[Jim Lattis, Director, Space Place, University of Wisconsin-Madison]
Alright, good evening and welcome to a guest presentation at Space Place. Tonight, I’m very pleased to introduce Professor Ellen Zweibel, who is in the U.W. physics and astronomy departments. She’s a plasma physicist, among other things, and won a major award last year, the Maxwell Prize of the American Physical Society. So, I have imposed on her to come talk to us tonight, and her title is “The Fastest Particles in the Universe. ” So, Ellen, thanks for coming.
[applause]
[Ellen Zweibel, Professor, Department of Astronomy, University of Wisconsin-Madison]
Thanks, Jim. I’m not new to speaking, but I am new to television.
[laughter]
So, I apologize in advance for any awkwardness.
The story of the fastest particles in the universe, or cosmic rays, is really a story of curiosity and where it goes, but, where it goes, as I said, but before I launch –
[slide titled, My Wonderful UW Colleagues – not to scale, with photos of all the students and instructors that Ms. Zweibel works with in her field of study]
– into the main part, I just want to acknowledge my – my wonderful colleagues here at the U.W. I couldn’t – I couldn’t work without them. So, everybody from undergrads, Tig Tomush and Cory Cotter, to the most senior faculty, yes, and in between, our graduate students and – and postdocs. And they really do so much to make life a joy.
[new slide titled, “What Ionizes Air?”, with the dates of 1785 when Augustin Coulomb discovered air was partially ionized – with a portrait of Coulomb and 1896 when Marie Curie and Henri Becquerel discovering radioactivity – with a photo of the Curies. Additionally, there is a representation of ionized air as plus symbols and minus symbols and normal air represented as zeros on the lower left of the slide]
So, the story really begins back in the 18th century when Coulomb discovered that air is partially ionized. So, I’ve indicated that schematically here with some little pluses and minuses, and everything else is neutral atoms and molecules. And we expect hot gases to be ionized, but we don’t expect air to be ionized.
So, part of the art to science, and there really is a lot of art in science, is knowing when something is interesting and really worth thinking about or whether it’s just a detail that you can discard. Just kind of an extraneous detail. And, in this case, they made the right decision, that this was really something worth thinking about.
In 1896, radioactivity was discovered, and so this led to the hypothesis that the ionization of the air is caused by high energy particles emitted by radioactive elements in the Earth. So, that was the hypothesis –
[new slide titled, Ionization Source is Cosmic, with the bullet points that in 1911-12 Victor Hess used balloons to show that ionization of the air increases with height and to see if the Sun was the source, he also took measurements during a solar eclipse. Also noted is that Hess won the Nobel Prize for his work in 1936. Additionally, there is a photograph of Hess and his crew in the basket of a hot air balloon and a picture of the Eiffel tower which Hess originally wanted to use for his research but it was not tall enough]
– but then, in 1911-1912, an Austrian physicist, Victor Hess, went up in a balloon to test this idea. So, if the ionization is produced by particles coming from within the Earth’s crust, it should drop off with height. But what he showed instead is that it increased with height. And – and then he thought, “Well, maybe these are particles coming from the Sun.”
[Ellen Zweibel]
So, fortunately there was a solar eclipse. Sometimes you have to wait a long time, although there will be one this summer, and he went up during a solar eclipse, and he still found that the ionization dropped off with height. And so, he concluded that whatever was doing the ionizing, it – it came from the sky; it didn’t come from the ground.
Now, another sort of universal thing in science is that when you think of something, probably someone else has thought of it long before. And, in fact, this idea had been tried previously with the Eiffel Tower. But the Eiffel Tower is not tall enough to show this effect. You really have to go up several kilometers. And Victor Hess got the Nobel Prize in physics in 1936 for this work.
[slide titled, Cosmic Rays – Radiation or Charged Particles?”, with the note that studies in the 1920s showed more cosmic rays come from the West making them positively charged. Additionally, there is an illustration of the Earth and its magnetic fields]
So, the name that he gave this ionizing radiation is one of those, sort of, descriptive names that you give something when you don’t really know what it is, like adding “-itis” to a body part.
[laughter]
So, okay, cosmic ray, and so the question is – is this high energy light or is it charged particles?
