of resembles rheumatoid arthritis.
So, relatively few deaths from these emerging viruses, but they keep coming. They keep surprising us. When I was just out of college, I worked in a lab that studied Coronaviruses. The virus we studied was called murine hepatitis –
[Nathan Sherer, on-camera]
– virus. It was the only virus, the Coronavirus, that was known to cause disease. And my boss couldn’t get funding because nobody cared about the mouse. So, after I left the lab, the N.I.H. basically called her up after SARS showed up because now, we had a Coronavirus that had showed up in people and was causing disease. And they said, How much money do you need?
[laughter]
So, this is just to reinforce, you know, sort of, this need for basic research, you know? We need to study viruses even if we don’t think they’re important because we really don’t know what’s coming in the – in the pipeline. And what will the future bring?
[return to the – Viruses: Why Do We Care? – slide, described above]
This is sort of an estimate. It’s a bit dated now. This is almost 10 years old. But currently, there are at least 150 viruses that infect humans that we know about. And, you know, the prediction is, you know, over the next 10 years we’ll probably identify upwards of 50 more. And, again, as viruses fill evolutionary space and encounter people in different places and in different ways than –
[Nathan Sherer, on-camera]
– they have before, there’s always potential for a virus to find a new niche, cause a new disease.
Okay, so I’m just going to put on my ambassador hat for a couple of minutes and talk about virology on campus. So, I’m affiliated with the Department of Oncology. So, that’s cancer.
[slide titled – McArdle Laboratory for Cancer Research – 75 years of Discovery in Basic Cancer Research – featuring photos of the two buildings out of which the Lab has been based – the original building used from 1964 to 2014 and their new home in WIMR2 on 1111 Highland Avenue]
Why do we care about viruses?
[the slide animates on the sentence – Viruses Cause 15-20% Of Human Cancers! – under the title of the slide]
Well, roughly 15% to 20% of human cancers are caused by viruses. So, I named a couple of the viruses that cause cancers. So, different herpes viruses, hepatitis B and C viruses are – are tightly linked to human cancers. And the McArdle Laboratory, famous building right behind us, was – was – was really a hotbed for studying viruses that cause cancer and understanding how they worked in a variety of animal systems. And, as many of you know, McArdle has now vacated this – this – this famous building and has – has moved to WIMR 2. And – and – and – and it’s about the – it’s the 75th anniversary of the McArdle Laboratory.
[new slide titled – Landmark Discoveries – featuring the logo for the McArdle Lab and the following portrait photos of scientists along with their discoveries: Harold Rusch – Discovered that skin cancer is caused by U.V. light; James and Elizabeth Miller – Discovered how carcinogens are activated to cause DNA mutations leading to cancer; Howard Temin – Discovered reverse transcriptase, a unique enzyme in retroviruses (e.g. HIV) that is now the target of anti-AIDS therapies and winner of the 1975 Nobel Prize for Medicine; Charles Heidelberger – Discovered and patented 5-FU, a crucial anti-cancer therapeutic in use since 1957; Bill Dove – Developed the first mouse model of colorectal cancer suitable for drug testing]
And among many landmark discoveries that have been made at McArdle, such as Harold Rusch showing that U.V. light causes skin cancers; the Millers showed how carcinogens lead to cancer; Charles Heid – I’ll come back to Howard Temin – but Charles Heidelberger discovered 5-FU. This is a – a very potent anti-cancer therapeutic that’s still very commonly used in the clinic. And Bill Dove, who’s still very active in the department. He’s emeritus but continues several projects. He developed the very first mouse model colorectal cancer, suitable for drug testing. But, in terms of viruses, this all went back to Howard Temin. And this is another fortuitous basic cancer research story where Howard started his research –
[Nathan Sherer, on-camera]
– studying a chicken virus that caused a transmissible cancer called Rous sarcoma. And he was – and over time, it was found that, you know, Rous sarcoma was a – was a virus that was part of the family called retroviruses. These are viruses that are able to inject their genomes into cells, and we’ll get into the details in a minute. In 1975 –
[return to the – Landmark Discoveries – slide now with Howard Temins discovery surrounded by a red, rectangluar box]
– Temin won the Nobel Prize for discovering a reverse transcriptase, which is an enzyme. The – the retroviruses are R.N.A. viruses, they have R.N.A. genomes, but they convert that R.N.A. genome into double-stranded D.N.A. that looks just like the D.N.A. that’s inside all of our cells. And then it has a sophisticated mechanism for injecting that D.N.A. into the genome so that every single cell that gets infected inherits a copy of that virus. And Rous sarcoma virus –
[Nathan Sherer, on-camera]
– had picked up a cellular gene that it was carrying with it, which was called SAR kinase. And any time it would infect its cell, it would overexpress this kinase, and it would cause the cells to replicate more quickly. And that led to rapid cell proliferation and the development of – of sarcomas in chickens.
So, he did all of his research, and the reason that he won the Nobel Prize is because this idea of reverse transcriptase through what – what we call the central dogma of molecular biology on his head, where in the 1950s we knew about D.N.A., we knew that D.N.A. encoded messenger R.N.A.s, and we knew that messenger R.N.A.s were translated into proteins. And that’s sort of, you know, how cells worked. And, from there on, you can make all the enzymes and – and – and structures that you needed in order to make a cell and then form a tissue and then make an organism.
What Temin showed is that this R.N.A. virus – this could actually turn that mechanism on its head and take an R.N.A., turn it back into D.N.A., and then that D.N.A. could be inserted into the genome. And this – this discovery has been massively useful for biotechnology because now we can take messenger R.N.A.s that we can isolate from cells and turn them into D.N.A. copies that we can then store in the freezer and replicate and use over and over. And, perhaps most importantly, it turned out when HIV came on the scene in the – in the early ’80s –
[return to the – Landmark Discoveries – slide, described above]
– that it was – it was of the same family of viruses as that chicken virus. And it – it required the same reverse transcriptase activity. So, what Howard had taught the world about reverse transcriptase led very quickly to the design of drugs that turned out to be quite effective for inhibiting HIV. So, this discovery in a chicken virus ultimately led to, you know, saving millions upon millions of lives.
[new slide titled – Our Present and Future – and featuring the logo of the McArdle Lab and having two categories of researchers with their photos and research areas. The first is under the heading – Researchers at McArdle Laboratories continue to make ground-breaking contributions to cancer research – and featuring the photos and research of: Paul Lambert – Determining whether anti-estrogens can be used to control incidence and growth of cervical cancer; Norman Drinkwater and James Shull – Identifying common genes that control incidence of liver and breast cancer; Michael Gould – Developing rat models of breast and colorectal cancers; William Sugden and Shannon Kenney – Testing FDA-approved drugs for their impact on Epstein-Barr virus (the virus that causes mononucleosis and lymphoma). The second category of researchers photos is under the heading – New and established McArdle researchers are asking important questions still unanswered – and features these questions next to their photos – Caroline Alexander – How does cold stress impact tumor development? Wei Xu – Are there master programmers of gene expression that are good drug targets? Shigeki Miyamoto – How do chemotherapeutic drugs activate inflammatory pathways? Nathan Sherer – How does cell-to-cell transmission of HIV work? Robert Kalejta – When and how do latent viruses activate and type tissues? Elaine Alarid – How does estrogen receptor contribute to breast cancer?]
So, this is McArdle now. Temin really devoted a lot of his energy in the ’70s and ’80s to building a – a core of virologists in the department. So, our current chairman is Paul Lambert, who studies human papillomavirus that causes cervical cancer. At least in this slide we feature Bill Sugden and Shannon Kenney and Rob Kalejta and some other guy [uses mouse pointer to point to his own picture] who are in – who study – these guys study Epstein-Barr virus, which causes lymphoma and – and mononucleosis. Rob studies H.C.M.V. that causes birth defects. It’s another herpes virus and glioblastoma. We have many other prominent virologists in the department. And we interact with many fabulous other scientists studying signaling – cell signaling mechanisms involved in cancer and using genetic systems to try and determine the origins of cancer.
