Welcome everyone to Wednesday Nite @ the Lab, I’m Tom Zinnen, I work here at the UW-Madison Biotechnology Center. I also work for UW-Extension Cooperative Extension. And on behalf of those folks and are other co-organizers, Wisconsin Public Television, Wisconsin Alumni Association, and the UW-Madison Science Alliance. Thanks again for coming to Wednesday Nite @ the Lab. We do this every Wednesday night 50 times a year. Tonight is my pleasure to introduce to you, Tom Givnish. And he’s from the Department of Botany. He was born in Philadelphia, and then he went to La Salle College High School, which is a stacked noun, which I love. So that was the high school for La Salle College. Absolutely. Then he went to Princeton. How far is Princeton from Philadelphia?
– 50 miles.
– (whistles) Close. And at Princeton University, he studied math undergrad and stayed on there at Princeton to get his PhD in Biology. And then he went to teach at Harvard, and in 1985, he came to UW-Madison. Tonight he’s going to talk about one of the cool things ever. Plants that eat animals: Carnivorous plants. Is that fair enough? He’s going to talk about, “The Green Empire Strikes Back: The Ecology and Evolution of Carnivorous Plants.” Please join me welcoming Tom Givnish back to Wednesday Nite @ the Lab. (audience applauding)
– Thanks so much.
– I appreciate it.
– Thanks for those very kind words, Tom, I hope you’ll find it a very fun topic we’ll be discussing tonight. As you most plant species are eaten, in whole or part, by animals that act as leaf eaters, or pollinators or seed dispersers, but carnivorous plants have turned the tables, and consume animals as prey. Charles Darwin was the first to recognize, and ultimately strongly defend, this remarkable fact, beginning in 1860. He was walking through an open, acid, heath on wet, sandy peat in Southern England, when he was surprised to see the great number of insects caught by the glistening leaves of the common sundew, which is a low-growing herb. The sticky droplets secreted by the red glandular hairs, or tentacles on each leaf, glitter in the sun like dew, which gives sundews their name, and sundews entrap insects that range from small flies to larger butterflies and damselflies. Given the large number of sundews present in any given site, Darwin saw that, quote, “The number of insects thus annually slaughtered must be prodigious.” (audience laughing)
Close quote. Now Darwin realized that the sticky hairs of many plants, like petunias, kill insects without the plants receiving any benefit other than protection against being eaten by those insects. But he was able to show, through a series of careful observations and brilliant experiments, culminating in his classic 1875 book on “Insectivorous Plants,” that sundews and a few other carnivores differ in having extraordinary adaptations to entrap and digest insects. His son, Francis, soon thereafter demonstrated that sundews that caught prey grew faster and set more seeds than those that had not. Given how little nitrate is available to roots in acid, wet peat, Darwin argued that carnivorous plants should gain an advantage on those soils by augmenting their nitrogen supply via insect capture. Darwin demonstrated three crucial facts about sundews. First, their tentacles are exquisitely sensitive to pressure, and minute doses of nitrogenous liquids. Secondly, the tentacles, and in some species, the leaves themselves, you can see here, move quickly, over seconds to minutes, toward entrapped insects, further ensnaring them. Finally, the tentacles secrete fluids that digest nitrogenous substances, and then later resorb them. Today, we can visualize these extraordinary movements, and secretions and resorptions through time-lapse photography, enzyme assays, and… Microcopy to trace the movement of labeled proteins or amino acids into plant tissues and cell compartments. But Darwin didn’t have the luxury of access to such sophisticated approaches, and instead had to rely on his own drawings and a stopwatch, the fate of minute pieces of cooked egg white and meat exposed to secretions, and the microscopic examination of tentacle cells as they resorbed the digest.
Now through this, Darwin is able to document or infer the mechanism of prey capture in seven genera of carnivorous plants. These included the sundews of the genus Drosera, with 200 species worldwide. I was just actually in the great center of diversity for this genus in Southwest Australia that has about 2/3 of all the species in the world. These sundews bear active sticky traps with tentacles, and often leaves that move over seconds to minutes. And then also the closely related single species of Venus flytrap, Dionaea, restricted to a small area in the coastal plain of the Carolinas. These bear snap-trap leaves with two lobes bearing nectaries, and as you can see here, trigger hairs nearby. These lobes will close in less than a second when an insect touches at least two of the trigger hairs in quick succession. The widely spaced teeth or cilia allow small insects to escape while, as you can see, retaining larger prey as the lobes clamp shut. The closed leaf lobes form a tight chamber in which the prey is digested. And the leaf re-opens after digestion is completed, resetting the trap. Recent studies have shown that the Venus flytrap actually counts the electrical spikes caused by touching individual trigger hairs, with rapid trap closure driven by voltage-regulated ion channels, for sodium and potassium and calcium, that begin transmitting electrical signals within tens of milliseconds after stimulation.
