[cheerful music]
– Pete Weix: Are you ready to see some physics?
– Audience: Yes!
– All right! Well, now I’m going to read you a story.
– Children: No!
– Yes. [audience laughing] This is The Physics of Time.
– Woman: Ew, dust.
– Once upon a time, in the dark and dusty basement of the physics building at the University of Wisconsin, an intrepid scientist was hard at work in his lab. One night, he dozed off and he began dreaming about the many problems facing society and how the physics of time might help us solve some of these problems. Who, you may ask? Why of course, it’s our very own Professor Clint Sprott! [cheerful music] [audience applauding and cheering]
– Welcome to The Wonders of Physics. Now, I have been dreaming about the many problems facing society: climate change, the environment, energy, transportation, cybercrime, and it occurred to me, physics has a lot to say about these things, especially the physics of time. If there were just a way to slow down time, we could solve these problems at our leisure. I’m sure my crack team of physicists could make that happen.
– Yes, the world is facing great problems, but many people believe that we have plenty of time, and if we do nothing, everything will be just fine. And the idea of slowing down time is simply ridiculous. Meanwhile, Professor Sprott’s mind was racing, but he dreamed of going slow at the pace of a tortoise. [Clint snoring] [“Happy Trails”]
– Tortoise: Why, hello everybody. I sure like this idea of slowing down time. Then it’ll go at my pace. Now, before we can slow down time, we’re gonna have to be able to measure time. But even before we measure time, we can already learn a lot about physics by thinking about when things happen at the same time, or when something happens first and something else happens last. Now I’m famous because of a race. So let me be in charge of a few races right now, and we can see this in action.
First, I’m gonna prop up this lever arm with a stick, such that when I remove the stick, it’ll start to fall. And I can put a ball on this tee right on the edge. And we’re gonna see who wins in a race between the ball and the lever arm. If the ball falls faster, it’ll end up stuck under the lever arm. If it falls at the same speed, it’ll just kind of ride its way down. But if the lever arm goes faster, the ball will end up in the cup. Oh boy, for this to work, I have to be fast, snatching that stick away. Well, here goes. [lever arm bangs] [triumphant music] [audience applauding] Now let’s see that in slow motion.
– Child: Mommy! [audience gasping] [audience applauding]
– Now for our next race, we’ve got two different paths. They both go the same horizontal distance, but one drops twice as far, I’m gonna start two balls at the same height at the same time, and let’s see who wins the race back to me. Ah, the red ball won, but could it be a trick ball? Let’s swap the tracks and see. Again, ready, set. And again, the lower track’s ball came back first. That’s because in dropping further, it gained more energy and was traveling faster for more of the distance. So it made the trip faster and won the race. [triumphant music] [audience applauding] Now, I’m famous for one particular race. Can you shout out what I did to win?
– Audience: Slow and steady.
– Oh, do you wanna hear how I really won? [audience chuckling]
– Audience Member: Yeah.
– Well, up there is the hare who has graciously said he would come back to reenact things. And I’ve got this ball launcher perfectly aligned, aiming at him, way up there. But at the start of the race, he’s gonna start to fall and I’m gonna launch the ball straight at him, and let’s see what happens. Ready, set. [machine bangs] [triumphant music] [audience applauding] Let’s see that again in slow motion. [machine bangs] [audience gasping] [audience applauding] Yeah, I knocked him off the course. So slow and steady and knowing some physics helped me win that race. Let’s understand that physics in a slightly different situation. Here, I’ve got two balls. They’re gonna fall at the same time. This one’s gonna fall straight down, but because of this spring, this other one’s gonna get launched horizontally.
And we’re gonna see if they fall at the same time, and we only hear one clack when they hit the floor, or if one of these balls is gonna land first and then we’d hear two separate clacks. So keep your ears peeled as you watch it. Ready, set. [machine clicks] [balls clacking] [triumphant music] [audience applauding] Ah, now that was pretty fast. Let’s see that one in slow motion. [machine clicks] [balls clacking] So they land at the same time. Only gravity is acting on these balls vertically. And so even though one is being launched horizontally, it takes the same amount of time to land on the ground. And that’s the same thing that was happening when I aimed the ball up at the rabbit. Because the rabbit dropped with gravity and the ball was launched up, but gravity was pulling it down.
And that’s why I hit the rabbit much lower than where I aimed at him. But that’s not what was happening with this lever arm because I tricked you. The lever arm wasn’t just falling, it was also rotating because there’s a hinge. And in doing that rotation, the very end of it had to flick faster so that the whole thing could rotate around and the lever arm fell faster than gravity. And that’s why the ball was slower. Well, that’s enough races for today. Let’s actually work on some things that are supposed to get in sync with each other. Here I’ve got a collection of metronomes, and we’re going to start them out of sync with each other. And they’re sitting on this board that when I remove these blocks, will be able to wiggle. And we’re gonna see them get into sync with each other because they’ll actually share their momentum and energy with each other.
I’m really proud of this shellection of metronomes we’ve got. I do hope that this demo isn’t a turtle disaster, like it wasn’t the last show. [audience chuckling] Oh, it’s almost tortoise something useful. [audience chuckling] [metronomes clicking] I think we can have a shellebration now. [audience applauding] Now if I stop it briefly, they’ll get back out of sync because they can no longer share energy. You can see that’s already started to happen with some of these. Because they can’t wiggle anymore, they can’t share their energy. Now a metronome is really like an upside-down pendulum. And pendulums are also really wonderful for physics demonstrations. And the thing that matters for how long a period of a pendulum is, how long it takes to go from its starting point back to its starting point, is how long the string of the pendulum is.
So here’s a set of pendulums with different string lengths, and we’re gonna watch and see what happens when we displace them the same distance and release them all at the same time. And you can watch here or up on the screen behind me. Ready, set.