[new slide titled – The Institute for Molecular Virology – featuring three photos – one of the outside of the Robert M. Bock Laboratories building where the Institute is housed, one of Paul Kaesberg (1923-2010) who studied the Molecular Biology of RNA Viruses, and one of Roland Rueckert who studied the Structures of Picornaviruses. The slide also has the Mission Statement of the Lab in a red box – To conduct research and training in the area of virology, primarily at the molecular level, and to serve as the coordinating focus on the UW-Madison campus for research and training in virology]
And my other home is the Institute for Molecular Virology. So, that’s Bock that was I referring to earlier. And there we’re just all about viruses. So, this department was built by Paul Kaesberg, who passed away in 2010, but was one of the world leaders in understanding and characterizing small R.N.A. viruses, and with a prominent role from Roland Rueckert, who is one of the pioneers of understanding structural biology –
[Nathan Sherer, on-camera]
– using viral systems, with an emphasis on Picornaviruses such as polio and – and the rhinoviruses that cause the common cold.
So, at the I.M.V., we have many fabulous scientists. Our chairman is Paul Freisen, who studies –
[slide titled – The Institute for Molecular Virology – with the Institutes logo and the headshots and areas of study for these five virologists – Paul Freisen, Ph.D., Chairman IMV, Professor Dept. of Biochemistry – Baculoviruses and programmed cell death; Ann C. Palmenberg, Ph.D., Roland Rueckert Professor, Dept. of Biochemistry – Picornaviruses and the origins of respiratory diseases; Paul Ahlquist, Ph.D., Paul Kaesberg Professor, McArdle Laboratory for Cancer Research, Howard Hughes Medical Institute, Head of Virology, Morgridge Institute for Research – RNA viruses and cancer genetics; Robert F. Kalejta, Ph.D., Vice Chair, IMV, Professor and Assistant Director, McArdle Lab for Cancer Research – Human cytomegalovirus (HCMV) and herpesvirus-associated cancers; Nathan M. Sherer, Ph.D., Assistant Professor, McArdle Laboratory for Cancer Research – HIV-1 and oncogenic reverse-transcribing viruses]
– Baculovirus. Ann Palmenberg, who continues work on Picornaviruses. Paul Ahlquist, who’s one of the world’s most well renowned R.N.A. virologists. Rob and me, who – the three of us are also linked to McArdle and – and – and – and the oncology –
[new slide titled – 2016 Grandparents University, Researching Cancer Cures, featuring the logo for the Wisconsin Alumni Association and five photos of events that occurred on campus as part of Grandparents University described by Nathan below]
– department.
And I just wanted to set, you know, our mission –
[Nathan flips back two slides to the – The Institute for Molecular Virology – slide with their Mission Statement]
– is to conduct research and – and do a lot of training at the I.M.V. So, we – we want to serve as the focus for virology on the campus. We hold a weekly seminar series.
[Nathan Sherer, on-camera]
If anybody’s interested, it’s open on the – in – in the biochem building across the street at – at – at noon, all about viruses. And we – we – we play a big role. Every five years we host the American Society for Virology meeting in Madison at the Monona Terrace. And a couple weeks ago, we hosted a Grandparents University, both out at McArdle –
[return to the – 2016 Grandparents University – slide with the five photos from the event]
– and at the I.M.V. Here’s Ann Palmenberg teaching alumni and their grandkids about viral structures. Kelly Waters, a post doc in the department teaching them about sequences. So, just an advertisement, if you’re a grandparent, an alum, a grandparent, or – or –
[new slide titled – Want to Learn More Viruses at UW-Madison? – featuring the logos and website information for the Institute for Molecular Virology and their websites – virusworld and the Madison Virus Program, as well as the web information for the McArdle Lab and the Carbone Cancer Center]
– know of one, that should be pretty fun. And I’ll show this again at the end of the talk, but these are just if you’re interested in virology on campus. We also have fantastic virologists in the department in the Veterinary School, the Department of Medical Microbiology, Immunology and also in the –
[Nathan Sherer, on-camera]
– pathology and laboratory medicine.
Okay, so H.I.V./A.I.D.S. I mentioned more than 35 million people infected globally. We still have –
[slide titled – HIV/AIDS – featuring a world map with a color-coded key showing the prevalence of HIV/AIDS in each country of the world. The largest numbers are in the United States, India, and Southeastern Africa. In a box the slide also has the following list – Approximately 35 million infected globally, 2 million new cases per year with approximately 1 million deaths; No vaccine – 1 PERSON CURED OUT OF 70 MILLION; Combination antiretroviral therapy (ART) can be highly effective – As access to therapy improves, a reduction in spread]
– two million new cases per year and more than one million deaths. Despite huge efforts, we still do not have a vaccine for H.I.V. We have one person out of 70 million infected over the history of this – this pandemic who we think is cured, who has been off drugs for several years with no re-recurrence. That cure involved a stem cell transplant and some very clever gene therapy type considerations. So, it’s not a practical cure for anybody else.
We have great drugs since the mid-’90s that keep people alive and – and to have a happy and healthy life. But the problem remains getting everybody on drugs, getting everybody adherent to their drug regiments, keeping the drugs cheap enough and accessible. So, it’s still going to be an enormous task to eradicate. The current goals of the – the C.D.C., the N.I.H., and the W.H.O. and the U.N.A.I.D.S. are this 90-90-90 idea –
[Nathan Sherer, on-camera]
– where 90% of the people who are infected with H.I.V. are going to know that they’re infected. It means more and more people have to be tested. 90% of those people who are positive are going to have access to drugs and are going to stay on those drugs. And of those 90% who are on drugs, they’re going to have to have their viral load suppressed. If that works, the math looks really good that we could have an A.I.D.S.-free generation over one to two generations. So, that’s the current goal for between 20 and 20 – 2020 and 2030.
So, I’ll point out that, you know, typically these days we think about sub-Saharan Africa.
[return to the – HIV/AIDS – slide with the world map of cases with a red arrow pointing to the Southeast coast of Africa – Sub-Saharan Africa]
This is where the – most of the cases of H.I.V. are and most people are – are – are dealing with H.I.V./A.I.D.S.
[the slide animates on a new red arrow that points at the United States]
But I’ll also point out that, you know, we don’t talk about it much anymore, but we still have huge numbers of people who are H.I.V. positive in the United States. Well over a million, and the number continues to grow. And it – and – and its one of these problems that is particularly bad for under – underrepresented minority communities that have limited access to healthcare.
[new slide titled – What makes a Virus a Virus?]
Okay –
[the slide animates on the following facts – VIRUSES ARE REPLICATING NANOPARTICLES – Average size 30 – 300 nm diameter; Virus – Latin for poison, first used in reference to rabies in the 1890s; Transferable, non-filterable disease agent – smaller than bacteria; Obligate intracellular parasites – viruses encode their own genes, but are completely dependent on cells to replicate]
So, now the crash course in viruses. What makes a virus a virus? So, effectively, the simplest way to think about viruses is that they make things called virions, which are replicating nanoparticles. So – so, at the nano scale, about 30 to 300 nanometers is kind of a – a common size of – of – of the virions that we study and think about. Virus came from the Latin for poison. It was first used in reference to rabies infection in the 19th century. Around that time, it was – it – it was found that you could filter out bacteria from – from animal – animals who had things like rabies or – or this chicken sarcoma virus that Howard Temin studied. And you could still transmit serum and transmit the – the disease, which meant that there was something smaller than bacteria which we –
[Nathan Sherer, on-camera]
– which we couldn’t access, which was capable of causing disease. Sort of a terrifying concept at the time.