These cause the leaf lobes to clamp shut, and increasingly so, as the prey struggles within the trap touching the trigger hairs and it does through a welter of processes that we still don’t fully understood but almost surely involve water movement, and rapid cell wall growth, believe it or not, and a bi-stable lobe geometry will flip from concave to convex very rapidly. Electromechanical stimuli also release jasmonate, which is a hormone involved in responses to insect attack used by most other plants, but in Venus flytrap, it triggers the release of digestive enzymes over tens of minutes to days. While Darwin did not understand the precise mechanism by which Drosera and Dionaea rapidly close their traps, he was able to show that some kind of impulse traveled through their tissues. Understandably, he became quite enthused with all these discoveries, he considered the Venus flytrap to be the, quote unquote, “the most wonderful plant in the world” and he even wrote to a colleague at the time that quote: “At the present moment, I am more interested in Drosera than the origin of all the species in the world!” (audience applauds) Coming in 1860, just after the origin of species that’s saying a lot. By the way, by comparison Li-nay-ass, responding to some very early reports of possible carnivory that he received in the 18th century said it’s impossible that if plants were to eat animals, it would contravene the will of God, (audience laughs) and he dismissed it out of hand. Now the waterwheel plant, Aldrovanda, has a patchy distribution worldwide and essentially is a miniature aquatic version of Dionaea, with snap traps that close very rapidly underwater, and capture insect larvae, crustaceans, and even small fish.
Butterworts in the genus Pinguicula, including about 100 species in the northern hemisphere, have yellow, glandular leaves and inrolled leaf margins that serve as active sticky traps to catch small flies. The single species of dewy pine, Drosophyllum, from the western Mediterranean, has glandular leaves, but these glands and leaves do not move and so they form passive sticky traps. By the way, dewy pines are relatives of sundews, they are not pines. Pines are coniferous, not carnivorous. (audience applauds) All right. Corkscrew plants. Many of you I’m sure have never heard of these, of the genus Genlisea, 30 species of butterwort relatives from tropical Africa and the Americas, bear strange forked roots. Actually they are leaves, in water or wet sand, and each of them bears, as you can see, spiral grooves with the hairs all aligned up toward where the roots join and there’s a mouth that then leads to a passage way through a series of cones arranged more or less like a lobster pot or an eel trap and apparently ensnares protozoa, and cyanobacteria, nematodes, micro-crustaceans, especially copepods, mites, and unicellular algae. The prey are sort of like Shmoos. I don’t know how many people remember the old Al Capp cartoons involving L’il Abner, but the Shmoos threw themselves into the frying pan and these guys swing themselves into the stomach of the plant.
Finally, bladderworts in the genus Utricularia, with 250 species worldwide, have unique, underwater suction traps. We now know that these bladder-like organs have four-sided, or four-armed, pardon me, glands, so that plant really has four arms that are inside that pump water out and create a partial vacuum inside, and then a trap door, shown here, which is perched on a raised threshold. Now if a small aquatic animal jostles the door or the antennae coming off the door, it slips past the threshold, the pressure outside forces the water in, zips in and stage two, brings the prey in with it and then immediately snaps shut right after that. Now, as shown by this clip which shows a crustacean initially swimming behind the ladder, this all happens in a very short period of time, indeed only a few milli– (scoffs) There it was! (audience applauds) In just a few milliseconds. Almost looks like a quantum effect, one time it’s inside, one time it’s outside, and it’s a very rapid movement, much faster than the Venus flytrap, and it required the invention of high-speed cameras to study. Digestions begins soon after the bladders snap shut. Now Darwin’s closest friend and colleague, Joseph Hooker, he’s the guy on the right. He was the director of Kew Gardens, first described carnivory in pitcher plants in the genus Nepenthes in an address published to support Darwin’s ideas.
Nepenthes has about 150 species in Old World tropical forests, and its pitcher-like leaves serve as pitfall traps in which insects are attracted to nectaries around the pitcher’s rim or what’s called peristome. We’ll be revisiting this a number of times later tonight. They slip on that rim or a waxy zone just below it, they drown, and then are digested in the pitcher fluid. Darwin only briefly mentioned this plant in his book, as well as New World pitcher plants, Sarracenia and Darlingtonia, that had been studied in very brief form by the American botanist Mark Canby. These 10 genera, Drosera and Dionaea, Aldrovanda, Pinguicula, Droso-phyllum, Genlisea, Utricularia, Nepenthes, Sarracenia and Darlingtonia, form the carnivorous plant canon, and for many decades, these were the only plants considered to be carnivorous. Remarkably, Darwin, and his successors, never offered a formal definition of such plants, and that impeded the discovery and study of new carnivores. In 1984, I proposed a definition based on two criteria. First, carnivorous plants must be able to absorb nutrients from dead animals, and thereby benefit in growth, survival, or reproduction. Second, they must have one or more unequivocal adaptations for prey capture, attraction, or digestion. Adaptations for all three of these processes are not required, as evidenced by pitcher plants that lack digestive glands, and bladderworts that lack attractants.
The requirement for adaptations for prey attraction, capture, or digestion, is needed to separate carnivores from just every other every-day plant, given that earthworms and nematodes, and all manner of microbes are constantly dying in the soil, and their nutrients absorbed by the roots of plants. It turns out my definition has been widely adopted, and, based on it, we now recognize over 800 species of carnivorous plants in five orders, 19 genera and 12 families. Now carnivorous plants may seem to be oddities, but they raise many fundamental questions. How frequently have they evolved independently, when and under what circumstances? What are the physiological and ecological tradeoffs that restrict such plants to some nutrient-poor environments but not others, like epiphytic perches. And how do the advantages of carnivory differ from those of other unusual mechanisms of capturing nutrients? What fraction of their nutrient budget do carnivorous plants obtain from prey, and how does that affect their ability to photosynthesize? How do traps develop? What shifts in trap structure have evolved within lineages, and how are they related to species ecology? What special kinds of adaptations do plants have to capture, and benefit from animal prey? And what kinds of genomic changes have become associated with the– are associated with the evolution of botanical carnivory? I going to address many of these questions today, giving the greatest attention to the costs and benefits of the carnivorous habit and what that implies about where carnivorous plants should be dominant, and some of the really remarkable strategies that carnivorous plants have recently been shown to pursue.