– Child: Wow! [audience murmuring] [triumphant music]
– And they come back into sync. [audience applauding] Now, these patterns of different periods getting in sync with each other is particularly important this year, 2020, a leap year. Because we have to get the two periods of a year, the amount of time it takes the Earth to go in orbit around the Sun, and the period of a day, the amount of time it takes Earth to turn around its axis, to sync up, because a year isn’t an integer number of days, and we have to add leap days to make up for that. Now, pendulums are great for demonstrations, but they’re even more useful. Because they have a regular period, they can be used to tell time. So here I’ve got a really long pendulum string, that I can put a softball on. And I’m gonna have you guys help me figure out what its period it is.
So I’m gonna say “Ready, set. ” And then we’re just gonna count one one thousand, two one thousand, and see how long the period of our pendulum is, okay? Ready, set. One one thousand, two one thousand, three one thousand. So here it is back. But you know what? Softball isn’t very exciting. Let’s use a bowling ball for our pendulum now. I’m gonna pull it back the same distance. And we’re gonna do the same thing, okay? Ready, set. One one thousand, two one thousand, three one thousand. So it had the same period, even though it’s a much larger and much heavier ball.
But what if we increase the displacement?
– Child: Oh, gosh!
– Okay, is everybody ready?
– Child: Yeah!
– Ready, set. One one thousand, two one thousand, three one thousand.
– It’s the same period. So this feature of pendulums is why they’re so good at keeping time. No matter how you start them, it’s just their length that matters. And that’s why they’re still used in clocks, like your grandfather clocks that you might see. Well, unfortunately for Professor Sprott, I haven’t been able to slow down time. But we do have a way to measure it now, which is really useful, ’cause I’m gonna be late for pizza lunch with my cousins. You know, Leonardo, Raphael, Donatello, and Michelangelo. [“Happy Trails”] [audience applauding]
– Yes, we can use time, and use it to predict motion of falling bodies. But it was Sir Isaac Newton who wrote down the equations of motion that we use even today. However, there was a strange thing about his equations. They worked just as well backward in time as they did forward. Wouldn’t it be nice if we could just turn the clock back and undo our mistakes?
– Woman: Yes.
– Alas, some things in physics suggest that that’s not possible. And then it began, night sweats, a nightmare!
– Clint: No, no, not backward. Let’s go forward, onward, but always winding down. What does it mean? I’m so hot, burning up. Must get colder, seek Lord Kelvin for help. [Just-in huffs] [audience applauding]
– Greetings, we are but colleagues of Lord Kelvin. Just-in and Tim at everyone’s service.
– Lord Kelvin is master of all things thermodynamics. Hot and cold, order and disorder. It seems like Professor Sprott was in a rough spot. It looks like we have arrived, Just-in Tim.
– No, no, no, it’s “just in time. ”
– Ah, well, I like Tim more. And speaking of order and disorder, hot and cold, speeding things up and slowing down, everything involves time, and that includes thermodynamics.
– Indeed, and we have many ways to show that.
– Ah, to demonstrate, our favorite card game, 52 pickup.
– It is a perfect example of order and disorder.
– Yes, and here’s how it works. A deck of 52 cards in a neat and ordered state. But now. . . [blower whooshing]
– And voil, now disordered. And that hardly took any time. Maybe if we wait a bit and run the clock backwards, the cards will reorder themselves and go back in Tim. [clock ticking]
– Come on.
– Maybe not. That was an example of an irreversible process, and we can’t run the clock backwards. But some things look disordered and they actually aren’t. Unlike quantum physics, Newton’s laws, as you just heard, can be made to go backwards.
– Ah, an example of this is our reverse mixing demonstration.
– Just-in: And you can see Tim is taking the time to draw a pair of vertical colored lines in this liquid-filled cylinder.
– Yes, and I can mix these lines by turning this crank up over here. Now we’ll do it slowly together from one to three, ready?
– Tim and Audience: One, two, and three.
– Just-in: Oh, they’re all mixed up. I know I am.
– Ah, but let’s see if we can run the clock backwards and go back in time. So I will turn the crank now the opposite way from three down to one, ready?
– Tim and Audience: Three, two, and one. Whoa. [triumphant music] [audience applauding] Oh, that’s weird. The lines seems to have reappeared and looks like we went back in time. But no tricks here. Glycerin, which is much thicker than water, preserves its path taken. Reminds me of the breadcrumb trail in my favorite old fairy tale. I think it was called “Justin and Tim. ”
– Huh, try “Hansel and Gretel. ”
– Oh, right.
– And what about hot and cold? You may ask, how do they relate to Tim?
– Ah, you keep saying Tim instead of time. It’s driving me Just-in sane. I wish you would just leave me and my relatives out of this and say time.
– Fine, fine. Hot things move more quickly and expand, while cold things move more slowly and shrink. And for that, you need to measure the changes with time.
– Well, let’s not shortchange our audience and show them some examples.
– Sure, how about this? Look over here. I bet I can get this balloon to fit inside this glass container.
– Oh, well, so can I. That’s pretty easy. [balloon popping] [triumphant music] [audience laughing and applauding] Thank you, thank you, oh!
– Hey, that’s cheating, Tim.
– Tim: That’s cheating, Tim? All right, let’s see you do it, Justin.
– I can and I will. I just happen to have another balloon over here, and with liquid nitrogen, I will slow the gas molecules inside. But because the air pressure on the outside of the balloon is a constant one atmosphere, the balloon will shrink. That will happen when I take this Dewar, which is filled with liquid nitrogen at a cool -321 Fahrenheit, and pour it on top of the balloon, cooling it down. It’s shrinking! Oh, very nice. Inside you go.
Almost done, there, we got it. [audience laughing] [triumphant music] [audience applauding] Ah, and you know, with respect to just the balloon, this is actually a reversible process because all I have to do is warm the balloon up and it will recover its original shape. [audience applauding] [triumphant music]
– All right Just-in, impressive, sure, but shrink, shrank, shrunk, right? What does this have to do with time at all?
