– I’m Kevin Eliceiri. I’m from Morgridge and Biomedical Engineering on campus. I’m one of the coordinators of the conference, and thanks to everybody for coming today and coming for a great Science Saturday. We’re delighted to have today be part color in the Color Symposium, both Science Saturday and the Color Symposium. This is a public talk. You’re also welcome to stay afterwards for more of the research talks. But this public talk is really about engaging in color. I couldn’t think of any better speaker than Roger Hanlon, one of the great experts in color and camouflage, spent most of his career understanding the role of camouflage in marine life, and he really epitomizes collaboration. His work really transcends everything we talk about in this conference: art, computer science, perception, vision science, hardware developments, the list goes on and on. And you probably have seen his work if you’re a fan of PBS or Science, New York Times. His work has been featured many times. So really a real treat to have Roger Hanlon, and rather than hearing me speak, I’m going to hand it off to him and thanks, Roger, for coming. [applause]
– Well, thanks, Kevin. Can you hear me okay? Is the speaker on? Okay, fine. So, thanks, I really appreciate the invitation to come here. I’m hoping to entertain you today. Maybe you’ll even learn something along the way with our weird oceanic animals. And I’m going to take you diving for sure. So for all of you children, I’ve also got a little game embedded in the talk. So that’ll be fun, and you’ll get a little information about diving and all the neat animals that are underwater. So a big thank you to the organizers of this conference symposium, Terry, Karen, and Kevin. I really appreciate being here, so let’s go ahead and start and get some idea of what I’m going to really talk about. I’ll just point out right here, this is where I live. This is on Cape Cod in Massachusetts. And you can see we’re surrounded by water. So that’s one of the big reasons that I’m there. You see all the equipment that I take when I go diving. Getting pictures underwater is a little different from doing them on land. And then we have some of our animals up here, which I’ll introduce you to. But I want to talk first about camouflage, not exclusively about it, but it’s something these animals do particularly well. And so what is camouflage and how do you describe it? Well, here’s Hollywood’s version of what camouflage is. You know, all you children I think will definitely know who that character is. What I’m going to talk about today is an animal system that’s actually a little more bizarre than Harry Potter, but it’s the actual thing. It’s not an imagination of Hollywood. So let’s take a look at this and think about art for a moment. This is a very impressionistic image right here, and I want you to look very carefully to the next image, which is coming right now. It’s changing a little bit. Watch right in here. Changes a little bit more. And now it changes to this, and that is actually a photograph that I took. And right there is a cuttlefish. And it’s really an amazing bit of work this animal is doing to look like that, because there’s the animal right there and it just disappears in front of your eyes as soon as you put it on the right background.
So there’s a lot going on here in terms of masking the real edge of the animal, creating false edges, distracting attention. So this is a very sophisticated visual illusion that’s going on that these animals are doing. And they do this repeatedly on different backgrounds. So how does art inform science? A lot of you will be familiar with this famous painting. And as we sort of focus down a little bit, now we go down to the ocean and we have sort of a tree here, we have fish, and we have a lot of sand, wondering why we’re watching this. And all the sudden, wow. Where did that octopus come from. Did any of you kids see that octopus? [laughter] So now here’s the same octopus a little while later looking bigger than it really is and displaying to me and it’s kind of standing on two or three of its arms. It’s a very athletic move and making itself look big and dark and fearsome. So this is what they do in defense. But let’s go back to that camouflage for a minute, and let’s take a look at that. Smooth white skin, a ring develops around the eye. That’s five million little pixels in the skin.
They’re all controlled by the brain like electric skin. Another 25 million create the pattern. Here it comes. Watch the smooth outline of the body very carefully. Now you see the skin has morphed into 3D. This is like ultimate goosebumps, if you will. So the skin is really cool. And the optical trick works very well because if they kept smooth skin then you’d have the rough edge of the algae, then smooth skin, and a predator would see the octopus better. So this is a really useful kind of thing to do. So they have complete control over that. So there’s before and after. So the animal’s really changed its appearance dramatically. And the idea is that here’s the octopus eye and there’s another eye over here. They have their eyes where our ears are. Their eyes are looking this way. They’re not looking like this. So the right eye is looking at the calcareous algae, the left eye is looking at the sand, and the animal has to make a decision of which camouflage pattern to use, one that works on sand or one that works on the algae. And you can see the answer. So the animal is looking at it, and it’s looking at the pattern of this, making its posture fit in, the intensity of the pattern is similar, the color is correct, and the 3D texture. So the camouflage has a lot of aspects that have to be matched for that kind of camouflage. So it’s doing a good job. If the octopus goes here or over to that part of the reef, there’s actually an octopus right in the middle of that. I don’t expect you to see it unless you have binoculars, but even if you did, you probably wouldn’t see it. Or it can go to this really well-developed coral reef.
And my point here is that a coral reef is the most complicated visual environment on the planet. There’s more structure, there’s more color, there’s more contrast, more shape than you can get anywhere else, including a tropical rainforest. And these animals are going anywhere and in less than one second they’re deploying perfect camouflage. So this has been driving me kind of nuts for a long time. How do they do it, and how do they do it so fast? And so I’m going to give you a few of the answers that we’ve come up with. I’ve got a large collaborative team that have worked on this. So how and why do they do that? So let’s investigate this a little more and see where it can take us. But first I’m going to talk about squids. This is not camouflage. Here’s the male squid and here’s the female squid, and he’s been courting here all day long and fighting the other males and they use color change too. Here are two males fighting each other. The female’s over there. And look at the patterning put on. This is really a dramatic fight. There’s a pattern on the bottom of this animal, on the top of that animal, and they’re doing this gentlemanly fight and posturing. They don’t really fight and bit too much, although there’s a little nip at the end coming right about here. A little nip there. And this guy swims back. This one does, and he wins the fight and goes back with his girlfriend. [laughter] Now, watch here. Here’s the male. There’s the female. He’s putting a white pattern on to scare other males away. If he shows it to the female, she’ll leave. Watch him when she changes places. Watch what he did.