Now, over the last several years, several new groups of carnivorous plants have been documented by myself, and several other scientists, including two, species of two bromeliad genera, and one pipewort, each with pitfall traps; Roridula, from south Africa, which depends on pamerid bugs to eat and digest insects that have been trapped by the plants, and then excrete nutrient-rich wastes onto the plant. And the remarkable Philcoxia from Brazil, with tiny leaves just below white sand that trap nematodes. We’ve also learned that several carnivorous plants have evolved further to capture nutrients by acting as bivouacs or latrines for non-prey animals, or to recruit insects, to act as butlers to maintain the traps and keep them functioning. In some cases, we know now that carnivorous plants have co-opted genes that are normally used to defend against herbivores, like jasmonates, or to absorb of nutrients in the roots and these genes are instead expressed in the trap leaves. They are used to transduce signals to move trap components and secrete enzymes, and then resorb digests. So thanks to modern molecular phylogenetics, by which we can deduce evolutionary relationships among species rigorously from divergences in their DNA sequences, we now know that carnivorous plants have evolved at least 10 times, independently, over the past two to 85 million years.
Just a few months ago, my colleagues, and I used sequence data across all orders of flowering plants to place all the lineages currently know of carnivorous plants in the angiosperm family tree, and we calibrated that tree against time using the ages of two dozen fossils. The three most recent origins of carnivory, over the past 1.9 to 2.7 million years, occurred in the monocot order Poales, and involve a few species in otherwise non-carnivorous genera of bromeliads and pipeworts; all of them involve pitfall traps formed by multiple leaves, rather than the highly-specialized pitfall traps formed by individual leaves in the more ancient lineages of pitcher plants among the rest of the flowering plants. I discovered one of these new groups of carnivorous plants many years ago, in the bromeliad genus Brocchinia. Two species… they’re on the right of course, have cylindrical rosettes of bright yellow leaves that impound rainwater. Their inner surfaces are coated with a fine waxy dust that readily exfoliates so that insects lose their footing, and fall into the tank. The tank fluid is highly acidic, has a nectar-like scent, and contains, as you can see, numerous bodies of dead ants or bees. The leaf bases are studded with umbrella-like, absorptive hairs or trichomes, that absorb amino acids and mineral nutrients at high rates.
The two carnivorous species of Brocchinia dominate several ecosystems atop the rainy and extremely infertile tepuis, or sandstone table mountains of southern Venezuela. These are The Lost Worlds of Conan Doyle, which I, and my colleagues visited on foot, and by helicopter. We showed that Brocchinia has a greater diversity of specialized mechanisms of nutrient capture than any other genus of flowering plants, including carnivory, nitrogen fixation, the ant-fed habit, potentially a frog-fed tree and tank epiphytes that capture fallen plant material. We used DNA variation to infer the phylogeny or family tree of Brocchinia and trace its adaptive radiation in nutrient-capture strategies. The evolution of tightly overlapping bases that impound rainwater in tanks, and of absorptive leaf trichomes, were the key innovations that led to the evolution of carnivory, shown here in red, the ant-fed habit shown in blue, the frog-fed habit shown in green, and epiphytism shown as you can see in blue. Now six of the remaining seven origins of carnivorous plants occurred in, what we call, the asterids and caryophyllids among the dicots or if you know recent developments in plant systematic, the eudicots. And many of these asterids and caryophyllids bear glandular hairs, apparently defensive in nature. Darwin argued that such hairs were the first step toward the evolution of carnivory.
The largest and oldest of these groups, within the order Nepenthales, includes about 400 carnivorous species, about 1/2 the total, arose 86 million years ago, including plants with a remarkable range of trap mechanisms, including active sticky traps in Drosera, passive sticky traps in Drosophyllum and Triphyophyllum, snap traps in Dionaea and Aldrovanda, and pitfall traps in Nepenthes. All of them in one order. Of course they had 86 million years to do this. This phylogeny suggests that carnivory in the lineage arose associated with passive sticky traps akin to the defensive hairs seen in the non-carnivorous Nepenthales, that are sister to the carnivores, and that led to the rise ultimately of active sticky traps in the sundews, snap traps, the Venus flytrap, and the waterwheel plant and pitfall traps in Nepenthes, loss of carnivory in the adult stage of Triphyophyllum in tropical rainforests and then complete loss of carnivory in several related vines in tropical rainforests. Many species of Nepenthes, and tropical vines occur in rainy climates that would rinse away secretions from glandular hairs, rendering sticky traps inviable, and it was also, by the way, provide abundant fluid which to fill the pitchers of Nepenthes. The sticky traps of Drosera and Drosophyllum generally are found in climates that are substantially drier and hence less likely to wash the secretions away. The second largest lineage of carnivorous plants, including over 300 species in family Lentibulariaceae the butterwort family, in the order Lamiales, the mints and their relatives, evolved 43 million years ago, and includes highly divergent mechanisms for prey capture.
