– Welcome everyone to Wednesday Nite @ the Lab. I’m Tom Zinnen. I work here at the UW-Madison Biotechnology Center, I also work for the Division of Extension and the Wisconsin 4-H program that’s part of the Division of Extension. And on behalf of those folks and our other co-organizers, Wisconsin Public Television, Wisconsin Alumni Association, and the UW-Madison Science Alliance, thanks again for coming to Wednesday Nite @ the Lab. We do this every Wednesday night, 50 times a year. Tonight’s a pretty interesting thing. Yesterday was also an interesting day because it was the 125th anniversary of the publication of the Regents Report. That it ended up having that famous line on the plaque of “Continual and fearless sifting and winnowing.” I wanted to point that out, ’cause it’s one of the great things we get to do here. And since tonight is also about kids, it’s particularly appropriate ’cause I want to continue to invite Wisconsin 4-H youth and adult folks to think about coming to their campus and connecting to the people, places, and programs in science and throughout the campus.
And tonight’s a good example of the kinds of researchers that are doing things that help families and kids, and to be able advance their own science savvy. It’s my pleasure to welcome back to Wednesday Nite @ the Lab, Stephanie Jones. She’s gonna talk with us about sleep in child development. The A to Zs of building a healthy brain. Stephanie was born in Newburyport, Massachusetts. And she went to high school in Newburyport, Massachusetts. Then she went to the University of Massachusetts in Amherst, as did my mother-in-law, who may be watching. And there, she studied psychology. Then she came here UW-Madison to get her master’s degree in clinical psychology. And stayed to get her PhD in neuroscience. She’s with the Wisconsin Institute for Sleep and Consciousness. Which I think both things are a really good thing to have. [audience chuckling] So please join me in welcoming Stephanie Jones back to Wednesday Nite @ the Lab. [audience applauding]
– I am indeed here to talk about sleep and child development. I’ll first talk a lot about sleep and its function though, before I get to the child development part. Here’s a general overview of what I’ll talk about, and if I talk too fast, you can ask me to slow down. I do tend to talk a mile a minute. And if you have any questions while we go along, if something’s not clear, please let me know. I was gonna try to make this the most exciting talk ever, and then I got busy, so I wore this pink blazer instead. [audience chuckling] I figured it’ll kinda balance it out.
Hopefully it won’t be dull though. So, I’m gonna give you a definition of sleep and tell you how we measure it in the lab. I’m gonna give you evidence for why we know sleep serves an absolutely essential function. I’m gonna tell you what sleep does for the brain, what that function is. Then I’m gonna describe to you, given what sleep’s function is, why it’s particularly important during child development. And then, I’ll talk a little bit about how we can improve sleep during development. Development meaning, childhood and old age. So, what is sleep? It’s a state of reduced responsiveness to the environment that’s readily reversible. That means, readily reversible means, we can distinguish it from coma or hibernation, things like that. According to this definition, sleep appears to be an universal phenomenon.
Every animal in which it’s been studied, it seems to be present. Notice that I didn’t say anything about immobility or inactivity. So, in lots of animals, sleep doesn’t mean they lay still. And it’s certainly not an inactive time for the brain. On the contrary, it’s a really active, busy time. How, where’s my? Here we go. How do we measure sleep? So, just to give you a really basic outline, sleep is divided generally into non-REM and REM sleep. Non-REM sleep takes up about 70% of a night. Most of your sleep is during non-REM. REM sleep is about 25% of the time.
It changes over the course of development, and that’s important. But, so if you look up here, this is a hypnogram, this is basically what happens, just a kind of global view, when you go to sleep, you descend down into non-REM sleep stage three or four. This is what we call slow-wave sleep. And this is deep sleep. This when your brain is, it’s at its most disconnected, and when you are sleeping the most deeply. Meaning, your arousal threshold is higher. And this is the kind of sleep, this slow-wave sleep, that we associate with feeling restored in the morning. Okay, so how do we measure it? We measure it with EEG. This down here, these great big slow-waves, this is the sleep that I’m gonna be talking about most tonight. We measure it with EEG.