[Ellen Zweibel]
And to answer this question, studies beginning in the 1920s showed that cosmic rays arrive preferentially from the west. And so, the idea is something like this. Here’s the Earth. And here is its magnetic field –
[return to the Cosmic Rays slide]
– like the magnetic field of a bar magnet. And if we kind of zoom in close up to these field lines, charged particles are gyrating around them in kind of helical orbits. And the size of the orbit depends on the energy of the particle, and the direction of circulation depends on whether the particle is positively or negatively charged. Now, I put this all on one slide –
[new slide titled, This Did Not Come Easily, indicating that from 1930-1940 Compton lead a world-wide study of the geographical variation of the intensity of cosmic rays ultimately confirming the observation of J. Clay in 1927 of the influence of latitude on cosmic ray intensity. Additionally, this slide has a photo of Mount Evans and a photo of a ship rounding Cape Horn and a New York Times headline – Millikan retorts hotly to Compton in Cosmic Ray clash]
– but it did not come easily. There was a 10-year effort on the part of Arthur Holly Compton really to go all over the world and to – to work at sea level, to work on mountaintops. He rounded Cape Horn something like five times. He climbed Mount Evans in Colorado. Two of his research assistants were – were killed on Mount McKinley. And he – but he – he established that there was a geographic variation –
[Ellen Zweibel]
– in the arrival of cosmic rays that correlated with the geomagnetic field. Now, you’re going to see the name Millikan again. So, the New York Times, one of their front-page headlines, December 31, 1932, –
[return to the “This Did Not Come Easily” slide]
– “Millikan retorts hotly to Compton in cosmic ray clash.” So, these were two of the greatest physicists of the era, and they disagreed over whether cosmic rays were charged particles or photons.
[new slide titled, Energy Here Means Einsteinian Energy, featuring a photo of Albert Einstein and a bulleted list indicating that one applies a force on a particle to increase its mass, the speed will level off as it approaches the speed of light – c, its effective mass will increase instead and there is no limit on its energy]
Now, I want to take a little detour and – and emphasize that when I talk about the energy of a particle, I mean Einstein’s definition of energy. And, according to Einstein, if you – well, according to Newton or just us, if you apply a force on a particle, you increase its speed. But what Einstein showed is that the speed will not increase indefinitely. It’ll level off at the speed of light. Than you cannot get this particle to go faster than the speed of light. But you’re putting energy into it, and so instead its effective mass will increase.
[Ellen Zweibel]
And the energy can rise without limit. And to kind of illustrate what this means, so let’s say we take one proton, which is – it turns out that’s what most cosmic rays are, and if that proton has an Einsteinian effective mass of 10 protons, it’s going at .995 of the speed of light, and if it has an effective mass of 100 protons –
[slide titled, Speed vs. Effective Mass, noting that if the effective mass of a proton is 10 protons, it is going at .995 of the speed of light, at 100 protons it is at .9995 of the speed of light and at 1000 proton it is at .999999 the speed of light. Also noted is that an ultra-high energy cosmic rays are at .9999999999999999999999995 of the speed of light]
.99995 c., and this is the last one I’m going to read, 1,000 protons, .99999 c. The highest energy cosmic rays are going this close to the speed of light. There are, I think, 21 nines here. But they’re still going less than the speed of light. That’s the law.
[new slide titled, Large Hadron Collider at C.E.R.N., with the bullet points that the effective mass of fastest protons made at C.E.R.N. is about 6500 times their natural mass and their speed is about .99999999c]
So, how does this compare to the large – to the most powerful particle –
[Ellen Zweibel]
– accelerators on Earth? So, right now that would be the Large Hadron Collider at C.E.R.N., where the Higgs boson was discovered.
[return to the Large Hadron Collider slide]
And the – the Large Hadron Collider can accelerator protons up to about 6500 effective proton masses, and that corresponds to a speed of about so. So, not as many nines, not as high an effective mass as the highest energy –
[new slide titled, Discovery of New Particles, with a list of the following new particles. Mu-leptons (muons0, pi-mesons (pions), K-mesons (kaons), Lambda particle, neutrinos with mass. Also noted is that these all require high energy measurements which were first obtained with cosmic rays]
– cosmic rays. And that’s why a lot of particle physics was really done first on cosmic rays. So, here’s a list of all the exotic particles that were first discovered in the cosmic rays, including that neutrinos, the ghost particles, have a mass.
[new slide titled, Tennis ball vs. Cosmic Ray, featuring a photo of Serena Williams hitting a tennis serve and a photo of the Large Hadron Collider at C.E.R.N. and noting that the fastest recorded tennis serve has four times more energy than the highest energy cosmic ray ever detected. Also noted is the ball has 3.6 X ten to the 25th atoms but the cosmic ray is going 4.3 million times faster. Also mentioned at the bottom of the slide is that the fastest tennis serve is 10 million time more energetic than the particles acerated at C.E.R.N.]