These include active sticky traps in the butterworts at the top, the eel traps in corkscrew plants in the middle, and then the suction traps in the bladderworts. Homology, let’s say the evolutionary similarities among these trap types remain elusive. The hope is that by identifying the genes expressed in developing operational trap leaves, we will resolve this long-standing puzzle in the near future. Pitcher plants evolved in three separate lineages: Nepenthes in the order Nepenthales in the Asian, Madagascar and Pacific tropics; Sarracenia, Darlingtonia and Heliamphora in the Americas; and Cephalotus in Southwestern Australia. Their pitchers are remarkably convergent in several features, especially in the prominently grooved lip, or peristome, that arose independently in Cephalotus and Nepenthes. This convergence has occurred despite different parts of the pitchers having been derived, being homologous, in other words, different organs in different lineages. In Nepenthes and Sarraceniaceae, the pitcher lid appears to be derived from a leaf apex, a leaf tip, while in Cephalotus, the pitcher body, is derived from the leaf tip, while the lid is derived from the leaf body, so the Cephalotus pitcher is upside-down in terms of its homology relative to the other two families. Let’s turn next to the ecology of carnivorous plants, where some of the most remarkable findings in the past several years have occurred. In his pioneering book on insectivorous plants, Darwin devoted only a few lines to their potential ecological advantage, stating that the absorption of animal matter from captured insects explains how Drosera can flourish in extremely poor peaty soils, considering the nature of the soil where it grows, the supply of nitrogen would be extremely limited, or quite deficient, unless the plant had the power of obtaining this important element from captured insects.
Darwin thus explained why carnivorous plants occur on nutrient-poor sites but otherwise said very little about the functional or ecological significance of carnivory. It was not until 1984 that my colleagues and I outlined the first cost/benefit model that addressed the context-specific value of carnivory in detail. Over the intervening years, this model has provided a conceptual framework for dozens of studies on the ecophysiology of carnivorous plants, of their ecological distribution, and of the pathways to their evolution. Today, I will briefly review, and re-assess this model, discuss how it can be extended, as we have done just in the last few months, and evaluate the degree to which its assumptions and predictions have been supported. The traps of carnivorous plants provide an alternative source of nutrients, but also involve the costs, the energetic cost of lures and digestive enzymes, of nutrient resorption, of tract activation and resetting, and of photosynthetically inefficient leaves. To determine when carnivorous plants should evolve or be favored competitively, we must compare these costs with the energetic benefits of carnivory. Carnivory should be favored whenever the energetic benefits of a small investment in carnivory exceed the costs. Our 1984 model assumed that advantages in energy capture translate into advantages in competition, and thus, to advantages in fitness, the rate of production of viable offspring. We also assumed that the initial trap structure was a simple phenotypic step from ancestral forms. No matter what the energetic or fitness advantage of carnivory, it’s very unlikely that mutations encoding a complex trap could arise all at once.
Given these assumptions, selection within species or competition among species should favor carnivory whenever the initial marginal benefit obtained via carnivory measured as increased rates of photosynthesis per unit leaf mass, exceed its marginal cost of constructing traps, measuring the same units of carbon. Carnivory might provide, as you can see on this table, four kinds of energetic benefits, involving, first, an increased rate of photosynthesis per unit leaf mass; second, an increased rate of conversion of photosynthate to new leaf tissue; third, reduction of allocation to unproductive roots; and fourth, partial replacement of autotrophy and photosynthesis with heterotrophy; that is say, energy capture by eating other organisms. Now uptake of nitrogen, phosphorus, or other nutrients via carnivory could result in benefits one, two, or three, leading to increased concentrations of RuBisCO or other photosynthetic enzymes in new leaf tissue, increased stores of nutrients to convert stocks of carbohydrates into new leaves, or decreased demand for nutrients provided by the roots. Many studies show that feeding does enhance the growth of carnivorous plants, especially under nutrient-poor conditions, but few have tested the proposed elevation of photosynthesis by feeding.
The strongest support for feeding leading to higher levels of foliar nitrogen and phosphorus, and therefore higher photosynthetic rates in new leaves, supporting hypothesis one, was provided by Farnsworth and Ellison for 10 Sarracenia species, and by Pavlovic et al. for Drosera capensis. And you can see in both cases that feeding benefits growth and also in this case, you can see that the benefit is greater in sunnier environments as you’d expect given that RuBisCO is more limiting to growth and to photosynthesis in sun than in shade. Now, we know now based on a variety of studies that Carnivorous plants get 6% to 100% of their leaf nitrogen, and phosphorus supplies, from prey. Experimental tests of hypothesis two have not yet been conducted. Some support for hypothesis three comes from comparative data indicating that carnivores often have smaller roots than non-carnivores. And finally, most of the evidence argues against hypothesis four: terrestrial plants mostly obtain nutrients and not carbon from carnivory, although some carbon uptake occurs in a few cases, probably because of the absorption of amino acids. The situation might be different though for aquatic bladderworts, which often allocate very little biomass to leaves, and can grow in complete darkness if they’re supplied with a carbohydrate-rich medium. Let’s consider plants of a given size, and ask whether a small allocation to carnivory would increase their net growth, and, thus, competitive ability.