– Well, cold things move more slowly, so everything takes more time. In fact, there’s a special temperature, absolute zero in degrees Kelvin, at which all thermal motion stops. So it will take forever to do anything.
– Things taking forever to do anything? Sounds like somebody I know, old man.
– [groans] Very funny.
– I’m getting a bit of a brain freeze over all of this. So how about we just try speeding some things up?
– Oh, that sounds like a good plan. How about some warmed up gas molecules?
– Oh sure, but can we see warmed up gas molecules?
– Oh, it’s easy. Seeing is believing. All it takes is a little smoke and mirrors. Now for this demonstration, we need a light source and a camera. And to get the light from the light source to the camera, we have a mirror placed here. But you’ll notice in front of the mirror, we have a hot air gun. So when I turn it on, it will produce some warm air. That warm air will be a little less dense than the air around it, so it will rise and pass in front of the mirror. That warm air will also bend the light from the light source differently. And you will be able to see it on the screens on my left and my right. Very clearly the evidence of the warm air. Let me turn it on.
– Tim: Oh!
– Just-in: Do you see that?
– Tim: That’s warm air that we’re seeing?
– Yep, very easily. Now, it’s called the Schlieren effect. But people see this all the time in summer when they’re driving. If the road is really hot and you look in the distance, you’ll see the shimmering. Well, they don’t call it the Schlieren effect, they call it a mirage, but it’s the same thing.
– Hmm. [triumphant music] [audience applauding] Okay, that’s interesting, sure. But I do remember you mentioning something about smoke and mirrors and I see a mirror, sure. However, Just-in, no smoke.
– Oh yes, no worries, we got that.
– Ah.
– It also works with a simple match. I’ll just light the match. There’s the smoke. And then I’ll hold the match in front of the mirror. And again, you’ll see the Schlieren effect. [audience murmuring]
– Ah.
– Isn’t that lovely? [triumphant music] [audience applauding]
– All right Just-in, I think I like where this is heading. I wonder if we have any other ways to demonstrate hot air moving fast.
– Oh, of course. How about Tim, some hot gas bubbles?
– Hot, hot gas bubbles? Wait no, no, no. I’ve heard of this one before, and Lord Kelvin have told me that he does not like this demo.
– Oh pah, Lord Kelvin can complain all he wants to from his current location, which is far, far away.
– [laughs] I mean I guess he’s not here and he doesn’t have to know. And kids love bubbles, I guess.
– I know I love bubbles. I’ll just turn on this gas and you can see it bubbling in this glass beaker here, and out through the top. Pretty soon you’ll see some lovely bubbles. We’ll slow it down just a bit. Now these are filled with gas called methane or in your house, natural gas. And it’s used to heat your houses, and it’s also used for cooking. Here we have a lovely snake.
– Tim: Ooh.
– Because methane is a little less dense than air, so it floats very nicely.
– So yeah, I see the bubbles really nicely, but I’m almost scared to ask. You said something about hot air?
– Oh, that’s what this candle is for.
– Tim: All right.
– Hot air, faster air molecules, and a very irreversible process. Parents, don’t let your children try this at home. Come on, you. [Tim laughs] Awesome. [audience oohing] [triumphant music] [audience applauding] Now we’ve got to turn off the gas. There we go. Oh, that was interesting.
– Well, that was pretty cool, I’ll say it myself.
– I think that was pretty warm! [Tim laughs] Hot even. To be honest
– Well, I think we should probably see it again, huh?
– What do you think?
– Audience: Yeah!
– Tim: Yes, okay.
– Let’s see if we can get those bubbles to be a little less dense and rise up a little better. There we go.
Let’s see, the snake should come up. There it goes, it’s coming up very nicely. Let’s turn it up a little faster so that we get a nice
– Oh, this one feels like it’s gonna be a big one, huh? Well, we’ll see how long it goes. Sometimes the snakes get away from me, but I usually manage to catch it before it floats too far. I think that’s long enough. Okay. [triumphant music] [audience oohing] [audience applauding] [air blowing]
– All right, all right, that was pretty neat. So I wonder if we have any other ways to demonstrate how hot air moves fast?
– Well, I do if you like coffee with creamer.
– Oh, well I like cream, sure, but I’m not so fond of coffee creamer itself.
– Oh, so that’s how you feel? Even better, come with me.
I’ll show you a fun way to get rid of it.
– Okay.
– Okay, come here, Tim. So, some of you in the audience may have noticed that we have some coffee creamer here in this beaker, and it’s actually inside that funnel already. And I’ll just turn on the burner, because that will provide a light source, the heat source. Is it burning, yes. And Tim will simulate a dust explosion when he gives a really good blow on that tool.
– Oh, all right, I don’t know what you’re planning, but here I go. [air whooshing] [triumphant music]
– Woo! [audience applauding]
– Now, we can also slow time down in the slow motion right here.
– Child: Ooh.
– Child: Whoa, kaboom!
– Justin: Mm-hmm, very nice. Now, perhaps a good hot cup of coffee will calm Professor Sprott down– without creamer– when he finally wakes up.
– Tim: Oh, I think I hear Lord Kelvin calling us. We should probably head back to the lab.
– Oh, oh dear, we’ve gotta run. See you later.
– Bye bye. [triumphant music] [audience applauding]
– Another day and another story. So having dreamed of the tortoise, Professor Sprott began to dream of a hare. [audience laughing] [brass music] What’s up, Doc
– Drill Instructor: One, two, three, four!
– Bugs Bunny: What’s up, Doc?
– The Hare: I’m late, I’m late.
[The Hare huffing] [audience applauding] I don’t think I’m ever gonna catch up. Did you notice it go by?
– Woman: No.