[laughter] He just put the fighting pattern on the opposite side. So they’re using their visual thing to give dual signals. This is love. That is hate. [laughter] And so they’re very, very elegant about how they do this. So this is sort of, you know, a dating scene, and if he makes a mistake and shows her the white powder and she hits the road instantly and he wasted a whole afternoon and a lot of money, so to speak, dating her and it didn’t work out. So there’s a lot of dramatic things going on using this changeable coloration, which is really kind of cool. So, now, camouflage is not what you think it is. I showed you an image in which the camouflage looked a lot like the background, but that’s not the only way camouflage works. I’m going to show you something very different in terms of a octopus on a Pacific reef. And here is it, perambulating along. And when they move, they change all the time because if it’s moving, the camouflage doesn’t work so well. So they change their appearance and they change their appearance 170 times per hour when they’re out foraging, so you can’t form a search image. But watch what happens when the octopus decides to park here for a little bit. It gets up on the promontory and says, okay, I’m going to look around here quickly and decide what camo pattern I use, and, whap, it’s got it. So now I ask you, does that octopus look exactly like any of the surrounding corals?
– No.
– Okay, if anyone says yes, you probably ought to leave now. [laughter] So, no, it doesn’t. And this is the coolest thing about this because I call this a generalist solution to a very complicated problem because there’s a lot of visual information and they don’t have to look exactly like the background, but it still works. But why is that and how does that work and how did they make that choice is one of the things we’re trying to figure out. So it begs a fundamental question: how many camouflage patterns are there on Earth? How many does an octopus have to go on a coral reef? How many does a baby deer have to go hide in the grass? These are questions that have never even been posed in biology. I’ve searched literature. I can’t find it. And so here we have an animal that goes out there and camouflages all the time. So if I look at our cuttlefish right here, any individual cuttlefish can look like all of those things and even more. So it looks like a big mess. Too much data to mess with. And how many patterns is that? Octopus, same way. So we looked at this very carefully, did a lot of diving, did a lot of photography, and we came up with a big surprise. And what we did was very crude. It was kind of like what we might do in a beginning art class or even kindergarten, which is I had a bunch of photographs and I started putting them in piles. If they looked sort of alike, I went, “It goes in that pile. You know, there’s not much science behind that. But I only have four or five piles, and I went, “I have to get more pictures.” Well, now I have a photo library of over 100,000 images, and there’s still only four or five piles. What? That sounds crazy. So let’s see how this works. One of those piles has what we call a uniform pattern, and that means there’s little or no contrast. The color and the pattern are the same from one end of the animal to the other.
And on a uniform background that can create some pretty good camos you saw in the first one. But there’s another thing we call mottle, and it’s got light and dark little bits in here, some repetition of the pattern, and only moderate contrast. The difference between the light and the dark is not very great. And that on a different background can create some blending in as well. But now we’re in for a big surprise in more than one way. And that is this:
– For disruptive coloration we now have a very different allay of large scale, high contrast, light and dark components, multiple orientations and scales here, partly to break up the recognizable form, but of course, as shown here on the right background, also creating a fair amount of background matching as well.
– So my lab had fun dressing me for that one. But you get the picture, hopefully, a little bit from that. And the idea is, why three patterns and how does that work? Well, I don’t have time to go into the details of that, but it turns out that you don’t have to look exactly like the background. You can either do something not to be detected or, if you’re going to be detected, not to be recognized. And that crazy last pattern of disruptive coloration cuts down the recognition even though out of context it’s quite conspicuous. So is this a universal phenomenon? Are all animals constrained or just able to use those three basic pattern types? We’ve looked at a lot of animals. You know, we got primates and amphibians and reptiles and fishes and insects. There’s a lot of uniform coloration. No big surprise there. There’s a lot of mottled coloration. We threw some birds in for good flavor here. And there’s a lot of mottle. This is what hunters use. They use mottle coloration more than anything else. Same with soldiers. But if you look in the animal kingdom, there’s a lot of disruptive coloration. And disruptive coloration has bright black and white high-contrast markings, which only in certain backgrounds work real well. An extreme example is a panda bear. You say, that’s camouflage? This guy is nutso. Well, it turns out that if you view that animal up against the sunlit sky, bright sunlight, deep shadow, washes out the retina. This is bright light, that’s deep shadow, you no longer recognize the outline of a bear. That’s sort of one of the concepts that’s going on there. So there’s a lot of this going on, but it’s hard to prove exactly how it works. So, who cares? Well, the idea is that if you only have three pattern types in all animals, it’s a very profound kind of discovery. It means that it’s found a way to deceive all these visual predators on the planet. And we think this is what’s happening with the pattern designs that nature has forged. There are hundreds of visual systems out there eating these animals, and so it’s responding to a lot of selective pressure. And they’ve changed so fast that it’s just mind boggling how that can happen. And we’ll tell a little bit about that. Well, this is a controversial idea. I don’t mind a little controversy because I’m old enough I can’t get turned down for tenure. I don’t have tenure anyway where I work so it doesn’t matter. But I’ve actually published this back in 2007. And it is controversial, but no one has a better hypothesis out there.