As the amount of energy invested in carnivory rises, to the right, costs should rise proportionally in its C-curve. In addition, prey capture, nutrient absorption, and thus the rate of photosynthesis, or conversion of photosynthate to new leaves, should increase, as shown by that top curve, B, rising, rapidly at first, but then plateauing, with increasing investments in carnivory as the efficiency of prey capture or the conversion of nutrients to enhance photosynthesis or more leaf mass declines. For the moment, we’re going to assume that root allocation doesn’t vary with allocation to carnivory. And under those assumptions, carnivory should be favored whenever the initial slope of the benefits curve exceeds that of the cost curve; that is, when the benefits of small initial investment in carnivory exceed the costs. The dash lines indicate the difference between the cost and the benefits and the optimal investment in carnivory should occur right at the point that maximizes the difference between the benefit and cost curves, where their slopes, in other words, are equal. Photosynthesis is unlikely to rise with allocation to carnivory unless nutrients are in short supply, so the greatest net benefit of carnivory should occur on nutrient-poor sites.
Indeed, the usual increase in the growth of carnivorous plants when supplied with prey, seen in 17 of 20 studies reviewed recently, disappeared in almost all cases when the substrate was fertilized. If light or moisture are in limited supply, then they should also reduce the extent by which nutrient capture via carnivory can elevate carbon gain. Let me just go back here. So that’s the lower curve in shady or dry circumstances, less of a benefit. In addition, we’d expect that rich soils should elevate photosynthesis but eliminate almost all further gains that could be due to carnivory. Consequently, we reached the conclusion that carnivory is most likely to be favored on sites that are sunny and moist as well as nutrient-poor, in agreement with the habitats actually occupied by almost all carnivorous plants. And this goes beyond what Darwin had explained in the past. What kinds of habitats are nutrient-poor? Wet soils are mostly deficient in available nitrogen, either nitrate or ammonium, due to anoxia reducing nitrogen fixation, root function and decomposition. Bogs are precipitation-fed and wet, and thus deficient in both phosphorus, it usually comes out of the weathering of a rock, as well as nitrogen. Our Wisconsin bogs harbor one species of pitcher plant, several species each of the sundews and of the bladderworts.
Ancient, highly leached sands are also deficient in phosphorus and nitrogen, accounting for highly infertile soils in boreal and maritime areas that have high rainfall relative to evaporation, supporting a lot of carnivorous plants. As well as, lots of carnivorous plants in the Atlantic and Gulf Coastal Plains of the US, on very wet sandy soils, the tepuis of the Guayana Shield that I mentioned earlier, and ancient sandstone landscapes of South Africa shown here, Southwestern Australia, where my students and I were just a couple weeks ago, and in Borneo, all of them are hot spots for carnivorous plant diversity. Paradoxically, highly calcareous soils… Oh, let’s just see it, have I? I guess I’ve skipped over something here. There we go. Highly calcareous soils over limestone or dolomite are cation-rich, but often support a lot of butterworts, and we think this may be because they’re actually phosphorus deficient, the calcium carbonate complexes with phosphorus, and that may account for the abundance of butterworts on limestone. Based on tissue stoichiometry, the ratios of N, P, and K, most terrestrial carnivorous plants appear to be limited by N or P or N and P. By contrast, Aldrovanda and some bladderworts appear to be limited by potassium, by K, which may reflect, the large potassium requirements for constructing and pumping water from their aquatic traps. A second prediction of our economic model is that fire over wet, infertile substrates should favor carnivory. Frequent fires can further impoverish soil fertility by volatilizing the leaf nitrogen in the tissue burned, and they increase light levels and soil moisture by removing competing foliage, doing all that, they favor carnivory.
Many carnivorous plants are indeed associated strongly with nutrient-poor, moist habitats that burn frequently. Examples include, as shown here, Drosera, Sarracenia, Pinguicula, and Dionaea on fire-swept pocosins, and low-lying pine savannas of the Southern Atlantic Coastal Plain; Darlingtonia in serpentine fens kept open by fire in the Siskiyou Mountains in Oregon and California; Brocchinia, Heliamphora and Drosera on fire-swept, wet sandy savannas on tepuis in Southern Venezuela; Roridula, and Drosera on fire-swept damp fynbos in South Africa; and Byblis, Drosera, and Cephalotus in fire-swept kwongan, and reed-swamps in Southwestern Australia. Seed germination in Byblis, the plant at the bottom, is even driven by smoke! Germination is enhanced by chemicals in smoke. Other forms of disturbance over sterile substrates, including logging, lake drainage, or fractures or landslides that expose bare rock, can also favor carnivores without, of course, volatilizing N. A third prediction of our economic model is that Epiphytism works against carnivory and favors myrmecotrophy. That’s being fed by ants. Perches on tree boles and branches are nutrient-poor and often sunny, but are also episodically dry, and thus unlikely to support carnivorous plants. Only 17 epiphytic species are carnivores, far fewer than that expected given the number of carnivorous species and epiphytic species overall. Almost all of these epiphytic carnivores are Utricularia growing in cloud forests with traps buried below damp moss. Sunny epiphytic perches are more likely to support ant-fed plants than carnivores, due to reduced rates of evaporation in the swollen internal chambers of theses ant-fed plants via the, versus, pardon me, the active secretion of nectar and digestive fluids in most carnivores.