And then, I’m a cell biologist or I like to think of myself as a cell biologist, so the – the coolest thing about viruses is that they’re obligate –
[return to the – What Makes a Virus a Virus? – slide]
– intracellular parasites. They’re not capable of replicating on their own. They have to find a cell in order to replicate. They all have their own genomes and their own genes. They make their own proteins and their own enzymes. But they – they need cells, or their – or they – or they can’t do it.
[new slide titled – The Virion: A Single Unit of a Viral Transmission – featuring a cross-section illustration of a virion of the Human Papillomavirus which is circular in shape and has Capsid proteins on their outer edges and then an inner octagonal shaped area made up of Genomic DNA that connect to larger areas called Histones. The bottom of the slide has a box with the info on the key bits of a virion – Nucleic acid (RNA or DNA) which is their genome and a protein shell which is the outer capsid]
So, this is the – the transmissible unit of a virus which is known as a virion. So, even though in the newspaper they’ll show a picture of this, and they’ll call it a virus, remember to always correct – correct them and say that’s a virion. The – the – the core of a virion is there’s a genome so it’s nucleic acid. So, it’s either D.N.A. or R.N.A. It can be double-stranded or single-stranded depending on the virus. There’s every permutation on the planet because there’s so many viruses on the planet. They all have different ways of operating. This is the human papillomavirus that causes cervical cancer, and other cancers can also, different versions of it can cause warts on your skin. And so, papillomavirus has a D.N.A. genome that looks quite similar to the genome in our cells that’s even wrapped up on these things called histones. And it’s packed in a – in a – in a – in a capsid core, which is – which is shown here, and that protects the genome. And the idea here is that this is Uber of the virus. The virion can take the infection from one cell to another.
[new slide titled – To Understand the Virus, Understand the Cell – with the statement that – Viruses cannot move, generate energy or synthesize proteins outside of the cell. The slide features an illustration of a virion approaching a cross-section of an Animal Cell which at its center has a Nucleolus which is in the center of the Nucleus which is surrounded by the Nucleopore and Rough Endoplasmic Reticulum that contain Ribosomes. Additionally, outside the Rough Endoplasmic Reticulum is the Smooth Endoplasmic Reticulum, Microtubules, Centrioles, Golgi Bodies, Lysosomes, Mitochondrions, and Cytoplasms]
So, if you want to understand the virus, you have to understand the cell. And all viruses evolve to understand cells better than we ever could, which makes them really good tools for research. So, the virion needs to get inside a cell. So, this is a depiction of an animal cell. You see the nucleus here where the D.N.A. is stored. All the organelles in the cytoplasm that are there for metabolism and processing proteins and making things work. So, again, this concept where the viruses can’t move, generate energy, synthesize proteins outside the cell.
There is an age-old debate over whether viruses are alive or not –
[Nathan Sherer, on-camera]
– because if this guy is on its own, it can’t replicate. It doesn’t have everything that it needs. In the context of a cell, some people argue that a virus is alive, but, you know, you kind of end up with this – this metaphysical concept of what is – if – if it’s really a – a – a living organism or some other kind of organism.
So, what we’ve learned, especially with new sequencing technologies, is that there are an insane number of –
[return to the – To Understand the Virus, Understand the Cell – slide, described above]
– viruses on the planet. So, every virus needs a cell, and the world is full of cells. So, we’re made of cells. Plants are made of cells. The soil is full of cells. The ocean is – is massively full of cells. It’s just teeming with bacteria and other – and other microbes. And every one of those is potentially a host for a different type of virus. So, it’s now predicted that there are at least 100 million different viruses on the planet. Most infect bacteria in the ocean, and a recent realization is that these infections of bacteria are actually involved in a huge amount of nutrient turnover on the planet. So, it’ll probably play very important roles in terms of – of maintaining the – the viable ecosystem for the beautiful planet that we live on.
In mammals, there are at least 320,000 different viruses currently predicted. And I mentioned this earlier, we know that there’s at least 150 viruses that infect humans. Not all of them cause diseases.
[Nathan Sherer, on-camera]
Some of them might actually help prevent diseases. So, it’s a – it – its a complicated world when it comes to viruses.
And this is – I saw Jean-Yves walk in, who is – Jean-Yves Sgro who has worked for the I.M.V. in the Biotech Center and has done all these amazing illustrations of different viruses –
[slide titled – Virions Come in Many Shapes and Sizes – and featuring 29 illustrations of various virus capsids to show the wide variety of virus structures with credit to Jean-Yves Sgro and the website – www.virology.wisc.edu/Virusworld ]
– capsids and different virus structures, which are accessible at this great website called Virus World. So, if you get a chance, check it out. These are some examples of the beautiful structures that viruses come in. Many of them are icosahedral in structure and spherical. Many of them end up making what we call nucleo – spherical or filamentous nucleocapsids, which are linear.
[the slide superimposes the sentence – and can also have spikes, tails or be coated in lipids (envelopes). Additionally, the slide superimposes the spherical shaped HIV virus and the worm shaped Ebolavirus cross-sections and notes that HIV and Ebolavirus have lipid envelopes but very different capsid/nucleocapsid structures]
There’s every sort of shape and size that you can imagine. And then on top of that, beyond the protein shell and the genome, you can get all these accessories. So, some of the viruses have tails and spikes. Many of the viruses that we’re most concerned about, Influenza, Ebolavirus, H.I.V., they have a lipid envelop. So, they steal some lipid from the surface of the cell and surround themselves. They add an extra layer of protection on top of the protein that –
[Nathan Sherer, on-camera]
– that protects their genome. So, here’s H.I.V. It has a beautiful protein conical core that coats –
[return to the – Virions Come in Many Shapes and Sizes – slide with the cross-sections of the HIV virus and the Ebolavirus]
– two R.N.A. genomes on the inside. And then that’s surrounded by a lipid. And then this is Ebola, which has this long filamentous nucleocapsid surrounding a – an R.N.A. genome, which is – but it’s also wrapped in a – in a lipid. So, again, a huge amount of diversity in different evolutionary strategies –
[new slide titled – A Typical Viral Life Cycle]
– that viruses have adopted to do what they do.
[the slide animates on an Ebolavirus Life Cycle which is a cross-section of a cell that becomes infected with the Ebolavirus. Steps 1 – 4 are delivery steps – Entry to the cell, Macropinosome, Late Endosome-Lysosome, and Trigger; Step 5 is Replication; Step 6 is Transcription; Step 7 is Translation]
Okay, so what happens in the cell? So, I’m going to use Ebola as an example. This is an image from a relatively recent review on Ebola replication. And basically –
[the slide animates on the following information – Virions have very small genomes (4-250kb of nucleic acid) compared to cells (3 time 10 to the seventh power kb in human genome). Despite this, they must encode enough information to initiate and complete the infectious cycle: 1) Delivery – attachment and cell entry, 2) Decoding – viral mRNAs are translated to proteins by cellular machineries, 3) Replication – Genomes are copied by viral or cell polymerases, 4) Release – of assembled particles that encapsidate the genome (virions)]
– what needs to happen, viruses, they have relatively small genomes since they’re much smaller than cells. So, they have enough to replicate themselves, but everything else has to come from the cell. The first step is delivery. So, for Ebola, these first four steps over here are delivery. It’s going to bind to a cell. It’s going to be taken up into the cell, and it’s going to find a way to get into the cytoplasm where it can access all the enzymes that it needs to replicate. Two is decoding, where the viral mR.N.A.s – so, the genes in the virus make messenger R.N.A.s that are made into viral proteins to make new viruses. And then, number three is replication. So, here we see protein synthesis and things starting to come together. The genomes are being replicated down here, step five. And then, finally, everything has to come back together in kind of this symmetrical process, make a new virus, or new virion, sorry, and then move on to the next cell.