In other words, my hypothesis is there’s a thousand camouflage patterns. Okay, prove that to me and tell me how that works. Well, no one’s doing that. So art and science. Here’s the same animal looking very different, right? And one’s camouflaged, one’s conspicuous. Well, I held a conference years ago with artists and scientists, and we looked at these features, you know, how light it is, the shape of it, the edges, and we tried to figure out who has those in common. Well, these are what artists and photographers and architects, makeup artists, you know, marketing and advertising, they’re all manipulating these features to look very differently, in this case the way the cuttlefish is. So we actually worked on that and brought artists in to really work on this. Here you see artists deal with these issues all the time in how they present things to human beings.
And I particularly like Bev Doolittle who understands disruptive coloration better than biologists do. And I really love this book because, you know, you got this horse right here and it’s got this bright high-contrast pattern on it. In the right background, it does a number of visual tricks. You can look at this beautiful impressionistic painting, and on a two-dimensional palette, you get the impression of three dimensions. Because you have waves in motion, you know that’s in the distance and that’s in the foreground. That’s a real talent to be able to paint something like that and give the impression of three dimensions. And so the cuttlefish is doing the same thing because the cuttlefish is a three-dimensional object on a two-dimensional background, and it’s flattening itself visually into the background, which is a very cool trick. So we’ve looked at this edge design of the animal in great care, and I was teaching a course in an art school in Rhode Island and this is one of the students who decided to take her 3D shape and flatten it into the background. And this is a student who really takes her work seriously. She painted herself and put herself up against the wall. She got an A for sure, believe me. [laughter] I thought that was very inventive. I don’t know how long it took her to get the paint off, much less put it on. Now we look at architecture and we can kind of look at that here, too. We might say that if you look at a home design, this home design is meant to be stared at. I mean, you cannot miss that. This is the conspicuous view of it. Whereas, if you get this beautiful home near Big Sur, California, it’s taking use of the ambient trees and the shape and the color and the texture and it’s blending in what I might call it camouflaged house.
And, in buildings downtown, this is standing out. This building with greenery on it is not standing out nearly as much. And out in the desert you can have a house that stands out or doesn’t. The point is our cuttlefish do the same thing. They can either stand out or they can blend in. That’s a very big cuttlefish, and it’s blending in very well to the background. So there are a lot of ways you can do this, and with study we did, we were looking at all those aspects of camouflage. So let’s move on from there and ask the question of how effective is camouflage? Now, all you kids, we’re going to have some fun now. This is a test to see if you can find Waldo. Does everyone know the “Where’s Waldo?” game? We’re going to play that a little bit, and there’s a little music. So… As we go and do this, here’s what I’m going to do. I’m going to flash up some pictures of octopus. They’re only going to be there for two seconds. One, two, next picture. One, two, next picture. You have to find the octopus. And I’m going to help you on the first few, but then you’re just going to have to look and see how many you can find. Okay? So, here we go in just a moment, about now. That’s easy. That’s pretty easy. Uh-oh. [laughter] There it is. Look again. There it is. See it? Now this is an easy one. One, two, three. Parks right here. There’s one going to do the same thing up on that rock.
There’s a closeup to prove it’s an octopus. There’s one in the middle. That one’s hard to see. Now this one’s going to swim away to a new rock. There it is swimming Do you see it? Closer. Closer. It’s right there. Now we’re going to California and the kelp forest. The animal is right in the middle of the picture. There’s the closeup. Also in the middle of the picture. Closeup. Now it’s going to go in for a lunar landing here. It goes down on the sand, digs in a little bit, changes the pattern. It’s right there, but you can’t see it. And there’s a self-portrait. [laughter] Okay. All right. So we have a little fun with that. Now, did anybody see all the octopuses? [laughter] They’re hard to see, I’ll tell you. So it’s really hard to pick them out, but there was an octopus in every picture. Okay, so let’s move on from there and go to the next thing. And we’ll talk about color in the eye of the beholder. Now, it’s one thing that this is a symposium on color, and how do you measure color in the eye of someone other than a human? That’s really hard to do. We don’t know what a barracuda can see. But we have developed a new camera to do this. So I’m going to take you on a dive trip for four minutes and show you this idea we’ve done to try and approach this problem.
– This is the center of the heart of everything alive on planet Earth. This is the place to look for something new.
– The thrill of being in the field, there’s just nothing like it. The ah-ha moments come out here in the animal’s world. That’s the ship we work from. We’ve come here because Raja Ampat has shallow, colorful, clear water coral reefs. That is an ideal situation to get maximum color in our technological new camera. The basis of the expedition is to try and understand what animals see in their color world, not in the human color context. So we have a new development of a very special technological camera we’ve just developed called a hyperspectral imager. This is a big step forward in biology. No one’s ever been able to do this before. The focus is really strange. [inaudible] Something’s not fitting, I said.
– I’m Derya Akkaynak. I’m from a fishing village on the west coast of Turkey. Water and islands has always been in my family, and our house back in Turkey situated on a beach where there’s plenty of cuttlefish and octopus.
– Derya is along on this trip for a very good reason. I’ve literally called her the queen of color, and that’s a compliment. For this kind of trip and this kind of study, she has the ideal skill sets that she can bring to this. Number one, she understands color in great detail. She understands how to pull the data out of the hyperspectral imager and how to analyze it and to collate it very quickly. Moreover, she’s a fantastically solid scientific diver, and this is very important. You can’t go down and do research underwater unless you are a really comfortable and accomplished diver. So it’s a pleasure to have someone as talented as she is as a bright young scientist. The HSI is just an acronym for hyperspectral imager. So a typical human-made device, a smartphone or whatever, has three colors in every pixel, red, green, and blue. Ours has 16 colors, and they’re not tuned just to human vision. They’re tuned right across the full visible spectrum, including the ultraviolet because other animals can see those colors that we do not see. And so the idea is we want to understand what the color world looks like in the eye of the beholder. The animal out there on the reef under natural lighting conditions. Not humans with a human-made machine and our somewhat restricted color gamut that we work with. There it is. The father and his baby. [laughter] This is the first time after five years of chasing this down. So I’m real proud of this thing. We’ll see if it works on this dive. This is the moment of truth, if ever there was one.