Indeed, almost all 300 species of ant-fed plants are epiphytes. Next, optimal investment in carnivory, at least in terrestrials, should increase toward the sunniest, moistest, and most nutrient-poor sites. Perhaps the most striking confirmation of this prediction is the cline in the relative size of the photosynthetic keel versus the carnivorous tube of Sarracenia purpurea, the common pitcher plant in New England. As shown here across 26 bogs, keel size increased with the concentration of ammonium in soil water in these natural bogs, excluding two other sites where nitrogen was not a limiting nutrient. In a bog remote from industrial areas, plants sprayed with different amounts of ammonium nitrate showed a similar pattern. As you can see on the bottom. Pitchers in another bog that received direct doses of both N and P also showed a shift to larger keels with increasing nitrogen. As expected, more keel-rich pitchers had higher photosynthetic rates. Patterns of seasonal heterophylly, producing different kinds of leaves, also support our predictions. Most carnivores do not produce trap, or produce leaves with a low allocation to carnivory, during unfavorable seasons when moisture, or temperature, or light might limit growth, as seen in the pitcher plants, Cephalotus, the Venus flytrap, and a number of other groups. Optimal trap mechanism and form should depend on tradeoffs associated with environmental conditions, with prey type, and trap type. That makes, I think, qualitative sense.
Studies on Nepenthes over the past decade provide compelling illustrations of this idea. Most Nepenthes grow in forest openings over highly infertile soils and they prey on insects. Both climate and prey type appear to shape the pitchers of these species, and it turns out that waxy traps with narrow cylindrical pitchers, narrow apertures, mostly grow in the seasonally dry lowlands, and they capture creeping insects, while traps with wide apertures, and large peristomes that are slippery when wet and have a sticky or viscous trap fluids. The trap fluid itself is a bit of a trap. Dominate in constantly wet forests, usually at higher elevations, and mainly capture ants or flying prey. Nepenthes is one of five genera of pitcher plants that feed to a very large degree only on ants. This has always struck as odd. Ants are famously successful in localizing enemies, and food sources via scouts, who then return to the nest, and recruit workers and bring the whole ant nation down to deal with either. Yet ants, I know from watching all these plants, avidly visit each of these green anteaters. So, what gives? One possibility, according to Ulrike Bauer, plant biomechanics expert extraordinaire, is that many species of Nepenthes have outwitted those smarty ants by having peristomes, or pitcher rims, that are slippery only when they are wet. Most pitchers, including Nepenthes, have a zone of waxy crystals below the pitcher opening and nectaries that attract prey; and these crystals readily detach, causing insects to lose their footing, and it turns out, the broken off scales contaminate the adhesive structure on the feet of the insects; again, causing them to lose their grip and to slide on other surfaces.
As a result, on these plants, the insects slip and fall into the abyss, under all circumstances. But Bauer has shown that several Nepenthes have highly specialized peristomes that give good traction when wet, but become incredibly slippery to insects when, pardon me. Good traction when dry, they become incredibly slippery when wet. And then it turns out that the ridges and grooves, you can see at different scales here, keep water on the peristome surface via surface tension, and they prevent the tarsi of the insects from contacting the plant surface, and so the prey just aquaplane into the drink. (audience laughing) During dry weather, the peristomes allow scouts to locate pitchers, and their nectaries and survive, return to the nest, and recruit large numbers of workers to the food source. If conditions remain dry, the ants make hay. But as you can see if things go misty, oopsy-daisy, columns of workers slide into the tank. So these traps, active only part time, which at first seems inefficient and unlikely to be favored by natural selection, might actually provide plants with bonanzas based on a crucial weakness in the social behavior of their prey. Truly a bait and switch worthy of unsavory politicians (audience laughing) by these green Machiavellis. It turns out, by the way, by hooking up electrodes to these plants, Bauer was able to show that as the film of water on the peristome increases in thickness, the prey capture rate goes up, so it’s high in the morning, in the afternoon, when the humidity is high or after rain storms.
She has also found a remarkable strategy, another way of preying in the rain in Nepenthes rafflesiana, shown here. Its pitchers have lids with waxy crystals on their undersides that reduce traction that allow insects to crawl safely under most conditions. But when it begins to rain, some of the ants and flies will seek shelter under the lid, under that little umbrella, and when the raindrops strike the lid, it bends down and then recoils, (audience laughing) and the insect is precipitated into the abyss. These movements are much faster than the Venus flytrap, require no energetic input from the plant, and essentially use rain and the prey’s own inertia against it. Basically, these are crafty jiu-jitsu warriors. (audience laughing) Now three Nepenthes species with giant pitchers at high elevations have evolved into latrines, and depend on the feces of tree shrews for nitrogen. Apparently these tree shrews are all males, because they always leave the lids up! (audience laughing) These three species at low elevation, tolerate substantial shade, apparently because they have greatly reduced secretions of nectar and tank fluid, and capture nitrogen either by acting as bat roosts, in this Nepenthes hemsleyana, or capturing leaf litter, in Nepenthes ampullaria.