But to kind of bring this down to Earth, it turns out that the highest energy cosmic ray ever detected has an energy about one-quarter of the fastest recorded serve. So, here’s Serena Williams killing the ball. So, the tennis ball is more energetic than that cosmic ray, but that tennis ball has 3. 6 times 10 to 25 zeros, and the cosmic ray is going 4. 3 million times faster. So, morally, maybe it’s sort of a tie.
[new slide titled, Radiation Belts, featuring an illustration of the Earth and its radiation/magnetic belts and noting that trapped low energy particles have small magnetic orbits while free high energy particles have large magnetic orbits]
So, to get back to what this means for how we detect cosmic rays on Earth, the Earth has these radiation belts, which are due to the magnetic field, and low energy particles with small magnetic orbits are trapped in the radiation belts but high energy particles with large magnetic orbits, like cosmic rays, can just come in and they’re kind of deflected and they arrive preferentially from the west. So, that explains –
[Ellen Zweibel]
– what Clay and then Compton and so many others later saw.
Now, the highest energy cosmic rays, the sort of tennis ball equivalent cosmic rays –
[slide titled, Ultra-High Energy Cosmic Ray (U.H.E.C.R.) Orbit, with an illustration of the Milky Way galaxy and a yellow line representing a cosmic ray coming from outside the galaxy and flowing through the galaxy to return to outside the galaxy]
– and I’m sometimes going to abbreviate ultrahigh energy cosmic ray or ultrahigh energy cosmic ray as U.H.E.C.R. So, astronomers love acronyms, and instead of saying this, sometimes I’ll say this. And so, this is what our galaxy probably looks like. Of course, we’re deep inside it, so we can’t verify that. But these – but the orbit of a cosmic ray, ultrahigh energy cosmic ray in the magnetic field of the galaxy looks something like this. Whereas the cosmic rays that we detect mostly in Earth have an orbit about the size of the Earth/sun distance.
[new slide titled, How Common are U.H.E.C.R.?, featuring an illustration of a cosmic ray hitting the Earth with the bullet points that about 40,000 strike the U.S.A. every year, which translates to about one person every 2.5 years and also noting that a few people die every year from being hit by baseballs. Also noted is that high energy cosmic rays break up in the atmosphere, reducing their threat.]
So, how common are these U.H.E.C.R.? Well, they’re not common but they’re not terribly rare. About 40,000 strike our country every year. And if you estimate how much area our population takes up, that would translate to about one person every two-and-a-half years. Now, this is actually a – a smaller number than the number of people who are killed every year by being hit by baseballs. That’s a couple of people every year. But you’ve never heard of anyone being killed by a single cosmic ray, and that’s because these high energy cosmic rays break up in the atmosphere and they create these showers of high energy particles called extensive air showers.
[new slide titled, Adding It All Up, with the bullet points that one in a billion particles in interstellar space is a cosmic ray, moving at near the speed of light, the energy in cosmic rays equals the energy in the rest of the gas and that if it happened in the room the temperature would drop by half and one billionth of the molecules would become lethal]
There’s another way that we can think about it. So, these most –
[Ellen Zweibel]
– these highest energy cosmic rays are very rare. The lower energy ones, still moving at nearly the speed of light, are more common, and that adds up to about one in a billion particles in interstellar space. But the energy in that one billionth is equal to the energy in the rest of the gas. And if that happened in this room, if we could make that happen in this room, the temperature would drop by one half. So, from whatever it is to 35 degrees or something like this. And one billionth of the molecules would become absolutely lethal. So, we don’t expect this to happen in rooms on Earth, but somehow it happens in interstellar space, and part of our job is to explain that.
[slide titled, Origin of Cosmic Rays [1932 New York Times], featuring a newspaper article clipping with the headline that Cosmic Rays Only Thing Immortal]
So, let’s get back to the origin of cosmic rays. So, here’s Millikan again, and here’s another headline in the New York Times – “Cosmic Rays are the Only Thing Immortal.” This is back in 1932. “Neither stars nor worlds, sunlight or heavens can science admit to be eternal. Only one thing known to science can be called immortal – the cosmic rays investigated, amongst others, by the famous California physicist Dr. R.A. Millikan. These rays may even be relics of days before there existed any universe as we know it now.”