– This is a big camera, and it’s not necessarily very ergonomic to use. It’s not necessarily diver-friendly. To swim around with it and maintain your buoyancy and make sure the dome port does not scratch, it takes a little bit of practice, but then you get used to it. We’re studying cuttlefish and octopus specifically on this trip. They camouflage. And when we capture a scene with one of the animals camouflaged, we can then say, how would an eel with a visual system of these properties sense this scene?
– Today was spectacular because the housing worked beautifully, the camera seemed to work beautifully, it handles like a dream underwater, which I never imagined, and a one-hour dive suddenly everything worked after all this time. I’m a real happy guy right now because I didn’t expect it to come together on the first dive and we did. We got really lucky, but luck’s a big part of research so we’ll take all we can get.
– Okay, so we’re trying to figure exactly how to work out those images, so I can’t give you any data on that right now. Back to cuttlefish, here’s some of the skin changing for a cuttlefish that’s fighting. You can see that in the skin there’s a lot going on in that skin. It’s very much under control. These are millions and millions of little chromatophores in the skin, innervated directly from the brain, as I said earlier. So there’s a lot going on even with the fighting and not the camo. What I want to show you now, since this is a color symposium, is just a few images of how beautiful these animals are in their skin. So we’ll just look at that very briefly. There’s an octopus. There’s a different octopus. Again and again. You can see there’s a lot of color diversity, a lot of pattern diversity. Much like birds and insects or lots of animals. But look at this blanket octopus, Tremoctopus. This is an incredible creature that lives in deep water. It comes up to shallow water occasionally. They can change their color and pattern too. If we look at cuttlefish, that’s the giant Australian cuttlefish. There it is again, camouflaging. You’ve got the little pajama squid, aptly named for its stripe pattern. And then this is the flamboyant cuttlefish, which is truly bizarre. Beautiful animal. And then we look at squids right there. And you can see a baby. This is a hatchling squid that just came out of the egg. It’s ready to go. The chromatophores are in good shape right away. There’s a closeup of its head and chromatophores. And then the grown up animal right there. Very beautiful indeed. Lots of blue iridescence and green patterning.
And so we’ll look at the skin in a little more detail. And here you see a cuttlefish. I’m filming this at night, and the animal has got transverse bars, light ones and little dark ones, little dark spots. You see these waves of color going back and forth. A little bit of mottle. Now it’s gone kind of yellowy. And now it’s going to sit on the bottom and park there. It’s got its landing gear down right there, and plops on the bottom for some camouflage. So they can do that very well. So the idea is if you have changeable camouflage, you have to control it. And these are eyes are very large and they have a very large brain and they’re visually looking at things. Just like I showed you the squids at the beginning, the female’s on this side, the female’s on that side. What do I show? It’s all visually controlled. And they have this wonderful skin also. And one of the questions is whether we can make changeable materials that we might use based on the skin of these animals. And I’ll show you some of that. But first, here’s how the skin works. You have yellow and red chromatophores. You got muscles attached. When the muscles contract, it pulls that little bag of marbles, the pigment, out into a disc of color. And when there is no excitation by the nerves, then the muscle relaxes and, boink, it just goes back into a little dot that you can’t see. So it’s an open/close kind of system. Underneath you have all this beautiful blue and orange and pink and green iridescence, and you’ve got a white base layer so that by three layers of skin you have everything in the artist palette, so that skin is very beautiful.
Now, I bring this up to remind everyone, I’m sure everyone in the crowd, just about, has a smartphone. But I ask you, where does the color and pattern come from out of your smartphone? It comes out of a battery and a computer that costs a lot of money and the battery runs out by the end of the day and you go out in the middle of the day and you can’t see it because there’s too much light falling on it, right? Well, our animals are doing everything that you see there for color and pattern, and they’re not creating any light. They’re only using available light. So why don’t we create devices that are as efficient as the skin of this animal to use available light? That’s kind of the burning question. So we’ve studied this, and the secret is that they use a combination of pigments and reflectors in the skin. Pigments, a red pigment, like some of the red sweaters in here– There’s a lot of red in Wisconsin– is absorbing all those other colors and reflecting red out. So absorption is very important. Reflectors do not absorb anything. They just reflect light and they operate by a very different method. Look at this iridescence on this squid right here. Look how beautiful that color is. There’s iridescence on the eye. It’s silver. There’s a little bit on the arm, and you got pigments up here. So the skin is very delicate and it’s very beautiful and it’s very changeable. It’s hard to imagine a more delicate-looking animal than this. It’s also a fast swimmer. So if you look at the skin of that animal, you see no iridescence, and out of nowhere it pops up. And so where did that iridescence come from? And watch the shift, first it shows up, then it goes from red all the way over to green. It goes right across the rainbow. And it’s beautifully controlled.