Nepenthes bicalcarata though beats all. It tolerates shade by having very long-lived pitchers. These are kept functional by ants that they house in little swellings on their tendrils. You see that little twist next to the tank, the pitcher of the plant. And these butler ants, basically keep the peristome clean of fungi and algae, and, we know from experiments that have been conducted by Clark and others that the presence of the ants triples the amount of nitrogen input to the leaves as a consequence, and it’s particular effective on older leaves. Uniquely, these commensal ants can swim in the pitcher fluid, it sort of makes sense. They’re constantly cruising the peristome which gets slippery when wet so they’re going to slide in, but they evolved the ability to swim and then get back, pull themselves out of the pool. And often they will eat mosquito larvae living inside the pitcher, and in so doing, they prevent some of the food, taking up by the plant from leaving the plant and flying off with the adult mosquitoes. These ants of course will defecate into the tank fluid. Now Darwin suggested that snap traps in Dionaea, the Venus flytrap, with rapid closure, escape of small prey via spacing of marginal teeth and digestion in an enclosed chamber, may have arisen to capture larger, and more rewarding prey that could be immobilized in the sticky traps ancestral to sundew family.
Here at Maddison, Tom Gibson and Don Waller, have developed a model supporting several aspects Darwin’s ideas, and highlighting how important for the growth of Venus flytrap prey capture early in its lifespan is. Mutualistic interactions between ant prey, and pitcher plants may further affect and favor the carnivorous habit. Daniel, Joel and I, a number of years ago, independently proposed that pitcher plants in Cephalotus, Nepenthes, and Sarracenia, all of which prey primarily on ants, may actually be mutualists with their prey. Well, that’s easy to say if you’re the plant. What’s the advantage to the animal? Well, look, plants in nutrient-poor habitats are long on carbohydrates. They’re photosynthetic for God’s sake, but short on N and P, and other mineral nutrients, whereas ants in the same environment may be relatively long on nutrients, because of their predation on insects, but short on energy, on carbon. Given the large amount of nectar provided by these pitcher plants that are specialized by ants, and a few individuals from colonies we observe actually being lost to pitchers, it might pay ant colonies to sacrifice the occasional sister into the well for all the sugar gained, just as it might pay the plants to provide ants with large amounts of sugar, if they gain enough nutrients, thereby, to elevate photosynthesis by a greater amount. Now Moon et al. have recently demonstrated that… one… North American pitcher plant, Sarracenia minor, earns a net benefit from its interactions with ants.
The question remains whether a net benefit also accrues to the ants. If ant specialists like Sarracenia minor shown here are mutualists with their prey, it could generate a positive feedback between the potential benefits of carnivory and increasing prey density, with the abundance of each growing larger and larger until they eat Sheboygan. (audience laughing) Carnivorous plants should have lower photosynthetic rates, and relative growth rates. The advantage of carnivory does not lie in absolutely high photosynthetic rates, but simply in increasing those rates minus the costs. A meta-analysis by Ellison and Adamec appear to confirm these predictions for terrestrial carnivores, but there’s a deep problem with these data: The non-carnivores analyzed often grew on rich soils while the carnivores grew on poor soils. And so this study really should be redone, if you’re interested, call me. (audience laughing) Astoundingly, no experiments have yet compared growth and carbon uptake by non-carnivores, and fed versus unfed carnivores under field conditions or competitive interactions among the plants. I hope to pursue such an experiment in the near future, and if you’re interested in helping conduct that study, again please see me after the talk! Just a few last points here: Possession of defensive glandular hairs should facilitate the evolution of carnivory. The pre-existence and the co-use of such hairs would reduce the cost curve and, consequently, reduce the energetic threshold for carnivory. In accord with one of Darwin’s proposed paths to carnivory, five of the 10 carnivorous clades appear derived from ancestors with glandular leaves, as exemplified here by the non-carnivores to the left of the red line, Plumbago, Frankenia, and Tamarix, their sister to the carnivorous clade of Nepenthales. Selection for carnivory should increase if sticky traps also continue to protect carnivores from herbivores.
Field removal of secretory glands from this Mexican butterwort, show that glandless plants sustain 18 times more damage due to insects than the controls. “Vengeance shall be mine!” sayeth the insects. (audience laughing) Increased ant visitation to Sarracenia minor reduces herbivory and pitcher mortality independent of ant capture. So, remnants of defenses against insect herbivory, from which carnivory is thought often to have evolved, may play an important role in maintaining selection for the carnivorous habit. Now anoxic or toxic soils should favor carnivory, and replacement of roots by trap leaves on open moist sites. Such conditions render root function costly, and favor shifting part or all nutrient uptake to trap leaves aboveground. The widespread occurrence of carnivores on sodden soils, and their small, shallow root systems, support this hypothesis, as does the restriction to heavy metal-rich, serpentine soils of Darlingtonia, several species of Nepenthes, and few species of Pinguicula and Drosera. But, assessing the significance of shifts from root to trap allocation using our original model would ignore the complex interplay of allocation to the leaves, roots, and traps and their impacts on whole-plant growth. And so my colleagues and I have recently developed a new, two-dimensional model to explore these trade-offs. It predicts increased allocation to traps versus roots on sites.