[the slide animates in the following information in a box at the bottom of the slide – All viruses must find a suitable host cell; All viruses must evolve a strategy to move from host to host]
So – so, viruses are very specific in terms of the cells that they can infect. They – theyve adapted to certain cells. That’s why we don’t – your dog might get a –
[Nathan Sherer, on-camera]
– canine parvovirus, but it won’t be able to infect you because you don’t have the right cells for that virus. And all viruses must evolve a strategy to move from – from host to host. Some use blood products. Some will – some prefer sneezes and door handles. And – and – and – and – and then, within the tissues, they have to have ways that they can circulate in order to make enough copies to move on to the next person.
Okay. And then, I really like this other point that a lot of viruses really ravish their cells.
[slide titled – Many viruses ravish their host cells; Some, like HIV, need to be more nurturing – featuring three illustrations of how viruses attach host cells. The first, labelled a shows a dsRNA virus that enters the host cell and performs plus RNA synthesis that extracts the plus RNA which through translation and packaging enters new cells and performs minus RNA synthesis to form a new virion. The second shows a plus RNA virus that enters the cell and extracts the plus RNA and some through translation creates new virion proteins while the rest goes through template recruitment translation where replication proteins are created that go through minus RNA synthesis and then plus RNA synthesis which removes the plus RNA which in turn combines with the virion proteins to create a new virion. The third illustration, labelled c, is of a Retrovirus. The virion enters the cell and performs reverse transcription which causes DNA integration through transcription at which point the plus RNA is removed and through translation and packaging form the new virion]
So, they – theyre – theyre kind of smash and burn. They go into the cell, they replicate, they get out, and they trash the place. And a lot of double-stranded R.N.A. viruses do this and also positive-strand R.N.A. viruses. And I make this point because for H.I.V. that’s not really the case. H.I.V., because it integrates its D.N.A. into the genome, it really wants to keep the cell happy because it’s going to be its home for a while. And this is especially true, this is why we can’t get rid of H.I.V. because once you’re infected with the H.I.V., it inserts its genome –
[Nathan Sherer, on-camera]
– into the cell forever, and it hides out in those cells so that even if you’re on drugs, if you ever go off drugs, there’s going to be a few cells that are latently infected or persistently infected, and then the virus will come raging back.
So – so, in – in terms of Madison real estate, its kind of – some – some –
[the slide superimposes two photos – one over the dsRNA and plus RNA illustrations of a scene from the movie Animal House and a second photo over the Retrovirus of a young woman with a sponge and spray bottle tidying her kitchen]
– people are more invested in their homes than others. So, the – so – so, we have these – these smash and burn type – type viruses and the others that are very committed to keeping the cell happy and – and healthy.
[new slide titled – Seeing is Believing]
And then that has consequences for the pathogenesis.
Okay, so seeing is believing.
[new slide titled – How Do We Visualize Something So Small? – featuring three illustrations in a rectangular box. The first is of a cell which is portrayed as a small white square in a larger blue rectangle, and which has a measurement at the bottom as 10 microns. The second illustration is labelled – bacterium – which is shown as a very small purple rectangle, and with a measurement of 1 micron. The last is labelled – virus – which is shown as a tiny blue dot, and which has a measurement of 100 nanometers]
How do we see the viruses? So, if you look at your hand, there’s a bunch of cells there –
[Nathan Sherer, on-camera]
– but you can’t really see them. You can see them, but they’re all stitched together. You can’t see an individual cell unless you have a microscope; it’s too small. So, an average cell is probably about 10 microns –
[return to the – How Do We Visualize Something So Small? – slide with the illustrated cell, bacterium, and virus]
– in diameter. Bacterium is about tenfold smaller than that. About a micron. And then a – a typical virus is about 10 times smaller than that. So, we can’t see individual cells in our hands, in our saliva, anywhere. Kind of the – the exception would be eggs, which are really big cells. But the- bact – we can’t see most bacterium unless we have a microscope. And then viruses are – are a severe challenge beyond that.
[new slide titled – Electron Microscopy – featuring a photo and four microscopic images. The photo is of the large electron microscope on campus; the first microscopic image is of the T2 Bacteriophage showing a size of 120 nanometers; the second image is of several Tobacco mosaic viruses with a scale of 300 nanometers for each; the third image is of several round HIV viruses and showing a scale of 150 nanometers; the final image is a combined image labelled – Modern Cryo-Electron Microscopy plus Tomography at a single nanometer scale]
So, how do we deal with that?
Traditionally, the way that we’ve studied viruses, especially up until the 1980s, was using electron microscopy, where the idea is we had technology, and some of this was actually developed by Paul Kaesberg in the – in the I.M.V., where we could take viruses, or what we thought were viruses, and put them on to a very thin grid, coat the grid with metal, and then bombard the sample with electrons. And whatever light got through could then be detected, or whatever electrons got through could be detected, and then leave an image of – of what’s there. So, that’s – thats how the viruses were originally detected and characterized using the electron microscope, and you could get resolution data 120 nanometers.
This is the T2 Bacteriophage, which is a famous virus of bacteria. You can see it looks like a lunar lander module. So, the – the genomes up in this –
[new slide titled – Time Lapse Light Video Microscopy – featuring an image of an electron microscope hooked up to a laptop]
– capsid shell on top – I hit the button.
[return to the previous – Electron Microscopy – slide with the microscopic images]
And then there’s this long syringe-like extension or tail, and then it’s got these little – these little landing pads that it uses to latch onto the bacteria and inject its nucleic acid. It’s almost designed like a syringe. This is tobacco mosaic virus, one of the most famous plant viruses – early viral model systems. And actually, makes long helical capsids. The capsids that are about 300 nanometers. This is one of our images of H.I.V. coming out of a cell. So, this is a – this is a human cell making envelope H.I.V. particles. You can see the tiny little conical capsid in the interior and the lipid envelop on the outside. And then, recently, in the last 10 to 15 years, we’ve made huge advances using a – a – a technique called Cryo-Electron Microscopy with Tomography, or Cryo-EM.
And the idea here is that you can make a bunch – one, you can –
[Nathan Sherer, on-camera]
– freeze the samples instead of using harsh fixatives, and you can maintain very good structure of viruses. So, you can maintain the lipids and the proteins, keep them really happy. And then you slice them up very carefully, put them on an E.M. grid, and – and – and you take a picture of each one of the individual – so, it’s like slicing up an apple. You take a picture of each individual slice, and you – you turn it slowly in the beam so that you get a whole bunch of different images from different angles of that apple slice. And then you can computationally put them all back together and actually get a three-dimensional image of – of what’s there. And this has gotten so –
[return to the – Electron Microscopy – slide with the four images]
– good now that the – the detectors can now achieve single nanometer scale in – in – with some virus particles. So, some of the structures would be down to, you know, 2.6 angstroms which is – used to be – all we could – the only way we could get that kind of resolution is with crystal structures.
[return to the slide labelled – Time Lapse Light Video Microscopy – with the microscope connected to the laptop which he mistakenly went to previously]
So, it’s an exciting time for E.M., and it continues to advance even though it’s a very old-school technique.
Alright, so, in the ’80s, people started coupling fast cameras with microscopes and moved into the age of time lapse video microscopy. And this is my favorite thing.