But there was another idea that came along a couple –
[new slide tilted, An Alternative Theory, featuring a photo of the Crab Nebula and photos of Walter Baade and Fritz Zwicky and noting that in 1934 Baade and Zwicky proposed that cosmic rays originate in supernovae which occur about every 50 years in the Milky Way]
– of years later, and this was due to two other Caltech astronomers, Walter Baade and Fritz Zwicky, and they proposed that cosmic rays originated in supernovae. So, here’s an image of a young supernova remnant. This is the Crab Nebula, seen actually many forms of light are put together. So, there’s about one supernova every 50 years in the Milky Way, but we see them much less frequently than that because most of them are obscured by interstellar dust. And at the time they made their suggestion, Baade and Zwicky had absolutely no real physical basis –
[Ellen Zweibel]
– for saying so. It was really just a speculation based on intuition. Supernovae have a lot of energy. Cosmic rays have a lot of energy. Maybe somehow, they’re connected.
But Millikan’s hypothesis was – was tested in 1948 by Phyllis Freier –
[slide titled, Were Cosmic Rays Born in the Big Bang?, featuring a photo of the 1932 New York Times clipping from the previous slide along with a photo of Phyllis Freier and the periodic table. Noted is that in 1948 Ms. Freier then a graduate student showed that cosmic rays contain many different kinds of atoms, while only Hydrogen, Helium and some Lithium were created in the Big Bang]
– who was then a graduate student, one of the real pioneer women in science. And she showed that cosmic rays contained many different kinds of atoms: hydrogen, helium, carbon, nitrogen, oxygen, iron, but the only elements that were created in the Big Bang, so by 1948 people already knew about the Big Bang, are hydrogen, helium, and a little bit of lithium. So, that was pretty definitive proof that cosmic rays have not been around since the beginning of the universe. They’ve been created much more recently.
[new slide titled, Golden Age of Cosmic Ray Discoveries, featuring photos of Marietta Blau, Stephen McGuire and C. Jake Waddington along with two photos of tracks made by high energy particles in plastic]
And so, beginning in the late ’40s, 1950s, 1960s, there was really kind of this golden age of cosmic ray discoveries. And I’m showing here two different tracks of high energy particles made in plastic, and here’s another one, and here are just a few of the pioneers and they contributed in so many different ways. Some to the technology, some to the theory, some of them by climbing mountains. It was a huge human enterprise.
[new slide titled, High Elevations and Balloons Were Important, showing two photos, one of the Sulphur Mountain site and one of a balloon launch near Fort Churchill in Manitoba, Canada]
Some of the other tools were high altitude balloons. The first research job I was offered in college was with a group that launched these balloons, not from Manitoba but from – but from Hudson Bay, and I tried to picture myself driving around on the frozen tundra in a – in a giant truck. And then I took a job with a radio astronomer.
[laughter]
And it worked out, worked out very well.
[new slide titled, Gamma Rays – A New Window on Cosmic Rays, featuring 3 images of a supernova remnant as seen on scientific equipment and noting that Baade and Zwicky were partly correct]
A couple of decades ago, we developed, really, a new window on cosmic rays –
[Ellen Zweibel]
– with the ability to detect cosmic gamma rays. And so, it turns out – so, this is a gamma ray –
[return to the Gamma Rays slide]
– view of a supernova remnant, and it turns out that supernovae remnants are just lit up in gamma rays. Here’s the Large Magellanic Cloud, the small dwarf galaxy that you can only see from the southern hemisphere, and here’s a region where a lot of stars are forming, and supernovae are going off and it’s all lit up in gamma rays too. And what this means is that 70-80 years after Baade and Zwicky made their intuitive suggestion, we’ve shown, really, that they’re at least partly right. Cosmic rays do have something to do with supernovae remnants, the origin of cosmic rays.
[new slide titled, Cerenkov Radiation – A Sonic Boom of Light, featuring two photos, one of a jet breaking the sound barrier and one of a cone shaped illustration of the principle of Cerenkov Radiation showing a similar effect. Also noted is that light in water is slower than light in a vacuum, so cosmic rays traveling through water are faster than light]
So, another way in which we can detect cosmic rays relies on a – a, sort of, sonic boom phenomenon in cosmic ray, in – in light that – that we call Cerenkov radiation. So, here’s – here’s an airplane breaking the sound barrier, a very sleek-looking airplane. And what Cerenkov radiation is about is that the speed of light in water is slower than the speed of light in the vacuum. And so, if a cosmic ray goes tearing through a tank of water, it’s not going faster than light in a vacuum but it’s going faster than light in water. And so, the radiation that it emits can’t spread out –
[Ellen Zweibel]
– in all directions from it the way it can for a normal particle. It gets concentrated in this cone. And this is called Cerenkov Radiation –
[return to the Cerenkov Radiation slide]
– and it’s similar to a sonic boom.