And you’ve also got a squid like this that has these bands of color. And if we look closer and closer, you see that some chromatophores are open, some are not open. So you get a pattern in the skin. So that’s basically how that happens. And I’ll move on. If you look at those chromatophores, they’re opening and closing. They’re either tiny and you don’t see them, or they’re open and you do see them. And you can see the muscles radiating out here, as I showed you in the diagram earlier. And now just look at the skin and how different and beautiful it can be. So you get about all the colors you can think of with different species. There are about 700 species of cephalopods. Maybe as many as a thousand. That’s not very many in the animal world. There’s 20,000 Tilaiya fishes and I don’t know how many insects, tens of thousands, maybe hundreds. Anyway, I showed this kind of image at a TEDx talk to the company Este Lauder, which is a cosmetics company. And the title of my talk was that, “Nature’s Cosmetic Champions: The Octopus Garden of Dynamically Changing,” and told them that if you want to have changeable cosmetics in 10 years, look at this animal group and then you can have mascara and eyeliner and rouge and you can control it yourself because it’s exactly what the animals are doing. It’s a viable animal model to go in that direction. It needs a lot more study. Here’s an individual chromatophore. And there’s a grid mark down here, you can barely see it, and the point is that the skin in these animals is moving all the time. And the chromatophore has to got open and still give the right color and the right pattern. So the animals have this beautiful flexible organ that cannot accommodate all of the movement. And we’ve actually looked at this, and some colleagues in Harvard engineering have patented this idea of a chromatophore, maybe to make displays in the future.
Now, there’s white in there too, and I’ll quickly go through this. This is some of the whitest white in the animal kingdom. And if you look at the animal very carefully, you see the white here. And I’m putting a neural electrode here and I electrocute it here, and electrical there. And you see that you can create patterns, very strong, high-contrast patterns by this relatively simple mechanism of pigments and reflectors working together. And we’ve looked at the skin very carefully and isolated one individual cell that creates the whiteness, and we’ve modeled that right here. And the reason I’m telling you this is that we can model this and give it to a nanotechnologist who then can make structures like this at the level of 200 or 500 nanometers, which is really tiny, and create some efficiency in reflecting available light. That’s the idea. And so we’ve done a lot of work, and maybe they can make a better Kindle, which is the reading device that uses just ambient light, which only has 40% efficiency of the light it reflects. Our animals have 70% to 90% reflectance. If Kindle could just get that 40% up to 50%, they’d sell a lot, you’d buy a lot more Kindles in other words, and they’d be happy and you’d be happy. Okay, so we’ve worked with engineers and others. We published this paper looking at biological displays and man-made displays and tried to show the parallels of where the deficiencies are here and the strong points and where the deficiencies and strong points are here so that engineers and material scientists can get together with the biologists, learn something, and implement it in the manufacturing world. So that’s all going ahead.
Very quickly, here’s some of the toys that I play with, mainly cameras everywhere. Putting them in underwater housings. Here’s some of the underwater housings. We use spectrometers to measure light underwater. We use underwater vehicles sometimes. Although, even though they’re cool on television, I don’t like them because they can’t do anything near what a diver can do underwater, and they’re all made for different projects. We culture animals in the laboratory. We use a lot of microscopes. But, this is still one of the most important things right there. The human eye and the human brain as an observing machine. It’s really unparalleled. So we don’t forget that. My work does take me around the world. These are some of my primary diving sites around the world. So I’m a traveling fool whether I like it or not. And now I’m going to speak to you very quickly about how we test how the animals look at a background and pick out the right camo pattern. And this is really quite an interesting hide and seek challenge. The idea is that if there are only a few camo patterns, as I suggested earlier, they only have to look for one visual cue to turn on one pattern and one other visual cue for another and they can ignore most of that complicated coral reef. Can we prove that in the laboratory? Well, we’ve now, over 10 years, published 30 papers, experimental papers, and I think we have some of that answer. Basically, we put a cuttlefish in a tank, we give it a background, and it either puts out one of these patterns. So we can see the answer quickly. Put a cuttlefish in uniform pattern, it goes uniform. Put it in a non-uniform pattern, it goes mottle. And we amp up the size scale of the checkerboard, and the animal has morphed into a disruptive pattern. The only difference between these two is the size and the contrast of the checker. And so that is the visual cue, here, is when the white checker is about the same size as the white unit on the skin, they’ll use that as the signal to go into disruptive camouflage. So it’s a relatively simple method that shortcuts all kinds of brain processing because if they did that, they’d have to have the brain the size of a Volkswagen, and they don’t. So it’s really kind of a cool system. So I’ve just summarized a lot of data there. You’ll have to believe me. But look at the cuttlefish.
Uniform pattern on a uniform background. We pull the rug out from under it. Underneath is a different kind of background. The animal is looking at that background right now, all upset because we did that so rudely, puts on a mottle pattern, and that little white square which is disruptive, that would go away if we gave it a few moments and we didn’t do that so quickly but we don’t. Instead, our special, talented, three-handed technician reigns in these white rocks from heaven. And the animal looks at the white rocks, moves over here, and puts on a disruptive pattern because, like the checkerboard, when the white objects are high contrasted about the size of that, that’s the signal they use to shift their camouflage tactic. And it’s really quite amazing to see this happen underwater and with stuff as stupid as checkerboards. There are no checkerboards underwater. It’s that they’re not looking to be like a checkerboard, they’re looking at the white splotch on a dark background. That’s the signal. Okay, so look at this octopus right here doing the moving rock trick. Watch what happens. The pattern changes when it comes to the kelp. Now, it went to the color of the kelp and it went to the smooth skin texture of the kelp. This is supposed to be a colorblind animal. How did it get that color match done so well? And now what I’m doing is I’m turning my underwater lights off on this side and on that side. The color match at 70 feet where there’s not much color is easy. But I had video lights on and sunlight and the color match was still good and still changed appropriately. So whether or not they have color vision, you know, we scientists have not been able to find out whether they do, but I think they’ve got it by some other mechanism. So there’s a lot to learn there.