[the slide animates on a photo of a sick child in the lower left corner and the right-hand side now has a large, microscopic video of Rhinovirus A (common cold) which is subtitled – Human HeLa cells over approximately 15 hours with Kelly Waters and Ann Palmenberg]
So, we finally get to a viral video.
So, this – this is a movie that – that I did with Kelly Waters and Ann Palmenberg, who study human rhinovirus A in this case, which causes the common cold. And the idea here is that each one of these individual blips is a cell. So, these are HeLa cells from Henrietta Lacks that –
[the slide animates off the sick child and the Rhinovirus A movie and replaces them with a photo of two caterpillars (one normal and one destroyed by a virus) and a large, microscopic movie of Baculovirus which is subtitled – SF21 Insect cells over 24 hours – Gulam Manji and Paul Friesen]
– we use quite frequently for our research.
[return to the previous – Time Lapse Light Video Microscopy – slide with the Rhinovirus A (common cold) movie, described above]
And we’re going to infect them with the rhinovirus and see what happens.
[hits play on the Rhinovirus A movie]
And so, this is about 15 hours of imaging. And what you can see is the cells are not very happy. So, this is a smash and burn virus. This is a – this is a virus that does not care about itself for very long. It just wants to make copies of itself, make the cell explode, and release new copies of the variant. So, we can’t see the variants. They’re too small, but we can see what happens to the cells. And now you can imagine this is what’s going on in your lungs every single time that you have a cold.
[return to the – Time Lapse Light Video Microscopy – slide with the Baculovirus movie, described above]
So, not a very nice virus.
And then I really love this movie. This is from Paul Friesen who studies Baculovirus. This is an insect virus. I’m glad it doesn’t infect humans because what it does is it basically – it causes caterpillars to melt. It – its – its really ferocious. Its replication cycle is that it will actually reprogram –
[Nathan Sherer, on-camera]
– the caterpillar to climb to the top of a tree. It will make the caterpillar melt, and then it’ll drip down onto the bottom leaves and then other caterpillars will eat it. And that’s how it moves from one caterpillar to the next. It’s absolutely insane. So –
[return to the previous slide, described above]
– these are insect cells taken from drosophila, fruit flies, and they’re infected with Baculovirus. And this is –
[presses play on the Baculovirus movie]
– I think, yeah, 24 hours of imaging. And you can see what the Baculovirus does to the cells, which is extraordinary. So, the cells are moving around. You can kind of see a big sphere in the middle. That’s the nucleus. And they start to bleb. You know, that’s a sign that cells are getting sick, and they’re thinking about dying. And then we’re about 12 hours now.
[accidentally advances to a new slide titled – The HIV-1 Life Cycle Is Not So Disruptive – featuring an illustration of the HIV-1 lifecycle]
Whoops.
[return to the – Time Lapse Light Video Microscopy – slide with the Baculovirus movie, which he restarts]
Oh, man. Now I’ve got to restart the whole thing. I can cut to chase. And the virus starts cranking out protein like crazy. And Baculovirus actually turn out to be a wonderful tool for making protein for biotechnology purposes because – cause it completely hijacks cells, uses all the resources in the cell to do its own bidding. And – and what you see here are huge – these – these – these are called occlusions of viral capsid proteins in the interior. They make these – these big and remarkable cubes in the interior of the cell. And you can see at the end of the movie, you know, the cell looks nothing like it did at the beginning, and it’s a – its a – its a pretty drastic transition.
[return to the – The HIV-1 Life Cycle Is Not So Disruptive – slide featuring an illustration of the life cycle of HIV-1 below it]
Okay, so H.I.V., as I mentioned, is not so disruptive. So, you don’t learn much from studying H.I.V. just with a light microscope. You’ve got to have a trick in order to put a label into the virus so you can actually see it. So, I’ve kind of shown you this earlier, but the – the – the basic 30-second life cycle of H.I.V. is that it’s a virus that has two copies of an R.N.A. genome, has this lipid envelope, it recognizes a receptor on the surface of select cell types, and releases the capsid into the cell. This is Howard –
[new slide titled – Fluorescence Microscopy – featuring a photo of a Florescent Microscope and four illustrations of how it came to be. The first image is of a blueish Jellyfish which then has an arrow pointing to an illustration of the Jellyfishs Green Fluorescent Protein which then has an arrow pointing to an illustrated Engineered Fluorescent Virus which then has an arrow pointing to a microscopic image of Fluorescent Viruses in Cells]
– Temin’s discovery is that the –
[return to the – The HIV-1 Life Cycle Is Not So Disruptive – slide, described above]
– R.N.A. genome, which is red, gets turned into blue D.N.A., shown here, and then that gets taken up into the nucleus and stuck into our genome. And then after that it really pretends to be a cellular gene. So, this is a really clever virus. It – it just acts like all the rest of our genes. It tells – it uses our enzymes to make messenger R.N.A.s that are then taken out into the cytoplasm to make new proteins, and then those proteins make new virions, and they go on to infect other cells.
Okay, so how do we study –
[return to the – Fluorescence Microscopy – slide, described above]
– H.I.V.? And here the – the key breakthrough was in the ’90s where –
[Nathan Sherer, on-camera]
– it was identified by – by a few different groups that – that these jellyfish and other organisms in the ocean had fluorescent tendencies. And a few of the groups were able to isolate the genes that made them fluoresce. And the most famous one is this one called green fluorescent protein.
[return to the – Fluorescence Microscopy – slide]
Most of the work on this was done by Roger Tsien, who won the Nobel Prize with two other groups for this discovery. And – and their clever idea was, Well, if we could isolate this fluorescent protein, we could attach it to whatever we want, and then we could put it under a microscope and we could do just what the jellyfish does, is we could – we could – we could use that fluorescent protein to detect light and then emit a different wavelength, and – which is the essence of fluorescence –
[Nathan Sherer, on-camera]
– and we could track that protein in the interior of the cells. And this would open up all of cell biology because now we could actually see what’s going on inside a cell, not just watching cells die. So, when I was in grad school, we started engineering fluorescent viruses.
[return to the – Fluorescence Microscopy – slide]
There were a few other groups that were doing this as well, but I think we were the best.
[laughter]
But the – but the idea here was if we could make that capsid, so that cone in the center of H.I.V. or other retroviruses, green, for example, and use other fluorescent proteins. So, by the time that I was in grad school, there was a whole rainbow of different fluorescent proteins that could be used, so you could actually make two- or three-color viruses. We could make a – make a fluorescent envelope. Then we could actually look inside cells, and we could see how the viruses came out of the cells, how they went into the cells, and how they moved from one –
[Nathan Sherer, on-camera]
– cell to another. So, that’s what we set out to do.
And this work was done with Walther Mothes at – at Yale University when I – when I was –
[new slide titled – Rainbow Retroviruses: HIV-1 and Murine Leukemia Virus (MLV) – and featuring a small headshot photo of Walther Mothes, and an illustration of how the red and green fluorescent proteins are extracted from the Jellyfish DNA – noted as Gag and GagPol – and with the proteins labelled GreenFP and RedFP. The slide also note that the Green Gag-GFP works with HIV, HTLV, ALV, etc., and that red psuedotyping allows for Gag/Env mixing and matching. The virions also retain high infectivity]
– a graduate student. So, we engineered murine leukemia virus, which is a virus very similar to H.I.V. It has a very similar lifestyle. It – it’s also a retrovirus. We did the same thing for H.I.V., but most of our emphasis was on M.L.V. It was a bit safer and easier to work with as a model. And – and we fused the capsid protein, which is called gag, which makes this – this shell in the center of the retrovirus to green fluorescent protein. And then there’s a glycoprotein that the virus makes that sticks on the outside. So, it’s the spike protein. We made that red. We found a place to insert the fluorescent protein that wouldn’t screw up the virus.