– What, you didn’t notice my voice go by? I’ve been chasing it all day. Well, maybe it wasn’t a loud enough sound for you to notice. Hm, I have an idea. Right over here I have a hydrogen balloon. These are loud, this’ll get your attention. You might want to cover your ears. Ready? Okay, now see if you can notice the sound when it goes by, okay? Okay, in five, four, three, two, one. [balloon pops] [The Hare laughs] [triumphant music] [audience applauding] Do we see it again in slow motion?
– Children: Yeah.
[balloon pops] [The Hare laughs] [audience laughing] I bet you noticed that sound go by!
– Man: Yeah.
– Man: Oh yeah.
– Yeah, but that, but how fast did it go? Does anybody know what the speed of sound is? You know what, let’s find out. Because as you’ve already learned, anything involving speed involves time. So speed is how far something goes divided by how long it takes to get there. Hear that clicking sound? [speaker clicks] That is coming from my speaker right over there. And it’s showing up on my oscilloscope. Where’s my oscilloscope? There it is, showing up on my oscilloscope is this yellow spike. And over on the other side of the room, I have my Lightning McQueen umbrella. [audience chuckles]
– Woman: Woo!
– Yeah, well today it’s not an umbrella, it’s a collector. It’s focusing those sound clicks onto a microphone over there. And those sound clicks are showing up on my oscilloscope as this blue squiggle. And I notice that the yellow spike and the blue squiggle show up at different places on the screen. That difference is time. The time it takes for sound to go from the speaker to the microphone. Now, I happen to know that that distance is 15 meters, so we’ve got that part of our equation. Now we just need to know how long did it take? Well, according to my oscilloscope, it took 44 milliseconds to get there, or 44 thousandths of a second. So, I take my calculator and I would take 15 and I divide that by 44 thousandths of a second, it gives me about 340 meters per second. That’s about 767 miles per hour. Or put another way, that’s the length of three football fields in one second, including the end zones. Do you think you can run that fast?
– Children: Yes!
– No, I don’t think so, I don’t think so. So now we know that, oh, wait a minute. He hates this clicking sound, let me turn this off. [switch clicks] [switch clicks on] Hey, just kidding. [switch clicks off] Hey, for my next demonstration, I’m gonna need a helper from the audience. Someone who’s not been up here yet.
– Boy: Me!
– Right there, you sir, yep, come on down. Right this way. All right, what’s your name?
– Henry.
– Henry, you get to hold onto this for a minute. Just hold it up there nice and high. What we have here is a wood whistle and four balloons, each with a different gas in it. Now, note that we hear that comes out of this is also depends on time. The time it takes for sound waves to bounce back and forth inside the whistle, which in turn determines how many sound waves come out of the whistle every second. Now scientists call that frequency, and they measure that in waves per second or hertz. So what’s with the balloons? Well, the speed of sound depends on a lot of things, including what the sound wave is going through. So for this first balloon, it’s filled with air. So, Henry, I want you to just squeeze that together. I’ll hold this. Let’s see what it sounds like with air coming through.
[mid range tone whistling] Okay, that’s what it sounds like with air. Now, this next balloon has got helium. Now, helium’s a very light gas. What do you think’s gonna happen? Let’s find out, let’s open it up. [high range tone whistling] Open it nice and wide. Oops, it’s getting a little twisted there. [high range tone whistling] Wait a minute, keep going, keep opening up. [high range tone whistling] We represent the lollipop guild, the lollipop guild [audience laughing] But notice how much higher that pitch is, right? That’s because helium molecules are much lighter than air molecules. So it means at a given temperature, they move much faster. Well, faster-moving molecules mean sound waves move much faster than the gas, which means more sound waves out per second, which means a higher pitch or higher frequency. Now this next balloon has got carbon dioxide. You think it’s lighter or heavier than air?
– Audience: Heavier.
– Heavier? Let’s listen carefully. [low range tone whistling] Yeah, I think that sounds a little bit lower-pitched. Carbon dioxide molecules are a little bit heavier than air, so that means that sound moves through them more slowly. So it means we get less waves per second, a lower pitch. Okay, close that up. Now, speaking of heavy gases, is this last one here has got sulfur hexafluoride, which is a really heavy gas. Just take that clip all the way off. Just take it all the way off.
[lower range tone whistling] Those sulfur hexafluoride molecules are very heavy compared to air, which means they move very slowly compared to air. So you get fewer waves per second out and a lower pitch. That was great. Let’s give him a big round of applause. Thank you so much. [audience applauding] [triumphant music]
– Snuggle Bunny: Hey, where are you? You know I can’t start dinner until you bring those carrots home! Quit dawdling, I’m hungry!
– Oh my gosh, I’m late for dinner! Coming my little Snuggle Bunny, coming! [triumphant music] [audience applauding]
– Yes, sound travels very fast, but some things seem to happen immediately. When I flip this switch, the light comes on right away. But even electricity takes time to act. And in his sleep, Professor Sprott was dreaming about the speed of electricity and its many consequences. [electricity buzzing]
– Hello everyone, I’m Professor Stein. First name, Frank. [audience applauding] And what do you think my middle initial is?
– Boy: Frankenstein?
– Not N but Ein, Frank Ein Stein. Frank N. Stein was my father. [audience laughing] And who are you?
– I’m Professor Sprott’s worst nightmare, Igor.
– Hey, snap out of it, Igor! This isn’t a nightmare dream sequence.
– Oh, oh, excuse me.
– It’s the dream exploring the physics of time.
– Wow, oh, that was weird. Ooh, I guess I’m back now, yeah. Ooh, I kinda liked the nightmare sequence though. Maybe tomorrow night, okay?