But here’s an important bit. In our lab, we use something called high density EEG. You can see a child wearing the net right now. It’s 256 electrodes. But, and what it does is it gives us a nice spatial view of sleep. This is important because we now know that sleep isn’t necessarily a global phenomenon, it can be a local phenomenon. So you can, parts of your brain can be asleep, or can sleep more deeply. And the converse is true. While you’re awake, you can have periods of micro-sleep, where little regions of your brain can go to sleep. So, we use high density EEG, we produce topoplots that look like this.
When you see the dark red, this means that non-REM sleep, these great big slow-waves, are most deep, there’s most power there. So, you’ll be seeing a lot of these topoplots as I go along. Basically, this is just in an adult, this is where most of the power in slow-waves happens. Okay, so how do we know sleep serves an essential function? First all, we spend a great deal of our lives sleeping. This is data from the Bureau of Labor Statistics, where I also learned that the average woman spends 17 years on a diet. [audience laughing] So, if you have been, when people tell you they’ve been married for 25 years, it’s really more like 16. Because you were unconscious for about 36% of that time. [audience laughing] So, presumably, if it weren’t important, nature would have somehow rid us of it, right. Okay, the other thing is, as I alluded to when we first started, is sleep is universal. Every organism that has been studied, down to the lowly fruit fly, engages in sleep.
And nature has devised all, or evolution has supported all kinds of adaptations for this behavior. This is a parrotfish. When he’s gonna go to sleep, he secretes a mucus bubble for himself. Dolphins and other aquatic mammals will sleep unihemispherically. This is so they, so they put one half of the brain to sleep and keep the other awake, so they can move and come to surface to breath. Birds similarly, they will engage in unihemispheric sleep. And long distance migrants will sleep in flight. So if they’re coasting over the ocean, let’s say. So it’s an universal phenomenon. This is my cat and my dog by the way.
So, this another piece of evidence to support the idea that sleep is essential, right. Evolution wouldn’t have come up with all these ways of keeping sleep if it weren’t important. Sleep is also dangerous. We don’t think about that as humans. But for other animals out in the wild, right, the risk of predation is high. For us, sleep is dangerous in the sense that it comes at opportunity costs. We can’t work, we can’t reproduce. That’s my cat again. In my family we play a game, sleeping or dead? [audience chuckling] Because this cat’s spends most of its time just like that. How else do we know it’s important? It’s developmentally regulated.
So sleep, everything about sleep changes across the lifespan. So the time you spend in sleep changes across the lifespan. These are recommended sleep durations. You can see there’s tremendous change. The composition of sleep changes across the lifespan. I’ll go back to that slow-wave sleep I’m gonna talk about. As children, so this is the first year of life, during infancy, the first year babies spend a lot of time in REM sleep. This is probably partly about developing motor systems. But, as a child ages, the percentage of time the child spends in non-REM sleep, particularly this deep sleep I’m talking about, slow-wave sleep, increases. And it does this in concert with brain development.
So this is the first piece of evidence I’m gonna give you, that maybe it’s an important part of brain development. This is an EEG. Just from the front of the brain of an 11-year-old child. This is, same location, same scale, a 20-year-old. You can see this is an incredible change, right? So, the other reason I’m arguing that we know sleep is essential is because it is developmentally regulated. So how do we know it’s essential and it has an essential function? Takes up a lot of time, every organism does it, every organism does it despite risks and costs, and it changes across development in profound ways. How else do we know sleep is essential? We know just generally that when sleep is abundant, the brain works well. We hear this all the time, learning and memory is better. This is just some data, this is 8,000 students in Norway, 16 to 19 years old. This is just showing the relationship between grade point average and hours of sleep.
So basically, as you can see, so this is fewer hours, more hours. As sleep increases, so does performance. I could’ve shown you any number of studies like this. This is just a kind of 3,000 foot view. How else do we know that sleep is important? We know that when we don’t get enough sleep, things go really wrong. We know that emotion regulation is compromised. This is certainly the case in children, but in adults too. We know that learning is compromised. I’m showing you here, this is just for me to highlight for you that we know learning is compromised. This is one study where they took participants, they deprived them of sleep, and then gave them a task.
A bunch of images, let them go off. They went to sleep, they came back the next day and were asked to look at those images along with some other new images. As you can imagine, the people who had been sleep-deprived before the task did poorly, despite the fact that they slept after the fact. In contrast, the typically sleeping controls performed the task quite well. When we don’t get enough sleep, so we’re sleepy, we’re grouchy. To put some– To make it concrete, we know that risk of driving accidents is quite high when we are sleep-deprived. Teen drivers are at the highest risk of car accidents due to falling asleep. And it accounts for about 50% of all crashes. And some major disasters, Chernobyl for example, have been credited with sleep deprivation. So, we know that when we don’t get enough sleep, things don’t go badly.