[new slide titled – Virions Surfing on Filopodia – featuring a small headshot photo of Maik Lehmann and then a photo and four microscopic images. The first is of a fluorescent microscope video of MLV entering a host cell – the host cell is green and the MLV is red. The second is an electron microscopic image of the same cell and MLV and showing red arrows for the flow of the virus and noting that the viruses do not fuse at filopodia. The image is a photo of a surfer riding a wave next to two electron microscopic images of MLV, one in which the cell is not receptive (with arrows pointing to a gap between the virus and the cell) and one in which a cell is receptive (with arrows pointing to the virus just before entering the cell). It is noted that the surfing of the virus is receptor dependent]
And then, actually –
[return to the – Rainbow Retroviruses: HIV-1 and Murine Leukemia Virus (MLV) – slide, described above]
– in – in the back – you know, so we could spin down those – those virions that were secreted from cells and make big tubes full of these fluorescent viruses that we could then – that we could then throw on cells.
[return to the – Virions Surfing on Filopodia – slide, described above]
So, this is work from a post doc in Walther’s lab, Maik Lehmann. One of the early cool things that we found was this phenomenon called virus surfing. Where this is an uninfected cell [using mouse pointer to indicate the video] which is green. So, it’s fluorescent green so you can see it. And it turns out, when you start looking closely at fluorescent cells, they’re covered in these actin-rich protrusions. So, you know, actin is the –
[Nathan Sherer, on-camera]
– the protein that runs our muscles, but it’s also used in cells to guide a lot of motility in different functions. So, you see these long fibers sticking out. Those –
[return to the – Virions Surfing on Filopodia – slide and the fluorescent microscope video]
– are called filopodia, and one of the things that we characterize – was when you added virions to cells, they would bind to these actin-rich filopodia and move inward. And we can show by electron microscopy that the virions are actually on the outside of the filopodia. So, they were actually surfing along the outer surface. That’s why –
[new slide titled – Virion Surfing Precedes Entry – featuring a fluorescent microscope video of green MLV virions entering red endosomes (Clathrin). Additionally, there is an illustrated cross-section of a virion entering a cell wall with the virion as a red dot that is approaching a yellow cell wall with actin on the outside; the illustration shows a green squiggly line that shows the entrance of the virion into the cell with a question mark near the actin that says – signaling? At the bottom of the slide is this bulleted list – 1) Virion Env-receptor binding (MLV, HIV, ALV, and VSV-G); 2) Linkage to underlying actin; 3) Lateral trafficking; 4) Endocytosis or fusion at the cell body]
– we call it surfing.
And then – and then, you know, kind of our overarching model for why the – the viruses did this with several additional studies was that they would use these filopodia to get in towards – Retroviruses want to get their genomes toward the nucleus – so, this allowed them to directionally move from the outer space towards the inner space. And we can see them co-localizing with these things called endosomes, which are vesicles that take things from the surface of the cell into the interior in order to direct things inward.
[new slide titled – Visualizing Retroviral Assembly – featuring an illustration of the assembly and budding of retrovirus particles – showing small virions attaching to the outside of the cell and those parts of the cell that are receptive to the virions letting them in to form what look like bubbles of 3 to 4 virions in the cell. Next to the illustration is a fluorescent microscope time lapse movie of dark green MLV dots entering a cell where one frame equals 20 seconds at a scale of 10 microns]
So, I never published this, but – but this was one of my achievements where I think, you know, I was – I was – I was probably the first person to every – to ever see a retrovirus actually budding and forming in real-time. So, these are individual virus particles forming on the surface of a cell. You can see the cell moving and kicking around. The green signal is the gag protein, and every now and then it all coalesces together to form a little dot. And you can see an example of that here. And then you can see there’s an example of some other virions stuck on the surface. And there’s one, when it loops around again, about here that pinches off and floats away to go infect another cell.
So – so, anyway –
[new slide titled – Cell-To-Cell Transmission: There are Multiple Routes! – featuring an illustration of two cells – one is a red rounded rectangular infected cell with a smaller blue rounded rectangle as its nucleus. This cell has two half circles with small Ts sticking out of them representing virions in the cell. The second cell is a blue rounded rectangular cell with a smaller blue rounded rectangle as its nucleus. This cell has small Ys all around it representing actin]
– this was a useful tool for sort of starting to get –
[the slide animates a red circular virion with Ts all around it breaking away from the red infected cell and heading towards the blue healthy cell]
– understand how fast –
[return to the – Visualizing Retroviral Assembly – with the fluorescent time lapse movie, described above]
– viruses –
[Nathan Sherer, on-camera]
– were made, how they got out of the cell, where it happened. It was pretty clear from these types of movies that it was happening at the surface plasma membrane of the cell. At the time that wasn’t known. And how they get into the interior of the cells.
And the other one that we were very interested in is – how do viruses –
[return to the – Cell-To-Cell Transmission – slide with the red circular virion out of the infected cell and heading towards the blue healthy cell – now with the title – Cell-Free Mode animated in]
– move from cell to cell? –
[the slide keeps animating red circular virions out of the red infected rectangular cell]
So, you know, you guys are comfortable with the virion idea now, and it turns out there’s multiple ways that virions can move from one cell to another. There’s what we call the cell-free mode, which, you know, with an H.I.V. infection probably happens in the blood stream. There’s lots of little virions that are moving around and circulating –
[the slide animates the first red circular virion approaching the second blue cell, finding a receptive actin, and entering the blue cell which in turn becomes red to show that the second cell is now infected]
– and looking for new host cells. So, they can float through space and then infect a new cell.
[new slide titled – Cell-To-Cell Transmission – Cell-Cell Mode – which features an illustration of a red rectangular infected cell with a smaller blue rectangular nucleus in it and having little Ys all around its surface representing actins]
But then there’s also this –
[the slide animates on a new blue non-infected rectangular cell with a smaller blue nucleus. It too has actin Ys around its surface. The two cells are animated as bouncing around the same space]
– notion that in dense tissues, if you have a cell that’s infected with a virus, such as H.I.V., and it encounters a cell that’s uninfected –
[the slide animates the cells stopping bouncing around and instead meeting up at the same x-axis and also animates the Y actins interacting with one another and commingling to create a viral linkage or viral synapse]
– the two cells can actually interact to form intimate linkages, which we call virological synapses.
[the slide then animates circular red virions moving out of the red infected cell and moving into the blue healthy cell through the linkage to in turn infect the healthy cell which turns from blue to red]
And at these sites of contact is very efficient for infection to move from one cell to another.
[new slide titled – Retroviruses Spreading From Cell To Cell – featuring a fluorescent microscope time lapse movie which has two green cells – one on the right which is infected with MLV virions that are red, and one healthy cell on the left which is just green]
So, we set up some systems to try to visualize this in real-time. [presses play on the movie] And here’s my favorite movie ever. [The movies clearly shows red MLV virions entering into the healthy cell] So, what you see here is an uninfected cell. Here’s an infected cell. It’s making little red murine leukemia virus dots. And when the two cells interact with each other, they grab onto each other. You see the little dots form at the site of contact. And then they go running over from one cell to another. So, this entire movie is about 30 minutes, but it almost looks like a machine gun. You know, the virions are being shot over to the uninfected cell.
[new slide with a new fluorescent microscopic movie showing a three cells – one in green in the middle which is infected with darker green MLV dots, and two healthy cells in red on either side of the infected cell]
And then this one is kind of dramatic. It’s a bit bright up here. I don’t know if I can fix the – the light, but –
[Biotech Center worker, off-camera]
Uh, no.