– Frank: All right. Well, we can’t wait to see what you have in store for Professor Sprott tomorrow night, but for now, let’s focus our attention on what Peter pointed out with this light bulb, that seems to turn on instantly when I flip the switch. Yeah, it does seem to turn on instantly. But as it turns out, it’s not instantaneous. It does take some time. The reason is there’s an interaction between the charged particles in the wire and the electric and magnetic fields that govern their behavior. Let’s turn our attention now to what happens if I take some time to build charge up, but I release all of my built-up charge in a mere fraction of a second. To help us with that, there’s a Marx generator over there. And each of those bronze cylinders that you see are capacitors that can store up to 50,000 volts.
So we charge them up in parallel, but release them in series. So if I do some quick math here, there’s 10 of them, 50,000 volts each, carry the two, one, that’s half a million volts! Now that’s a lot of charge. When all of this charge is released at once, it creates one of the most powerful forces in all of nature. Shall we dare to try that?
– Audience: Yes.
– All right, so this will be, oh, and for the most dramatic effect, focus where Igor is pointing. Now, this will be loud, so I urge you, cover your ears. We’ll take 10 seconds to build a charge up, and then we’ll count down together so we know when it’ll be released, okay? All right, let’s go.
– In unison: Ten, nine, eight, seven, six, five, four, three, two.
– Remember to cover your ears, one! [Marx generator bangs] [triumphant music] Whoa, wasn’t that cool? We just produced a lightning bolt indoors. And who said physics wasn’t amazing? Where else could we get this close up to a lightning bolt and live to tell the tale? But now I’m reminded, we’re in a dream, so maybe we can be a little more destructive, explosive if you will.
– Boy: Woo!
– Yeah. So to help us with that, Igor has placed a wooden dowel in the path of those charged particles. Now, wood does not like charges to flow through it. We have half a million volts of them. So what do you think’s gonna happen? Let’s find out. So we’ll count down again from 10, and we’ll cover our ears ’cause again, it will be loud. All right, are we all ready?
– Audience: Yeah.
– Let’s go!
– In unison: Ten, nine, eight, seven, six, five, four, three, two.
– Cover your ears, one. [Marx generator bangs] [triumphant music] Oh, wow. Well, now more than ever, I think we can appreciate the advice of not sheltering under a tree during a lightning storm. Being under a tree [audience gasping] that gets hit by lightning certainly will not be pleasant.
– Isn’t that amazing? You didn’t think when you came in here this afternoon, you’d see an indoor lightning bolt, right? So let’s talk a little bit sports here, with our next demonstration. And we’ll have a little audience participation in just a few minutes, in just a few minutes. Okay, so I’m gonna start here with another type of capacitor. This type of capacitor right here is a little different than this one. This one only goes up to 5,000 volts, but it really contains more energy. There’s more energy in this capacitor. And the difference is rather than going across a gap to make a lightning bolt, when I release all of the electrons in this one, they’re gonna go through this copper coil right here. And this has a basket right here, and I have a Crush can.
And I’m gonna put that in the basket like so, and it takes about 10 seconds again to charge this up. This will be a little noisy again. And so just before I release the charge I’ll put my hand up, and you may wanna cover your ears at that point. So I will watch when it’s fully charged here on this galvanometer, and I’ll start charging it up. Again, it’ll take about 10 seconds and I’ll raise my hand at that point. We’re getting close, we’re getting up there. Here we go. [machine bangs] [can clatters] [triumphant music] [audience applauding] So you can see we have imploded the can, is what we did. What we made is an electronic can crusher, and we will be selling them in the lobby after the show. [audience laughing] No, just joking.
All right, so what happened here? I released all of the current, the current went through this copper coil, created a?
– Everyone: Magnet.
– The magnet created a current in the aluminum can. Aluminum is a good conductor, not as good as copper, but did create a current in here, which created an opposite magnet, which what?
– Audience: Crushed it.
– Crushed the can. All right, so by the way, you can crush a Crush can with this can crusher. [audience laughing] Say that three times real fast. We have an instant replay here. [audience oohing] All right, before the show started, I asked for a assistant, Gretchen here in the third row to help me out here and she has a baseball glove. So we’re gonna talk a little sports here. This time I’m gonna do exactly the same thing, except put, rather than put this Mountain Dew can in the basket, I’m just gonna put it on top, kinda like so.
And let’s watch what happens this time now. [“Take Me Out to the Ball Game”] [machine bangs] [audience cheering]
– Oh, good try. Good catch there. Let’s give Gretchen a hand here. [audience applauding]
– All right, for our last demo on electricity and magnetism, I will need a brave volunteer. All right, oh, so many hands. Okay, sure, you in the back. You, yep, you stretch your hand up [audience cheering] as high as can be. All right, thank you for volunteering. What’s your name?
– Miles.
– Miles?
– Yeah.
– All right, pleasure, Miles. Let’s walk over to the center here. And tell us, do you have electricity in your home?
– Yes.
– Yeah, what do you use your electricity for?
– Charging electronics.
– Okay, like for example?
– Lights, my phone.
– Your lights, your phone, yeah?
– Yeah.
– Is that it?
– Yeah. – Okay, that’s good enough, right? You gotta talk to people, right?
– Yeah.
– So, now let me ask you this. Your home has these like electrical outlets, right? Where you plug stuff in?
– Yes.
– Ever tried plugging, like, sticking a fork in there?
– I’ve seen those videos.
– Oh, so have you tried it? [audience laughing]
– No.
– No, does it end well for the people that do it?
– No.
– No, it doesn’t. We probably shouldn’t do that anyway. Home, work, school, do not stick things in plugs. But we’ll play around with electricity here under some pretty safe conditions, okay?
– Okay.
– At least they tell me it’s safe, I don’t know.
– Okay.
– Okay, so you’re gonna go over by Igor and he’s gonna get you situated in our electric chair– I mean our completely safe Faraday cage. [ominous music]
– Have a seat. Just keep your hands on the arms, okay? – All right, now, whatever you do, don’t feel the urge to grab that cage, okay?