But why is it? What is it about not getting sleep that makes things go badly? So what I’m gonna tell you about tonight, what I’m gonna argue is that, sleep is the price we pay for synaptic plasticity, or learning during our day. So, what is plasticity? Plasticity is the capacity for the brain to modify its structure and its function based on experience. This is the foundation of how we learn. So, from the brain’s perspective, plasticity is learning. And what I and those of us who do research at the Wisconsin Institute for Sleep and Consciousness would argue, is that, oh no, so, back up. So, the hypothesis I’m gonna talk to you about tonight is called the synaptic homeostasis hypothesis. And the basic idea, and it was developed by two researchers here, Giulio Tononi and Chiara Cirelli, both of whom are at the Wisconsin Institute for Sleep and Consciousness. So the basic idea is this, when you’re awake and learning, and by learning I mean simply engaging with your environment. I’m gonna show you learning in a formalized way, right, in a way you study it in the lab. But everything, as we go about our day, we are learning.
That means just taking in information and processing it. So, as you go about your day and you learn, synapses get stronger. Right? And then, when you got to sleep, and as that process happens, the brain becomes more and more expensive to maintain. When you go to sleep, sleep takes down all of the noise or the synaptic weight of the day. And in that way, it enhances learning, memory, just extraction, all those things. So that’s the basic outline of the synaptic homeostasis hypothesis. But I’ll go through it in detail. So when you’re awake, you’re learning. This seems like it would be intuitive, but people do talk about learning during sleep, learning from our dreams for example. But it doesn’t make sense for an organism to learn when it’s disconnected from the environment, right? So, when you can perceive your environment and adapt to it, you’re learning.
And this happens while you’re awake. Again, I’m gonna give you formalized examples of learning in a lab. But, we’re all learning right now. From a neuron’s perspective, learning is just increasing synaptic strength. So a neuron doesn’t think. But what it does is, when things happen by more than chance, it fires. So when it fires, neurons that fire together get stronger. So synaptic strength gets stronger. This is the neural mechanism for learning and memory. But plasticity is a demanding thing.
So the brain, even at baseline, is an expensive organ. It weighs about 2% of our body mass, and it consumes about 25% of our resources in terms of glucose. So more synapses become more and more expensive in terms of energy costs. They take up a lot of space, they demand a lot of resources. So, at a certain point, the brain has no longer capacity, has no more room, think of it that way. See stuff leaking out there. That’s the basic idea. So, what does sleep do? And I’m gonna describe in more detail. When I say sleep, I am talking about slow-wave sleep. But, so what sleep does is, it is in the business of downscaling these synaptic weights.
So in this way, sleep allows for the memory consolidation. All the things that we think about happen during sleep. You wake up and you’ve slept on a problem and suddenly it seems better, just extraction, insight, integration, consolidation of things you’ve learned. So what sleep does is it basically takes down the noise of the day, and what emerges is what’s important. So, I like to think of it as a reset button. Everything good so far? I’m gonna give you another summary. So what I just told you was that sleep serves an absolutely essential function. Waking is associated with learning, which is represented in the brain by increased synaptic strength. Sleep downscales synapses that aren’t important and preserves those that are. In this way, it sort of saves, it serves as a save button and a reset button.
So, I’m gonna give you a little bit more detail about slow-waves and what they are. So going back to this image, which I’ve already shown you. So, this is deep sleep, as I’ve said. And what it is essentially this, when you go to sleep, neurons start to have periods where they are silent, off periods, when they’re not firing, and then on periods where they are. And, in the cortex, the more of these neurons synchronize to one another. So the cortex is a highly integrated space. And as the cortex goes to sleep, and more of these neurons are having periods on, off, on, off, it emerges on the EEG as these great big slow-waves here. This is a picture of just one channel. So just one channel right in the front, that’s what you’re seeing here. So, what we know about slow-wave activity, what we’ve known for a long time is this, that when we just used standard EEG, it was always understood that sleep is hemeostatically regulated.