[slide titled, HAWC Cerenkov Telescope Near Puebla, Mexico, with a photograph of the telescope site and noting that the U.W. is a partner in this site]
And so, the U.W. is a partner in a cos – in a high energy cosmic ray detector called H.A.W.C. It’s a Cerenkov telescope. It’s being built in partnership with many institutions, primarily Mexico. So, it’s near Puebla. And everything you see here is a giant water tank. And when cosmic rays break up in the atmosphere above H.A.W.C., their particles come, sort of, streaming down and they set off Cerenkov Radiation in all these tanks. And by looking at the timing when the Cerenkov Radiation is seen in the tanks, you can estimate how much energy the particle had and where it came from. And I’ll be showing you some results from that –
[new slide titled, Theory of Particle Behavior in Magnetic Fields, featuring photos of researchers Miriam Forman, Randy Jokipii, Tova Yoast-Hull (at a blackboard) and graphical representation of their research plotted in a cube]
– a little bit later on.
There’s also theory. That’s what I do. Again, many, many players. This is a former graduate student of mine, Tova Yoast-Hull. And I just love this picture because look at this blackboard and she looks so exhausted.
[laughter]
But she did get her PhD, and she’s now a postdoc at the Canadian Institute for Theoretical Astrophysics in Canada. And this is the kind of thing we calculate.
[new slide titled, Unique Facilities – Pierre Auger Observatory, with an artist rendition of the observatory detecting cosmic rays that appear like lightning striking detectors on the ground]
So, I’ve mentioned these unique facilities. This is the largest H.A.W.C.-like telescope, Cerenkov telescope. This is called the Pierre Auger Observatory. It’s 1660 water tanks spread out over this large area of Western Argentina. And this is an artist’s conception of particles from an air shower as a cosmic ray breaks up, setting off these different water tanks across 3,000 square kilometers. So, this is the largest cosmic ray detector ever built.
[new slide titled, And Not So Unique – D.E.C.O. – Distributed Electronic Cosmic-ray Observatory – A Citizen Science Project, featuring a photo of U.W. scientist Justin Vandenbroucke and noting that your cell phone camera can detect elementary particles produced by cosmic ray showers using 2 apps that can be downloaded and whose data is automatically uploaded and sent for analysis]
But, in addition to these unique facilities, there are some not unique facilities. You can turn your cell phone into a cosmic ray detector. So, my colleague in – in the physics department, Justin Vandenbroucke, developed this project called D.E.C.O., Distributed Electronic Cosmic Ray Observatory. Your cell phone camera, so you have to leave you cell phone on, and it can detect the particles produced by air showers. And if you download two apps, the data will be automatically uploaded and sent for analysis, and you can become part of a high energy cosmic ray detection project. All you need is a smartphone, which I ironically do not have.
[laughter]
Okay.
[new slide titled, Origin of the Highest Energy Cosmic Rays]
So, now let’s talk about where the highest energy cosmic rays come from. And this is going to be – we’re not going to – we may not get to the answer –
[Ellen Zweibel]
– but we’ll go through kind of a process of elimination. So, here’s a view again of what our galaxy probably looks like.
[slide titled, What Our Galaxy Probably Looks Like, featuring a photo of the spiral Andromeda Galaxy]
So, this is, of course, not our galaxy, but it’s the nearest galaxy similar to ours, the great nebula in Andromeda. So, a spiral galaxy.
[new slide titled, How We See The Galaxy From Inside It, featuring a N.A.S.A. photo of a blue oval with a large white line across the center, the galactic plane, and around the white line reddish-orange and green highlighting, local fluff]
This is how we see the galaxy from where we are, namely inside it. So, the galactic plane, so most of the matter, the visible matter in the galaxy, is in a flat disc. And so, the galactic plane, which we know as the Milky Way as times that we can – at times that we can see it, is the brightest structure in the sky. Now, why isn’t the Milky Way bright? It’s not extremely bright because most of the starlight is absorbed by interstellar dust. And that is what gives the kind of patchy, lumpy patches of black. And when we see, kind of, irregularities in the plane, that – they turn out mostly to be associated with nearby structures. So, this is something called the North Polar Spur. It’s related, actually, to supernovae remnants. So, this is how we see our galaxy.