– Darn, I’m feeling it right now.
– Okay, now I didn’t point this out before he went into the cage, but what does it say over Tesla coil?
– Boy: A million volts.
– It says a million volts.
– Miles: Oh God.
– Does anyone remember the Marx generator? How high did that one go?
– Audience: Half.
– Half a million volts. We have twice the voltage. Is this safe? Let’s find out. [audience laughing] Igor, whenever you’re ready. [electricity buzzing]
– Miles: I didn’t melt.
– Frank: Oh. [triumphant music] [audience applauding] Okay, well wait, wait, wait. You can’t applaud yet, we don’t know if he’s safe. Are you safe, Miles?
– I dunno, I felt something in my hair.
– Let’s see, well he’s walking. So I think we can applaud now.
– Give me five. All right! [audience applauding] Give him a hand.
– I think you made it out okay. All right, thank you for volunteering. So what happened? How is it that a piece of wood was blown to smithereens yet with twice the voltage, he’s walking to tell the tale? Well, I’ll give you a secret about charges. They do not like going on the inside of metals. So because he was inside the cage and didn’t stick his hands outside, those charges never got close to him. They could have been any voltage, and as long as you’re inside of a metal, you are safe. So we’ve learned in a lightning storm, under a tree, not a good idea, but inside of your car or any metallic enclosure will be safe provided you don’t stick your hand outside the car. And so now, I think we can appreciate that time plays a very important factor in electricity and magnetism. We can’t just slow it down. It will be difficult if not impossible to change time.
– And remember, time is what keeps everything from happening all at once. [audience chuckling] [Frank imitates an explosion] [audience applauding] [triumphant music]
– Igor: Looks like they had fun.
– Electricity and magnetism move at the speed of light, and nothing can move faster than that, but why? And how can it be that two people, one moving very fast and the other one at rest, could measure the same speed for a beam of light passing them by? Grappling with these puzzles, Professor Sprott dreamed of Albert Einstein and awoke with a start. [“Yakety Sax”]
– Oh, oh! [audience applauding] I was working in my lab and I fell asleep and I had these really weird dreams about the physics of time. And just as I was waking up, I thought I saw Albert Einstein riding by on a bicycle, but I must’ve been dreaming because he’s been dead for a really long time. [bicycle bell dinging] Oh my goodness, I must still be dreaming. It looks like Albert Einstein. Didn’t you die in 1955?
– Oh, I see, you’re thinking my cousin, Albert. You know, that happens all the time. People say we look alike, but I don’t really see the resemblance. [audience laughing]
– Hmm, didn’t Albert Einstein marry his cousin?
– Yeah, yeah, but different cousin. That was our mutual cousin, Elsa Einstein. It certainly made the family reunions more interesting. No, no, I’m Albert’s American cousin, Alphonse Einstein. But you can call me Al.
– Well, Al, you know, I’m really desperate to solve a problem here. We want to slow down time, and these other scientists haven’t given us much hope. You’re our last resort. Can you help us with this?
– Yeah, of course I can. In fact, it’s as easy as riding a bicycle. You see, what my dear cousin Albert realized, is that time, much like his second wife, Elsa, is relative. [audience laughing] The rate the time passes for you relative to someone else depends on your velocity relative to that person. If someone is at rest compared to you, and you’re moving fast, time will go slower for you. And the faster you go, the slower time will go for you compared to that other person. That sounds crazy, but it’s at the heart of Einstein’s theory of special relativity. And believe it or not, we can actually prove it with just a couple of very basic principles. You only need to accept two basic principles and we can check both of those principles today. Should we do it?
– Audience: Yeah!
– Yeah, okay. So the first principle is that the speed of light, as we’ve already heard, we heard about electricity, it’s similar with light. The speed of light is very fast, but it’s not infinite. And in fact, it’s a constant and it’s the same everywhere in the universe. And it’s the same, no matter how fast you’re moving, you’ll always measure the same speed of light. So let’s go ahead and measure the speed of light. So we’re gonna do a very similar experiment to the experiment the White Rabbit showed us for measuring sound, but now we’re gonna measure light instead, which is much faster. So we’re gonna use this circuit here.
– Boy: There it is!
– Yep, there it is. So we have an emitter here that’s just emitting pulses of light, and that’s that yellow pulse there on the scope. And we have a detector here. And right now, the detector’s not seeing any light because the light’s not coming around to the detector. We’re gonna use this fiber optic cable to guide the light around and take it from the emitter to the detector.
This fiber optic is basically like a wire or a pipe, but for light. And to understand that you can see here, we have a much larger version of it. And when I shine this laser pointer through, even though there’s these curves, the light goes around and around the curves and comes out the end here. This is the same idea. And these fiber optic cables are actually the backbone of the internet. Almost all of modern day communications happen over cables like this. So we’re gonna take one end and plug it into our emitter. We’re gonna take the other end and plug it into our detector. And we see a pulse showing up there. Nope, I adjusted the delay by accident there.
And you can see that the pulse basically arrives immediately after it was emitted. It looks as though the speed of light is infinite. But that’s because this is a very, very short fiber, so it’s not taking long enough for us to measure the decay for the light to get there. Let’s try again with this much longer fiber. This is a 20-meter-long fiber. So the light’s gonna have to go around and around and around these bends and that’s gonna take some time. And so hopefully we’ll be able to measure that delay now. So we plug one end into here and the other end into here. And now we see that our pulse shows up quite a bit later, about a hundred nanoseconds later. Now that’s a very short amount of time, but it’s enough time that we can measure it.