Now this is the old moving rock trick. This is another thing out on the Tahiti coral reef. Here the octopus has to move across an open area that’s very dangerous, a lot of predators. And it makes itself look like a piece of coral and holds that posture and then tippy toes across the substrate like this. But it’s going very slowly and it’s not going faster than the ambient light from the sunlight dapple on the waves. And on a day where it’s really rocky like that, they take about 20-30 seconds to cross there, and on the next day when it was calm and it was cloudy, they took about three minutes to cross there. So the animals are calculating how much fake motion is out there and they’re equaling that amount of motion. It’s really quite sophisticated, the brains of what these animals can do cognitively. Okay, a little bit about signaling and then we’ll wrap up. Here’s an octopus that I’m approaching fast on purpose to get its reaction to me. And white is extremely important in these patterns. White’s a complicated color. White is all colors combined. And this is really white white. So you can see a lot of whiteness. And look at the giant Australian cuttlefish. This one is doing the passing cloud. This one’s making its arms very, very white and flaring them out in the beginning of a fighting display. So the white is a very crucial part of this signaling. I’ll finish up by showing fishes that change. You saw that change right there. Watch the vertical bar show up here. Here it comes right in a moment. Right there. And those are pigments and notice that it was fast. So it turns out that the cephalopods are not the only fast ones out there. So there’s some fishes out there that do this very fast too by direct neural control. They also have the structural coloration in here, but these animals have not been studied and there are 20,000 species of marine fishes and they’re totally unstudied. So anyone who wants to be a diver and study fish color change, you got a gold mine to look at here. I’m trying to convince my lazy ichthyologist to go look at some of this. Okay, so with that, a few summary thoughts. Color and pattern are very well developed in the ocean.
If you think about it, the ocean’s been out there much longer than the land animals. They had a lot more time to evolve some sophisticated patterns. There’s a lot of color underwater, especially in shallow water. Many of these subjects are very poorly studied, though. Biophotonics, that fancy word I haven’t used yet, but that’s just looking at light and pattern and color in a biological animal. That’s what that is. This is a field that’s really getting a lot of attention now. And this is a case where science and art and technology really come together. We don’t have to force ourselves to talk about art and science in something that’s as visual as this. And, you know, I work with artists. I have artists in residence in my laboratory. I teach courses occasionally at Rhode Island School of Design, and we really have some very fruitful collaborations. So, with that, I’d like to finish and just with this statement right here. This has been sitting in the library where I work at the Marine Biological Laboratory. It was put in there, it was 1873 is when Louis Agassiz came up with that statement. That’s sitting in the library. And sometimes to amuse myself I go to the library and I just watch that sign and watch all the students go in there and read books. And I’m going, get out of the library, go out there and find out what’s going on. And it’s my excuse to go diving. So, anyway, thank you very much. I’d be happy to take questions. [applause] So the question is that I hinted that all the control, this is not exclusively through the eyes. And because they don’t seem to have color vision, we decided to look around and see if there might be something in the skin. So we did a quick and dirty gene search because the opsin molecule, that is the molecule in the retina that actually is part of the light detection mechanism that turns into vision, the gene for that molecule was known and we did a quick and dirty gene search and found it all over the skin. So we did a lot more work on that. So, essentially, what we’ve found is that cuttlefish or an octopus, they have the molecules that are detecting light in the eye, those same molecules are spread all throughout the skin. We’ve not been able to really prove that they can do anything with light, but they’re all over the place.
And for those of you biologists will appreciate that all it is is molecules over the skin. They’re not aggregated into a cell or a tissue or an organ. Just a whole bunch of little molecules out there. So it’s hard for us to imagine how they could function, but we’re just ignorant of the whole process. There’s something there and we have to figure it out. So far no go. Too complicated for us. So I hope that helps. Yes? Oh, stereoscopic. The colors. They do not have binocular vision, if that’s what you mean. Except when a squid, I told you an octopus has its eyes here, and so does the cuttlefish and so do the squid, but squids can come up, and when they’re ready to launch their tentacles, their two tentacles to feed, they’ll scrunch their eyes forward to get some stereopsis just for a moment. And then they’ll flare their arms and launch the tentacles. So just for a moment they can have the stereoscopy or whatever that word is.
And so it’s just very, very briefly. So most of the time it’s essentially an animal that’s using one eye on the starboard side and one on the port side. Of course, this is what brains have to do. They have to integrate that information from two sides to get it right. And by the way, they never put one camouflage pattern on this side and one on that side. They always integrate that pattern, which is another interesting thing that brains can do very well. Yeah, I was wondering what is the greatest depth in the ocean at which you’ve done this type of research, and is there any correlation between depth of the ocean and the presence of camouflage animals or bioluminescence?
– Yeah, so I’m not a deep diver kind of guy. I like to have time underwater to get data. I have done some deep diving when I was young and foolish, and I survived that, thank goodness. Two things about that. Number one, the full spectrum of color is in the shallow water, shallow, clear water. The reds, which are long wavelength colors, warm colors, they get attenuated out very quickly. You don’t see any red below about 20 or 30 feet, even in clear water. It’s just gone. But there’s a lot of red in that shallow water. So we do depth work down to maybe 60 or 100 feet and depending on the different light field. Now, many animals are way deeper than 100 feet. Some of them are out there in a couple thousand feet. Light goes down to about 900 feet. Below 900 feet, no light from the sun can go below that. But there are animals down there, and they have bioluminescence. They have organs on their skin, photophores, that create light. And it’s always in that kind of blueish range as well. And they are using camouflage because animals in that habitat, the predators will look up against downwelling light because they vertically migrate at night and there’ll be moonlight or starlight, and the predators eat shadows and silhouettes, which are squids or other fishes or shrimps. So what do they do in defense? Those animals put on bioluminescence patterns on their ventral side, on their bottom side, and they have what’s called extraocular photo receptors on the top of their brain, that detect the amount of downwelling light and they turn on the downwelling bioluminescence on their body to equal the downwelling light so that they obliterate their shadow and they don’t get eaten. That is a very slick system. It’s a very sophisticated system.