[new slide titled, “Do Ultra High Energy Cosmic Rays (U.H.E.C.R.) Come From Within the Milky Way?”, featuring that same N.A.S.A. photo from the previous slide and a new image with the galaxy on an oval plot by R. Nemmen showing known features. Additionally, it is noted that if so, U.H.E.C.R. should show patterns similar to other emission, but they don’t, unless -]
So, okay, let’s hypothesize that ultrahigh energy cosmic rays come from within the Milky Way. So, there aren’t very many of them. You can actually plot the direction of each one, determined in the way that I explained using many, many water tanks and Cerenkov Radiation. So, this is their distribution on the sky. These red dots. And these blue objects are some, sort of, famous galaxies that we’ll talk about. And so, if ultrahigh energy cosmic rays come from within the Milky Way, you might think, “Well, then they should have a distribution similar to the light that we see from the Milky Way.” It should all be concentrated in this plane, but it’s not.
[Ellen Zweibel]
So, that is maybe a clue that cosmic rays, that these ultrahigh energy cosmic rays don’t come from within the Milky Way.
But there’s a possible way out, and that is suppose if they actually come from very close to the sun. So, now you have to put up with my cartooning.
[slide titled, Could They Come From Very Nearby, featuring an illustration with two uneven purple lines and within those lines lighter purple squiggles representing the matter of the galaxy and dots representing sources of unknown nature (cosmic rays) and a larger dot in the middle that represents the Sun]
This is kind of a little piece of the disc of the galaxy in which the sun is embedded, and the galactic center is – is this way. And this kind of purple scrolling is just all the matter in the disc of the galaxy. And these dots here represent the source of cosmic rays, and they’re, you know, of an unknown nature. We don’t know what they are. But if they’re close enough, if they’re all concentrated close to the sun, kind of, like bees in a nest, then we would see them coming from pretty much the same direction everywhere.
So, that would work, but we’ve never identified what these nearby objects could be that are capable of accelerating particles to such high energies. So, let’s put this idea on – on the back burner.
[new slide titled, Do U.H.E.C.R. Come From Galaxies with Active, Supermassive Black Holes, featuring the R. Nemmen galactic plot and a photo of the Centaurus A galaxy which is purported to have a large Black Hole that is shooting off large jets of plasma]
So, another possibility is that U.H.E.C.R. come from galaxies with supermassive black holes. And so, here’s an image, and this is a composite, not an artist’s conception but a composite of emission of light at many different wavelengths. So, here is the galaxy Centaurus A, and at its center is a supermassive Black Hole probably about a hundred million solar masses. And activity in the vicinity of the Black Hole has launched these enormous jets of hot plasma that are thousands of light years, hundreds of thousands of light years in size. And so, could – so, now this is a sort of “Baade and Zwicky”-type argument. Energetic thing, energetic thing, could they somehow be related? Well, we don’t see any concentration of ultrahigh energy cosmic rays coming from objects like Centaurus A so we can’t establish any correlation. That’s a suggestion maybe that’s not where they come from.
[new slide titled, Cosmic Rays Collide With Light Waves, featuring three photos of the scientists, Greisen, Kuzmin and Zatsepin and an illustration from their research noting that the highest energy cosmic rays lose the most energy so that they must be produced within 150 million light years]
Can we say anything about where they come from? Well, it turns out that we can. So, in the 1960s, three physicists simultaneously, roughly, Greisen, Kuzmin, Zatsepin, thought about what would happen if cosmic rays collided with light waves. And the light waves would most probably be the cosmic microwave background radiation left over from the Big Bang.
And so, a very high energy. So, you know, we don’t really think of colliding with a light wave as doing much damage. But that’s because we’re not a cosmic ray.
[Ellen Zweibel]
So, just as when a jet plane hits a chicken, it can do a tremendous amount of damage, when an ultrahigh energy cosmic ray hits a very feeble little microwave photon, it can break up. And they estimated that given the amount of radiation in the universe, the highest energy cosmic rays must be produced within 150 million light years. Now, that may not sound like a very small range to you, but it’s a tiny, tiny fraction of the volume of the known universe. So, we can at least – we can at least say this much – that the ultrahigh energy cosmic rays come from within 150 million light years.
Okay.