And if we do the same kind of math that we did for measuring the speed of sound, we’ll find that the speed of light is about a million times faster than the speed of sound. So now, the speed of light it turns out is three times 10 to the eight meters per second, or about 600, more than 600 million miles per hour. That’s really fast, but it’s not infinite. And the remarkable thing is I can make this, do this experiment anywhere in the universe. I can turn my experiment around. And even though the Earth is rotating around the Sun, is orbiting around the Sun at 67,000 miles per hour, and the Sun is going around the center of the galaxy at 450,000 miles per hour, it doesn’t matter how I do this experiment with respect to the motion of the Earth around the Sun or the Sun around the center of the galaxy, I’ll always get the same answer. And that’s the first principle in Einstein’s theory of special relativity. The speed of light is a constant, and you’ll get the same answer no matter how fast you’re moving or in what direction you’re moving. [triumphant music] [audience applauding] Okay, so now the second principle’s actually even easier to understand. And for this one, I’d like to do a thought experiment with all of you.
So imagine you’re sitting on a train and the train is stopped at the station. Imagine you’re holding a ball and you throw it straight up in the air. What’s gonna happen? If you throw it straight up, it’s gonna fall right back down into your hand, right? So let’s do that experiment. It’s not a very interesting experiment, but let’s do it anyway. We’ve got our train here. It’s got a little ball launcher in it, which is gonna be like your hand. And we’re gonna trigger it. Let’s see here. Let’s see if we can actually get it to go, okay. And the ball fell right back in the launcher, right? No big surprise.
But now let’s make the experiment a little bit more interesting. Imagine our train leaves the station and it’s moving fast. Let’s say a hundred miles per hour. And it’s moving at a constant hundred miles an hour. It’s not speeding up or slowing down. Now imagine you’re facing forwards towards the front of the train, towards the engine, and you throw that ball straight up in the air. Round of applause, how many of you think it’s gonna land in front of you? Not many people. How many of you think it’s gonna land behind you? [audience applauding] And how many of you think it’s gonna land right back in your hand? [audience applauding] Okay, well we’ve got some disagreement. So why don’t we try the experiment and see what happens? That’s the great thing about physics. We don’t have to argue about it, we can just find out.
So our train’s gonna leave the station. [triumphant music] Okay, we can watch that again in slow motion. So the idea here is that even before we threw the ball up, the launcher, the train, everything was already moving at the same velocity. Just like on the train, your hand, the air in the train, everything’s already moving at that constant velocity before you throw that ball up. So even once you’ve thrown it, it still moves with that same velocity, and it’s as if the train’s not moving at all. And that’s the second principle in Einstein’s theory of special relativity. It says that as long as you’re moving at a constant velocity, physics will act just as if you’re not moving at all. The laws of physics are the same in any frame, as long as it’s moving at a constant velocity. And with just those two basic principles that the speed of light is a constant, and that you’ll get the same answer no matter how fast you’re going, and that the laws of physics don’t depend on how fast you’re going, you can prove that time will slow down for you the faster you go compared to someone who’s at rest. Again, that sounds crazy, but we can actually prove it.
We can see evidence of it here in this room today. So let me show you that. Over here, we have a special kind of particle detector called a scintillation detector. Maybe we can dim the lights a little bit. You can see up here, it’s just a camera pointing at this detector. That green speckle is just noise. But every once in a while, we’re gonna see a bright streak pass through this detector, okay? I want you to keep watching while I’m talking and let me know when you see a good one. We have to be a little bit patient. So when we do see one of those streaks, those streaks are particles called muons. These muons are created by things called cosmic rays bombarding our upper atmosphere.
– There’s one. [audience gasping]
– Oh, it sounds like you saw a good one, good. So these muons are created by cosmic rays bombarding our upper atmosphere about 10 kilometers over our head and creating these particles called muons. Now, the funny thing is muons are very unstable. They only live for two millionths of a second, or two microseconds. And even though they’re traveling at close to the speed of light, two microseconds is only long enough for light to travel about 600 meters. So you would think these muons could never get down to us into our detectors. But we’re still seeing them. How can that be? Well, because they’re moving at close to the speed of light at 99. 8% the speed of light, time has slowed down for them compared to us.
So even though they only live for two millionths of a second to them, they live much longer to us. They live about 15 times longer, and that’s long enough for them to reach us here. And so we can see them on our detector. And in fact, there’s actually muons passing through you every second as a result of special relativity. [triumphant music] [audience applauding] But that’s all a little bit abstract, and it involves weird particles with weird names and cosmic rays. It sounds a little bit crazy. So let’s do a little bit more of a concrete demonstration. And for this, I need two volunteers who haven’t volunteered already. How about you there in the front row, and you there in the third row there, yep. Okay, come on down.
Okay, what’s your name?
– Ella.
– Ella, okay. And what’s your name?
– Andrea.
– Andrea and Ella. Okay, Ella, how old are you?
– Eight.
– Eight, okay, I’m gonna give you this clock. So you’re eight. And Andrea, how old are you?
– I’m almost eight.
– Almost eight, okay. Well, I’ll tell you what, for the purpose of this experiment, you’re both 36, okay? [audience laughing] Okay, so now, Ella, I want you to stand still and hold that clock. Andrea, I want you to run over to Peter there and then come running back. Okay, big round of applause for Andrea. [audience applauding] Andrea, you feel any different?
– A little.
– A little, yeah? Ella, you feel any different? Probably not, you’re just standing still, right? Yeah, well you’re right. Andrea, you’re actually a little bit younger now than Ella, even though you started out in this experiment at exactly the same age. Because you were moving and Ella was standing still, you’re a little bit younger now. And your clock will actually be running a little bit behind Ella’s. Okay, big round of applause for both Ella and Andrea. [audience applauding] You can take your seats now and I’ll take the clocks back. Okay, so now of course, these clocks aren’t precise enough for us to measure that kind of effect.