And so, yes, this camouflage is going on everywhere. Even in places where there’s no natural light, there’s bioluminescent light. So if you go down in a submersible to 5,000 feet and you turn all the lights off on your vehicle, the bioluminescence will show up. Question here? Okay, so she wants to know if I study the octopus’ eye and how do I know they’re colorblind? Yes, we study octopus eyes and we do a lot of tests on them to try and figure out what they can see, so I’ll give you an example. What we do is we’ll make a background with some rocks in it, and we’ll make them different colors. But we make sure that each color is exactly the right and same brightness so that the only difference between the rock is the color. And if you’re colorblind, those rocks will all look the same, but if you can see color, they’ll look very different. And we decide which camouflage pattern they turn on because if the rocks are difference color, they might put a mottle pattern on or a disruptive pattern. But if they’re colorblind, they just see it as a uniform background and they put on uniform. That’s how we do the experiment. And we’ve done quite a few of those experiments. There’s other ways to do that. You can look in the eye and you can look with electrophysiology, which is a big word, but you can look at the retina and you can see how it responds to light. So that’s another way to study it. And then we can look at the eye itself. So in your eye you can see color because you have rods and cones in your eyes. The cones are the color receptors. The rods are for black and white. And if you look at the eye in an octopus, it doesn’t have any cones. It only has one kind of photo receptor that’s a little bit like a rod. So they don’t have the equipment in their eye to perceive color the way we know color is in many animals. So, you know, I still think they can see color. [laughter] I do. I’m serious. But I have no clue how. So maybe you could study that when you go to grad school. So… [laughter] Yeah?
– So the animal that change their camouflage when you put the white rocks, my question is that are they getting a kind of like gestalt feel of the thing and that’s why thhey change?
– A gestalt what?
– A more wholesome view of that environment and that’s why it changed the camouflage from the first pattern to the second pattern? Because still the first pattern could work.
– The first pattern could work. That’s a very good observation. So there’s a priority rule, apparently, in their algorithm. And we’ve shown this before. So if that little cuttlefish when it went into the mottle pattern had stayed where it was, 80% of the visual field would say go and stay in mottle. Instead only 20% of the visual field, represented by the white rocks, they paid attention to that with a priority. So there is a priority rule in their algorithm decision. The same goes if a cuttlefish or an octopus, like in the first video I showed you. The left eye was seeing sand, which is overall light background which is saying go in a uniform pattern. The right eye saw the calcareous algae, which said go into a mottle pattern. The animal made a decision to go with the mottle pattern. That rock that it was on, I had a video, I was videotaping all that. When the animal first left that rock, it went down on the rock right adjacent to the sand and it went all uniform like the sand. So even within a slight movement, they’re changing their decision. This is a decision-making process. This is not a reflex. This is a very sophisticated analysis of visual information. And that’s really what’s so cool about this system.
– One more question: because the eyes are a different sides, do they have a sense of depth?
– Do they have a what?
– Sense of depth, because, like, they see like one side with just one eye.
– So, the skin papilla, the bumps that they put on, they’re looking with one eye to the background. So that’s not two eyes. With one eye they’re picking up the fine, three-dimensional texture, reproducing it in the skin. I’ve asked a lot of vision scientists how that might work and most people don’t even have a good suggestion. But their pupil, the pupil of a cuttlefish is W shaped. It’s not a round pupil. It’s one of these. So it’s possible that two angles of the W and the other two could somehow get some depth perception information. But that’s just a wild guess. I mean, I have no idea if that can work. So that’s a long-winded answer to say I don’t know. [laughter] So, I’m sorry.
– Your videos are really amazing, and the creatures seem, you know, so friendly and that sort of thing. Are there any dangers involved in this? Have you had any kind of hairy episodes with your dives? [laughter]
– Okay, all you children put your fingers in your ears. [laughter] Well, the cephalopods, the blue-ringed octopus, which is very small, is very poisonous. Luckily, it’s almost impossible to get them to bite you. But they have bitten, like, drunken soldiers and killed them. So it’s a neurotoxin that will paralyze. But they’re not really dangerous. I’ll tell you what is dangerous though, and I have been very frightened once in particular. In Baja California there’s the Humboldt squid. And the Humboldt squid gets almost as large as me. They can get up to about 150 pounds and they’re six feet long and they are the most powerful swimmers in the ocean. And I’ve been underwater with them in the middle of the night in a thousand feet of water. Of course, we’re at the surface. I’ve had those squids come right up to us because we were studying them for a National Geographic thing we were doing. And I’d never felt so defenseless in my life. That squid could have grabbed me and eaten me like an hors d’oeuvre because they’re so powerful. And the worst part is, don’t listen– [laughter] The worst part is they have eight arms and they have a mouth with a beak. And if they ever grab you and eat you, they’re pretty much going to grab you and one bite at a time. You get the picture. So I don’t want to get caught by a big squid. Fortunately, they– No one, no divers have been killed. A few have been grabbed, but not killed. So that time, you know, feeling pretty defenseless. The giant Pacific octopus that’s on Puget Sound and that area, it’s the most docile cephalopod known. So, no, that’s never going to bother anybody. So they really aren’t a problem. Sometimes some squids will bite us, an octopus, and I’ll culture them in the lab. You have to be careful, some people can react to it because what they do is they bite a crab or a fish and they inject a neurotoxin to paralyze it. Then they just eat it one bite at a time. And so they do have these neurotoxins in the salivary gland. Some people who are a little immunosuppressed might react to that a little worse than others. But, basically, it’s not a dangerous animal group to work with. Except those big squids. Yeah?