[Nathan Sherer, off-camera]
I didn’t have any instructions. [laughs]
[the slide animates on the cover image from the DVD for the movie Cover over the top of the movie of the cells]
But anyway –
[the DVD cover of Cover animates off leaving the three-cell time lapse fluorescent microscope movie]
– this was, in terms of folk stories, the a – this – this was a nice – this is a nice movie because it shows the – I lost my cursor. Oh well. It – it shows the – it shows an infected cell making green viruses in the center with two uninfected cells in either direction sort of pumping viruses in both directions. And if you’re looking for –
[once again, the DVD cover for the movie Cover animates on the slide over the top of the time-lapse fluorescent microscope movie]
– oh, here’s my cursor. Okay. If you’re looking for a good date movie, there were actually – a bunch of our movies were used as the intro to this – this – this movie about H.I.V. in the African American community. It’s a murder mystery by Bill Duke in the intro credits. So, that was one of my most exciting achievements. I have a – I have a –
[laughter]
[Nathan Sherer, on-camera]
– I’m in the movie database with the credits. So, check out Cover, directed by Bill Duke.
Okay, so now we’re working on, since we’ve come to Madison, when I did my post doc, I didn’t do any imaging, or very little, you know? And I – I learned all sorts of other techniques. But I worked a lot on R.N.A. trafficking and H.I.V. gene expression, and everything was very focused on H.I.V. So, when I got here, the thought was that technology has progressed to the point where we now have microscopes where we can – you know, a lot of those movies I showed you were half an hour, two hours – now we can keep cells alive for days, and we have automated stages where we can look at 24 different conditions for three or four days. We can look at multiple rounds of infections. We can look at how the cells are interacting. We can look at six different colors because of all these different fluorescent proteins. And we can use the computer to start to make these very elaborate models for what’s going on.
So, I’m getting late so I’m going to go through this relatively quickly.
[new slide titled – HIV-1 Genomic RNAs (gRNA): A Vertically Integrated System for Studying mRNA Trafficking, Translation, and Protein Function – featuring an illustration of a cell infected with HIV and the following list under the title – Key Players – gRNA – Unspliced genomic RNA; Encodes Rev Response Element (RRE); mRNA for Gag/Gag-Pol – Rev – Binds RRE; Recruits CRM1 – CRM1 – Transports gRNA to cytoplasm – Gag – Assembles particles; Packages gRNA]
But one of the systems that we set up was we wanted to understand H.I.V. gene expression, how we make a virus particle, and kind of the overarching goal of this is that we don’t have any drugs that shut off H.I.V. virion production. All the drugs that we have in the clinic kill the virions after they’ve been made. So, if we could figure out ways to shut off making new virus particles, that would be useful. And we know a lot about how this works but – but not enough. Still a lot of questions.
So, we set up this system where we could see H.I.V. genomes in the nucleus using one color. So, we can make green R.N.A.s that are going to be exported from the nucleus into the cytoplasm. They’re going to encode a blue gag protein. I brought this one up earlier. That’s the protein that makes the capsid shell that makes the virion. So, this is a budding virion at the surface of the cell. And then we’re going to use this – this red protein that I haven’t introduced yet, which is called rev, which is an activator of – it turns on gene expression for the virus. So, we had a system where, if we put in the red protein, that would take the green genome out of the nucleus. That would make the blue protein, and then the whole virion would get made at the cell surface. So, we wondered if we could – we could model this using three colors.
[the slide animates off the list and replaces it with a time-lapse fluorescent microscope movie of an infected HIV cell with the Gag in blue, the gRNA in green, and the Rev in red. It is noted that the actual time is 22 hours in a single HeLa cell]
So, we made this virus, which we called three-colored visible H.I.V., and then this is what it looked like in the cell when we could finally figure out a way to image for 24 hours without killing the cell. [presses play on the movie] In order to do that, you need cells that are very cooperative and that don’t move and very hardy and – and don’t mind being blasted with light for long periods of time. And again, HeLa cells are – are – are very good for this.
So, what you see in this movie is that, at the beginning, we’re starting to see the red protein, and that’s the protein that’s turning on gene expression. The green signal that you’re seeing in the center circle is individual genomes inside the nucleus. And then, over time, that green signal is going to move into the cytoplasm, and that’s where translation occurs, and proteins are made. So, we’re seeing nuclear export of messenger R.N.A.s and translation. And then, at the end of it, we start seeing bright blue and green dots, which are new virions being made at the cell surface. So, it worked great. We could see the entire –
[Nathan Sherer, on-camera]
– all the explosive events that occurred during H.I.V. virion assembly. And – and – and – and – and, really, we’re visualizing the central dogma. We’re seeing R.N.A.s being made. We’re seeing them exported from the nucleus. We’re seeing translated to make protein. And then we’re seeing the proteins do something, which, in this case, is make a virion.
And we’ve kind of set up this systems-based approach –
[new slide titled – HIV-1 Virion Production: A Day in the Life – featuring another time-lapse fluorescent microscope movie on the left and the an illustration of the virion making process on the right]
– where we can do these movies on thousands of cells simultaneously, and then we ask the computer to start to extract information from the movies. And this is work led by Ginger Pocock, with help from Jordan Becker in the lab.
[the slide animates on three graphs of data on the bottom of the slide, for Rev, RRE, and Gag]
And using advanced image analysis, we can then extract all sorts of intimate details about what’s going on in each single cell and then use that to build a model for how we think that virus assembly and virus particle production works. So, I –
[the graphs and the movie animate off the slide and the Day-in-the-Life illustration grows bigger with text boxes showing all the parts of virion production from transcription to translation to nuclear export to assembly to packaging. At the bottom of the slide is a text box titled – Systems-based framework for dissecting HIVs productive phase – and the following list – Dynamics and molecular mechanism; High content screening platforms; Novel image analysis tools (pattern recognition)]
– moved quickly through this, but this has allowed us to build a spatial-temporal model for –
[Nathan Sherer, on-camera]
– all these different aspects. So, between zero to 15 hours, we see genomes being made in the nucleus.
[return to the previous slide, described above]
They get exported to the cytoplasm. We can measure very carefully the rates of gag synthesis. And then we can – we can see –
[the slide animates on a time-lapse fluorescent microscope movie of the Gag-CFP]
– in this movie, we – we can see when virus particles start to be made very specifically.
[the movie animates off]
And – and now we’re using this for a lot of different things. We’re learning, using it to study mechanistic aspects of the virus. So, what cellular proteins and what viral proteins make this whole thing work and how can we perturb that in a productive way. We want to set this up as a high content screening platform where we could, you know, use drugs and not only know if they’re blocking H.I.V. replication but specifically at what stage are they blocking the pathway.
And we have great collaborators on campus to develop new image analysis tools cause this is really the future where we can use –
[Nathan Sherer, on-camera]
– imaging to extract as much information as possible from movies, effectively, and – and tell us lots of things. And these are just some – some additional fun –
[new slide with two time-lapse fluorescent movies – one titled – Identifying Cellular Proteins That Regulate HIV-1 where the HIV-1 RNA is shown in green, the HIV Rev protein is shown in red and an invisible additive Sam68 is also present – and the other titled – Identifying New RNA Trafficking Behaviors where Viral RNA is shown in green, the Cells DNA is in blue, and the Cells Microtubules are shown in red]
– viral videos. So, in terms of understanding the regulation of this export pathway, what you see here are H.I.V. R.N.A.s. We’ve been testing different cellular factors, which – which we think might be involved in the gene expression pathway. And when I turn this on, you’ll see the H.I.V. red protein come up in rev, and that’s the protein that turns on gene expression. But we’ve also put in a protein that you can see that’s called Sam68, and when we do that, it makes everything basically explode out of the nucleus. So – so, we’ve accentuated this – this – this – this spatial nuclear export activity that nobody’s ever seen before. And I’m very excited about this because it implies that – you know, we always thought that – that mR.N.A.s in the nucleus just trickle out of the nucleus – out of the pores – but here they all move into a big focus.