So, let’s – let’s –
[slide titled, Pulsars, featuring two photos one of the Crab nebula and one of a Crab pulsar along with a color illustration of the anatomy of a pulsar star noting that they are rapidly spinning neutron stars with strong magnetic fields, formed by supernova explosions]
– look around the universe and say, “Where are there objects with high energy strong magnetic fields, strong electric fields?” And so, one thing that comes to mind are pulsars. So, here’s the same image of the Crab Nebula that we looked at a few minutes ago. And here is zeroing in on the center of the Crab Nebula is this X-ray – this X-ray radiation that’s produced by the Crab pulsar. And so, an artist’s conception of a pulsar would be a neutron star. So, a neutron star is an incredibly compact ball of mostly neutrons. So, up to three times –
[Ellen Zweibel]
– the mass of the sun but concentrated in a sphere maybe 10 kilometers in radius. So, really the size of Madison, that’s a neutron star. And they have super strong gravity and super strong magnetic fields, and they spin on their axis hundreds or even up to a thousand times a minute. So, they’re among the most extreme objects that we know in nature.
And there’s a class –
[slide titled, Magnetars – Pulsars with Even Larger Magnetic Fields, featuring an artist’s rendition of a magnetar noting that the magnetic field is so strong that the crust cracks along with photos of the scientist Professor Chris Thompson from the University of Toronto and a high school student named Meghan Gialluca from Hollis Brookline High School in New Hampshire]
– of pulsars that are called magnetars. And their magnetic fields are even larger. Their magnetic fields are so strong, so this is again an artist’s conception, they’re strong enough to crack the crust of this neutron star. And so, a theory developed by Professor Chris Thompson at the University of Toronto is that these magnetars, the rotation of these magnetars with their strong magnetic fields, is what produces the ultrahigh energy cosmic rays. And here is a high school senior, Meghan Gialluca from Hollis Brookline High School in New Hampshire, and she just missed by this much being the Young Astronomer of the Year. She was beaten out by – by two guys who worked in radio astronomy. But she made a very nice study of glitches and anti-glitches. So, a glitch is a little change in the rotation rate of these magnetars. So, again, citizen science. Anyone can contribute.
[new slide titled, Advantages of the Magnetar Model, with the bullet points that they have super strong magnetic and electric fields to accelerate particles to high energies, they are formed rarely in spiral galaxies but that there are enough galaxies within 150 million light years to supply the U.H.E.C.R. that we see and that supermassive, accreting Black Holes are not required]
So, the magnetar model has some advantages. There are super strong magnetic and electric fields that accelerate particles to high energies. So, they’re formed rarely in any individual galaxy, but there are enough galaxies, probably doesn’t surprise you, within 150 million light years to supply the U.H.E.C.R. that we see. And the galaxies don’t have to be special in any way.
[Ellen Zweibel]
They don’t have to have an active nucleus, a nucleus with a supermassive black hole that’s actively accreting. Any galaxy in which stars are forming can make these magnetars. So, this, except for how the particles actually acquire their energy, this model really fits what we know.
So, we can go a little further. So, here’s another international –
[slide titled, The Neutrino Connection, featuring a photo of the outside IceCube Observatory at the South Pole and an artist’s conception of how the observatory works]
– project in which Wisconsin has an important role. In fact, we’re leading it. This is the IceCube Observatory at the South Pole. And these are, this is again an artist’s conception of deep holes that are drilled in the Antarctic ice that are lit up when high-energy particles come through. So, the IceCube Observatory has – has seen a lot. It’s detected a lot of cosmic rays, and it’s detected –
[new slide titled, High Energy Neutrino Sky, with an oval graph of results from the IceCube Observatory and posing the question – Are U.H.E.C.R. and high energy neutrino sources the same? And noting that so far they share the same “nots”]
– high energy neutrinos. And this is what the sky looks like in high energy neutrinos. So, purple is a neutrino. There are so few neutrons that they can be given individual numbers, although they haven’t been given names. Are the sources of these neutrinos the same as the sources of these U.H.E.C.R.? Well, they share the same list of “nots.” They’re not concentrated to the galactic plane. They’re not preferentially coming from active galaxies. And for now, that’s all that we can say.
[new slide titled, Bringing It All Back Home, featuring an artist’s rendition of U.H.E.C.R. falling to the Earth next to a photo of a lightning storm. Also noted is that “secondary” particles created as cosmic rays break up in the atmosphere can trigger lightning and modify the atmosphere but not by very much]
So, the study of cosmic rays began with the observation that air was ionized. So, if we, kind of, bring it all back home and say, “Okay, there are these high energy particles that are impinging on the atmosphere and breaking up into this cascade of other energetic particles. Do they do anything? Do they do anything noticeable to the atmosphere of the Earth?” And there’s pretty good evidence that they can trigger lightning strikes by kind of pre-ionizing the air. So, the same ionization of the air that Culham discovered back in 1785 is sort of providing a conduit for lightning strikes.