But physicists can build special kinds of clocks called atomic clocks that are precise enough to measure these effects. In the 1970s, physicists put one of these atomic clocks on an airplane and for the first time, saw time slowing down from flying in an airplane. So every time you get on an airplane, time slows down for you. But these days we can actually, physicists can actually build clocks that are so precise, they can even measure the effects of moving at the speed that Andrea was running at, or at the speed of riding a bicycle. And in fact, physicists are building one of those clocks in this building right here. [audience applauding] Now again, these are very small effects, but they actually impact our everyday lives. So for example, how many of you used GPS to get directions to the show today? Show of hands. Okay, well the way the GPS network actually works is that there’s a bunch of clocks in satellites, these very precise atomic clocks in satellites, orbiting the Earth above our heads. And each of those satellites is constantly broadcasting what time it is there. And your phone is picking up signals from a bunch of satellites and because of the constant speed of light that we measured over there, there’s a delay in the arrival time for each of those satellites, depending on how far away your phone is from that particular satellite.
And so by triangulating those delays and those distances from a bunch of different satellites, you can figure out where you are on Earth. That’s how GPS works. And the remarkable thing is because those satellites are in orbit and are whizzing over our heads, they’re moving pretty fast compared to us, and so time has slowed down for them. And if we didn’t account for the effects of time being slowed down for those satellites, GPS wouldn’t work at all. So we actually have to know about special relativity and about time slowing down in order to get those directions to the show on our phone. Okay, so I think I’ve solved all your problems now. All you need to do to slow down time is move really fast. Why do you think I’m so much livelier than my cousin Albert? I’ve been riding my bike around at close to the speed of light for pretty much my whole life. [audience laughing] That’s the secret to eternal youth. Just one piece of advice.
Don’t let the cops catch you doing that down University Avenue after the show, or you’ll get one heck of a speeding ticket. [audience laughing and applauding] So, I think I’ve solved all your problems and I’ve been standing still for far too long. I’d better get moving again. So, so long or as my cousin Albert would say, “Auf wiedersehen. ” [“You Can Call Me Al”] [audience applauding]
– So now we know that it is possible to slow down time, just as Professor Sprott wanted us to do. All of our problems are solved. Physics has saved the world. [audience cheering] [audience applauding] [cheerful music]
– Yes, it is possible to slow down time, but only by moving at 500 million miles an hour, or maybe by living next to a black hole. Since that’s not very practical, we’d better look for other ways to solve the many problems facing society. But one thing I know, physics will be important for solving these many problems, and especially the physics of time.
And I do dream of a better world even than the one we’re living in today, but we’d better be about solving our problems since time is of the essence. Now I’d like to end the show the way we’ve ended every one of the 300 shows over the past 37 years, by making for you a cloud. Some people think that making clouds is a way to combat one of our big problems of climate change. Actually, clouds have both a cooling and a warming effect. During the day, they cool us by shielding us from the warm rays of the Sun. While at night, they act like a blanket and they keep us warm. But here I have a container with 25 liters of liquid nitrogen, the same liquid nitrogen you’ve seen before. We force nitrogen gas into the container, that forces the liquid up into this pipe on top. The pipe has several dozen small holes. The liquid nitrogen comes out those holes still very cold, 321 degrees below 0 Fahrenheit, comes in contact with the warm air, and the water vapor in the air condenses into tiny droplets of liquid water.
And that’s what we call a cloud. And so with that, I thank you all for coming. [gas hisses] [cheerful music]
Search University Place Episodes
Related Stories from PBS Wisconsin's Blog

Donate to sign up. Activate and sign in to Passport. It's that easy to help PBS Wisconsin serve your community through media that educates, inspires, and entertains.
Make your membership gift today
Only for new users: Activate Passport using your code or email address
Already a member?
Look up my account
Need some help? Go to FAQ or visit PBS Passport Help
Need help accessing PBS Wisconsin anywhere?
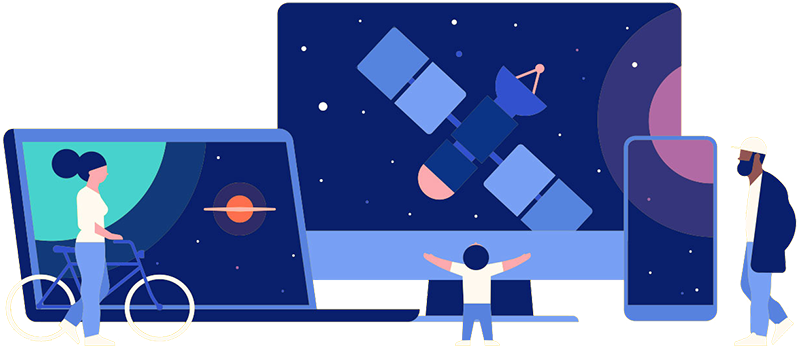
Online Access | Platform & Device Access | Cable or Satellite Access | Over-The-Air Access
Visit Access Guide
Need help accessing PBS Wisconsin anywhere?
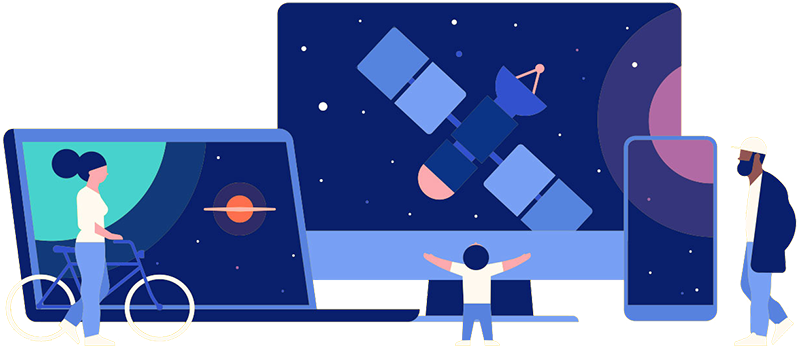
Visit Our
Live TV Access Guide
Online AccessPlatform & Device Access
Cable or Satellite Access
Over-The-Air Access
Visit Access Guide
Follow Us