We’ve also been able to use this system to identify new activities. So, what you see here are two cells that are actively dividing – a cell that’s dividing into two daughter cells. So, the red signal here is microtubules, which form the spindles that take the chromosomes into the two new cells. And the green signal is the viral R.N.A., and we found a specific viral element that – that clumps R.N.A.s very specifically onto microtubules. And we honestly don’t have any functional relevance for this, but it’s a pretty movie so we’re –
[laughter]
– were – were trying to understand why – why would R.N.A.s want to go to – to the end of micro – microtubules in these sub-organelles called the – called centrosomes.
[new slide titled – Towards a Comprehensive Model of HIV-1 Spreading Infection – featuring an illustration of an HIV-1 infection from the Lymph nodes to the surface of the skin]
And then the other thing that we’re working on that I’ll – that Ill – that Ill finish with is the – the most important thing is understanding how H.I.V. spreads in tissues and how it establishes long-term persistent infection. So, you know, H – H – H.I.V. is very good at hiding in – in – in dendritic cells and – and tricking immune cells to pass the virus on from one to the other.
[the slide animates off the illustration and replaces it with a time-lapse fluorescent video showing a collection of cells – HIV infected cells that are red and uninfected cells which are green]
So, we’ve been trying to make these – we’ve been trying to take a step back and set up systems where we can make scenarios that look like tissues and then mix cells together that are infected and uninfected and use fluorescent markers in order to track the virus moving over time. So, what you see here, the red cells are infected with H.I.V. and the green cells are uninfected. And they’re moving, and – and we’ve developed software where we can track how the cells inter – interact, when they become infected, all these different attributes of the infection system. And then, hopefully, we can develop this into better systems for finding ways to actually block-
[new slide titled – Molecular Regulation of Virological Synapses – featuring another illustration of the cell-cell method of infection at the top of the slide and below the illustration two time-lapse fluorescent microscopic videos showing this type of infection]
– the spread of infection.
[the slide animates off the viral videos and replaces them with illustrations of how the process works as well as a graph showing a graph of some of the experiment data]
This is just to show that we can measure stuff. [laughs]
[laughter]
[new slide showing a detailed illustration of the steps involved in the cell-cell variation of virion infections]
And by measuring these things, by making it quantitative, we can – we can come up with new models for how we think – how we think the system works. So, the quantification and the –
[Nathan Sherer, on-camera]
– computational aspects are very important. And so, in terms of cell-cell spread, our initial studies have allowed us to develop this model for how we think remodeling of the infected cell in red allows it to basically grab on to –
[return to the previous slide, described above]
– uninfected cells and – and then make them do their bidding.
[slide titled – Summary – featuring the following bulleted list – UW-Madison is an amazing place to study viruses; HIV/AIDS remains a huge global and local problem; Live cell imaging is a powerful tool for studying virus-host interactions; New single cell imaging platforms will pave the way for discovery, drug screens, and clinical diagnostics]
So, I’ll summarize and just say that, you know, U.W.-Madison is a fantastic place to study viruses. If you’re into viruses, this is the place to be. H.I.V./A.I.D.S. remains a huge global and local problem. And – and hopefully I’ve convinced you that live cell imaging is both fun and – and sometimes informative. We’ve learned quite a bit about how viruses work and how cells work. And – and some of these discoveries have been relevant to thinking about new therapeutic strategies. And – and, again, I think in the future, combining live cell imaging and different fluorescent imaging-based platforms is going to be tremendously useful with – with – with – with new statistics and new computational models for setting up these model systems, for understanding how viruses work, the next generation of drug screenings and even new clinical diagnostic tools.
[new slide titled – Acknowledgements – featuring a list of contributors to Nathans work as well as a group photo of the folks who work at McArdle Labs]
And finally, the most important part is the – the people who did the work. So, in my lab, I showed work from Jordan and Ginger, who’ve done the – Jordan’s done a lot setting up the – the visual system. Ginger is our – our image analysis guru. Jay Gardner is working on the cell-cell transmission system. And I’m grateful to everybody else in the lab. We have fantastic collaborators on campus doing a lot of different viruses and imaging projects. I especially emphasized –
[Nathan Sherer, on-camera]
– Paul Ahlquist and the Morgridge Institute for their investment in virology and helping young faculty on campus. These guys, Walther and Maik, were my mentors who provided a lot of the ideas. And then the people that make the – the research go. So, we have funding from the N.I.H., the – the Shaw Scientist Program from the Greater Milwaukee Foundation the Wisconsin Partnership Program, and we just got money from W.A.R.F., UW2020, to buy a new microscope. So, we’re incredibly grateful for all those contributions. And I’ll put this slide back up on there. Make sure you “like” McArdle Laboratory for Cancer Research on Facebook.
[laughter]
And I’m very happy to take any questions.
[applause]
Search University Place Episodes
Related Stories from PBS Wisconsin's Blog

Donate to sign up. Activate and sign in to Passport. It's that easy to help PBS Wisconsin serve your community through media that educates, inspires, and entertains.
Make your membership gift today
Only for new users: Activate Passport using your code or email address
Already a member?
Look up my account
Need some help? Go to FAQ or visit PBS Passport Help
Need help accessing PBS Wisconsin anywhere?
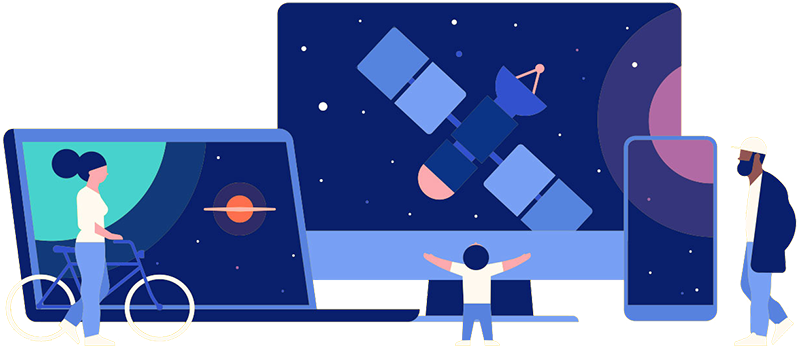
Online Access | Platform & Device Access | Cable or Satellite Access | Over-The-Air Access
Visit Access Guide
Need help accessing PBS Wisconsin anywhere?
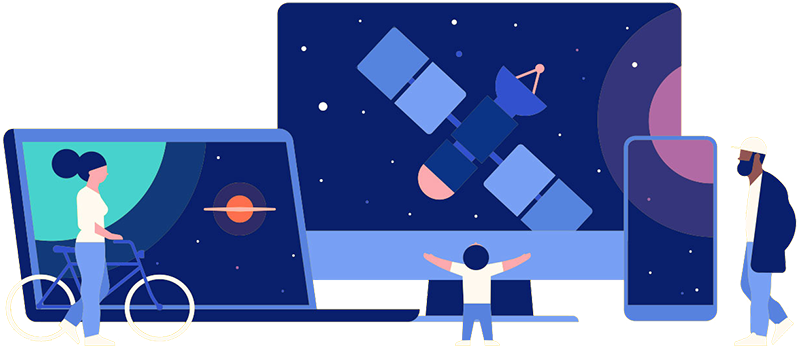
Visit Our
Live TV Access Guide
Online AccessPlatform & Device Access
Cable or Satellite Access
Over-The-Air Access
Visit Access Guide
Follow Us