And here’s a dramatic lightning – this is the best picture I found. This is actually a little town in Belgium. But lightning is the same all over the Earth. And so, a fair question would be – Do cosmic rays affect the chemistry of the atmosphere? Do they affect the climate? And this has been thoroughly studied because cosmic rays are always there, and the answer seems to be a little bit but – but not very much. So, they are primarily a cosmic phenomenon, and that’s, I think, where they’re going to stay.
[new slide titled, Summary, with and artist’s rendition of cosmic rays striking the Earth next to the bullet points that cosmic rays are an extreme form of matter, associated with some of the most energetic events in the Universe, a vast web of human effort in pure and applied science has allowed us to understand them and that there is much more work to be done]
So, what’s the summary? So, cosmic rays are an extreme form of matter. The highest energy particles that we’ve ever detected.
[Ellen Zweibel]
Much higher energy than we can make in any accelerator on Earth. And there’s no single person who made a decisive contribution to the study of cosmic rays. It’s just been this vast web of human effort. And it’s been both pure science and applied science. The creation of new plastics. The creation of counters to measure to faint Cerenkov Radiation. Contributions from all sides that have allowed us to understand them as – as well as we do.
[return to the Summary slide]
But there’s so much more work to be done.
[slide titled, The End, with Professor Zweibel’s contact info]
So, that is the end.
[Ellen Zweibel]
This is how you can find me. And thank you.
[applause]
Search University Place Episodes
Related Stories from PBS Wisconsin's Blog

Donate to sign up. Activate and sign in to Passport. It's that easy to help PBS Wisconsin serve your community through media that educates, inspires, and entertains.
Make your membership gift today
Only for new users: Activate Passport using your code or email address
Already a member?
Look up my account
Need some help? Go to FAQ or visit PBS Passport Help
Need help accessing PBS Wisconsin anywhere?
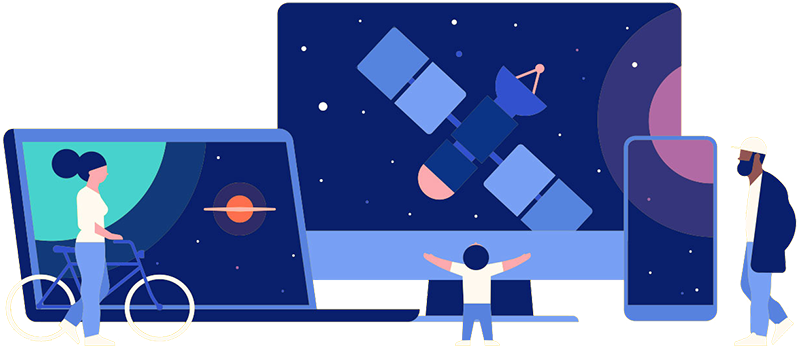
Online Access | Platform & Device Access | Cable or Satellite Access | Over-The-Air Access
Visit Access Guide
Need help accessing PBS Wisconsin anywhere?
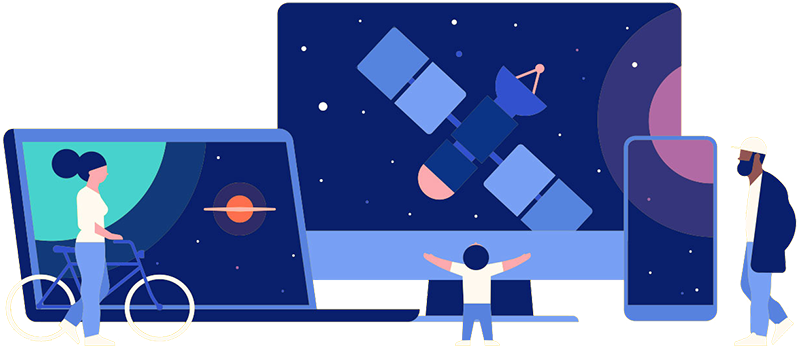
Visit Our
Live TV Access Guide
Online AccessPlatform & Device Access
Cable or Satellite Access
Over-The-Air Access
Visit Access Guide
Follow Us