The more inefficient roots are for nutrient capture and the greater the response of photosynthesis to nutrient supply. The key role, however, of roots in absorbing water favors a mixed trap-and-root strategy, even when carnivory is favored. Next, growth co-limitation by multiple nutrients may favor increased root allocation after prey capture. This hypothesis would seem to provide the only plausible explanation for this repeatedly observed paradoxical behavior that as some carnivorous plants catch more prey, they allocate more roots. If two or more nutrients co-limit plant growth, then prey capture elevates the level of one nutrient, increased root growth should be favored as a means of getting the other nutrient below ground. Adamec has argued that prey are such rich sources of nitrogen and phosphorus, and such poor sources of potassium and magnesium, the prey capture might favor increased root growth to retrieve potassium and magnesium. Last couple of points. Paradoxically, bladderworts in harder, more fertile waters should invest more in carnivory. Well, how can that be? Well, it turns out that harder waters contain more cations, and often more phosphorus and nitrogen, but they also contain more CO2 and more carbonate ions from which additional CO2 can emerge as photosynthesis proceeds. The greater the concentration of CO2 and bicarbonate, the more photosynthesis should be enhanced by a given increment of prey capture, favoring greater trap allocation in those harder but more fertile waters.
Indeed, Susan Knight, working here at the University of Wisconsin long ago, found that trap number in bladderworts in Northern Wisconsin lakes increases with water hardness and the fraction of biomass allocated to traps in Utricularia in Czech lakes increases with the amount of dissolved CO2. Adamec has shown that, in laboratory conditions, addition of CO2 greatly increases allocation to traps, while addition of prey only slightly increases trap allocation, and both of these are consistent with the predictions of our model. Finally, anoxic or infertile soils select against the evolution of tall woody carnivores. The almost complete restriction of carnivory to relatively short herbs is remarkable. And again Darwin did not explain that. Wet, infertile soils are favorable for carnivory but work against tall, woody plants of any kind because of their lack of, or channels in the roots and the ease of toppling such trees in wet soils. So I suspect that no matter how widely we explore the Earth for new carnivorous plants, (audience laughing) we need never fear an Audrey II scenario! (audience laughing) To conclude, many of the assumptions and predictions of the cost/benefit model for the ecology and evolution of carnivorous plants, in its original and new forms, appear to be supported. The most powerful tests of our models in the future would entail making them fully quantitative and then comparing quantitative predictions of optimal plant form and physiology with reality. In addition, field experiments on the growth and competitive ability of carnivorous plants versus non-carnivores in different contexts would be extremely helpful.
The revolution in our understanding of carnivorous plants is likely to continue as new genomic data allow us to fully understand the development and operation of the traps, assess selection on them in different environments, and quantify the impact that carnivory has on nutrient acquisition, photosynthesis, whole-plant growth, and competitive ability. Only then will we fully understand these most wonderful plants, which have ensnared our attention ever since Darwin. Thank you very much. (audience applauding)
Search University Place Episodes
Related Stories from PBS Wisconsin's Blog

Donate to sign up. Activate and sign in to Passport. It's that easy to help PBS Wisconsin serve your community through media that educates, inspires, and entertains.
Make your membership gift today
Only for new users: Activate Passport using your code or email address
Already a member?
Look up my account
Need some help? Go to FAQ or visit PBS Passport Help
Need help accessing PBS Wisconsin anywhere?
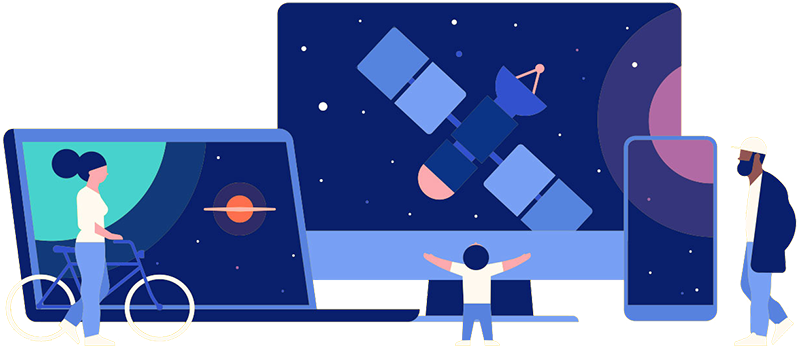
Online Access | Platform & Device Access | Cable or Satellite Access | Over-The-Air Access
Visit Access Guide
Need help accessing PBS Wisconsin anywhere?
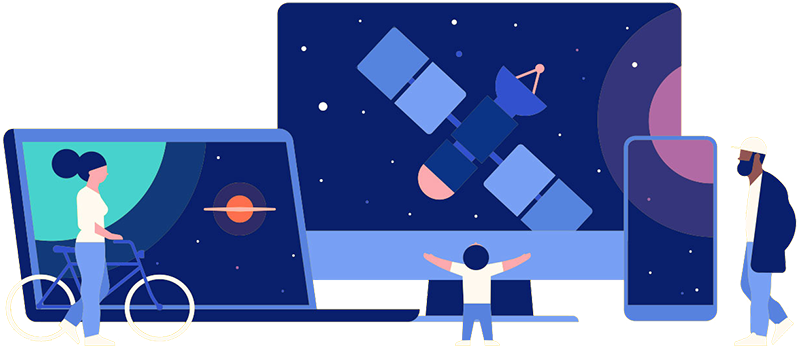
Visit Our
Live TV Access Guide
Online AccessPlatform & Device Access
Cable or Satellite Access
Over-The-Air Access
Visit Access Guide
Follow Us