– When did you get first excited about science, and then how did you come so passionate about cephalopods?
– I’m not passionate about cephalopods. What are you talking about? [laughter] I didn’t plant that question. [laughter] The answer is actually on my web page, believe it or not. So I was kind of a nerd when I was a kid. I got first interested in the moon and in astronomy. That passed pretty quickly. And then I was like an ultra nerd organic gardener when I was 12, 13, and 14. Believe me, our family was sworn to secrecy that Rog didn’t have a garden back there and organic gardening. So I was kind of an earthy character. The thing about marine science came when I was in college, and it was between my sophomore and junior year. I went to Panama to visit my brother who was in the army there. And he wanted to get rid of me one day, like all brothers like to do. So he gave me a mask and snorkel and took me down to the coral reef and said go swim for a while. I swam just here to the wall over there, shallow water, and I swam over this little depression where the coral was and something blew water on my stomach.
And I’ll tell you, I just leaped out of the water. [laughter] I was scared. Really. I was totally shocked. I circled around and came back and looked in this little depression and I didn’t see anything. It was a beautiful coral. And suddenly something moved and it was an octopus, about a two-pound octopus. And it was just there and I thought, how does that little bugger scare the heck out of me, you know? I just watched it. It started moving, changed its pattern, did the camo just like I filmed 40 years later, and I’m still intrigued by that. That was really the thing that really got me. And I eventually went to graduate school, got into diving, and I’m still at it. So, pretty simple story. Just pure fascination. Do all squid have camouflage? Yes. All of them have camouflage. It’s really amazing. Even the ones in the deep water have camouflage. The little ones have camouflage. The big ones have camouflage. And so all sizes of them have camouflage. Not only that, they have camouflage right when they hatch out of the egg. Remember I showed you that one picture of that little squid? That little squid is two millimeters long, and it had camouflage. And they can flash it on and off. So they’re using, camouflage is the number one defense of these soft-bodied animals. We joke a lot but we say the definition of an octopus is a yummy hunk of protein swimming in the ocean and everything’s trying to eat it. And that’s true because they’re right in the middle of the food chain, and the range of predators is enormous. So, you know, believe it or not, an octopus is related to an oyster and a snail. They’re mollusks. The phylum Mollusca. And it looks nothing, you know, those shelled mollusks have their protection on the outside, and the cephalopods got rid of all that shell. They developed this beautiful skin, big brain, and these beautiful eyes. And they do have a shell but it’s all a vestigial shell inside, and it’s, you know, through evolutionary time it’s just shrunk and that shell is internal. So they gave up all that protection on the outside to be smart and witty and change color and that’s basically what they’ve done. So they’ve all got the camouflage.
– We better end there, but thank you, sir. [applause]
Search University Place Episodes
Related Stories from PBS Wisconsin's Blog

Donate to sign up. Activate and sign in to Passport. It's that easy to help PBS Wisconsin serve your community through media that educates, inspires, and entertains.
Make your membership gift today
Only for new users: Activate Passport using your code or email address
Already a member?
Look up my account
Need some help? Go to FAQ or visit PBS Passport Help
Need help accessing PBS Wisconsin anywhere?
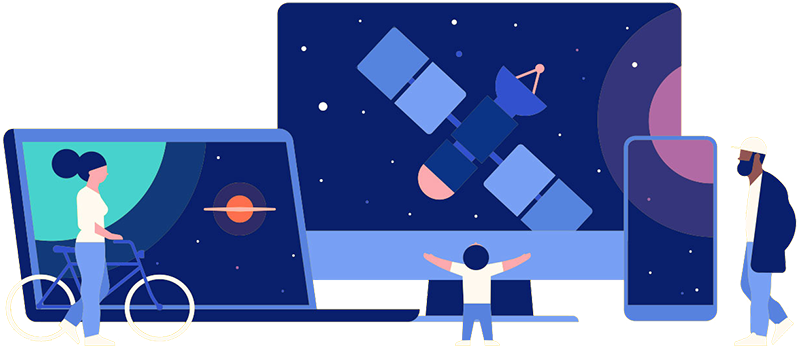
Online Access | Platform & Device Access | Cable or Satellite Access | Over-The-Air Access
Visit Access Guide
Need help accessing PBS Wisconsin anywhere?
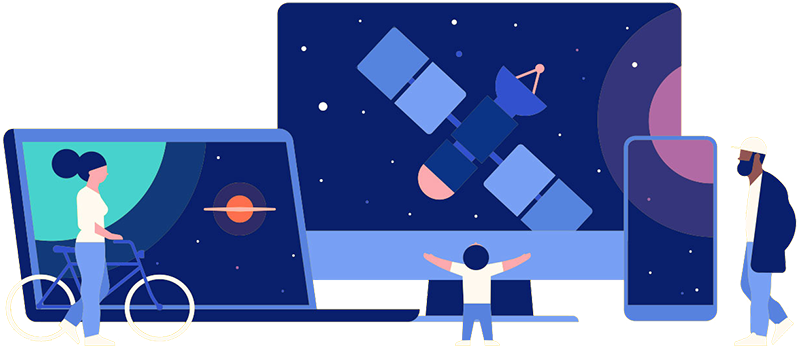
Visit Our
Live TV Access Guide
Online AccessPlatform & Device Access
Cable or Satellite Access
Over-The-Air Access
Visit Access Guide
Follow Us