So, the longer you stay awake, the more you need to sleep, and the deeper your sleep is. Deeper meaning, the more slow-waves you have, or the more slow-wave power, or slow-wave activity. So, if I were to keep you up for extra long, you would sleep more deeply. So this is what slow-waves would look like in your regular night of sleep. But say we deprived you for a night, what we would see is more slow-wave power. We knew that, we’ve known this for, that sleep was hemeostatically regulated for maybe 40 years. And this is, I’m showing you this same phenomenon here. But these are those topoplots, right. So this here is slow-wave power. Redder is more.
And so, what we know from using high density EEG is that, power is always greatest in the front of the brain. So this red electrode is the frontal electrode. So power is always greater here. As you sleep, this is the first sleep cycle, second, third, power dissipates. So you stay awake, you build up sleep pressure as it were, sleep pressure, the need to sleep, is dissipated by slow-wave power. And it’s important that slow-wave power in an adult is always greatest in the front. Because what we know now is that it’s not just about sleep need, it’s about use. So the more you use a brain region, the harder, or the deeper it will sleep. So now I’m gonna tell you what, I’m gonna talk about plasticity and learning. And so remember, I’m saying that the function of sleep, the function of these slow-waves is to regulate plasticity, which is the foundation of learning.
And I will show you some things that we have done in the lab to illustrate this plasticity/learning relationship. And again, so these topoplots, when you see red, that means slow-waves are highest there. If you see blue, they’re lowest. And I want to again stress that I am, we are using a simple accessible system. But presumably, so I’m gonna show you a simple learning task. But the broader picture is learning. It’s not just about these task-based learning. I’m showing you this just to illustrate this principle of the relationship between learning plasticity and sleep. So, and this is all work that has been done here over the last many years. The first thing that I’m showing you is just a simple thing.
Establishing the relationship between plasticity and slow-waves. And again, to illustrate for you that these, that sleep can happen regionally or locally. So you can take TMS, which is just a great big electrical coil, and you can stimulate neurons so that they fire. You induce what’s called long-term potentiation. And what you see if you do this, this is my colleague Brady Riedner, if you do this, what you see is a regional increase in slow-wave activity over the motor cortex. So right there, we’re stimulating the motor cortex. So what I’m showing you here is that basically I’m demonstrating that if you just induce plasticity, you will have an increase in slow-waves in the place where plasticity has been enhanced. But what I’m arguing here is that, learning, so plasticity is the foundation for learning. So, I can also show you that if you make a person use a particular part of the brain, in this case again, it’s the motor cortex. This is an MRI image showing the region where this particular task works.
So what we did is we took people into the lab. And they’re counterbalanced. We had them do the following. So one night they come in, and they just have to rotate a mouse to a target. There’s no learning involved, it’s just a motor task. So presumably it uses the motor cortex to some extent. And we know the task, this is the motor cortex. So, the next time they come in, they actually have to do an implicit learning task. And it’s this, they have to rotate the cursor again, to a target. But the target moves.
And they can’t figure out why it’s moving, but somehow they learn to adjust to the moving target. They sort of figure it out. What we know, what we see is that when they learn that task and we put them to bed at night, lo and behold, in that region, the region of the motor cortex that they used robustly, we see an increase in slow-waves. That increase in slow-waves is correlated with how they perform the next day. So the more slow-wave activity you have, the better you’ve learned. So we know that learning induces increases in slow-wave activity, and it’s directly related to how well you perform. So the basic idea here is that you use your brain to learn something, and in order for that learning to get better, you need slow-waves. I can also tell you that it’s true in animals. So, this is a similar kind of task for a rat. He has to reach in and grab a sugar pellet.
It’s difficult to see, but this is in fact a hard thing for them to do. So, here’s the little hand reaching, reaching, reaching. You have these, you train these rats to reach for this sugar pellet, and what you see is an increase in slow-wave activity, in the contralateral motor cortex. So if you look at one side of the brain, there’s no increase in slow-wave activity. But there is in the trained cortex. And because rats only need short bouts of sleep, you see this effect in the first bout of slow-wave activity. And the percent of how well the rat did is correlated with slow-wave activity in the subsequent sleep. So if they’re really good at it, they have a lot of slow-wave activity in the trained motor cortex. Does that make sense? So basically, stronger synapses, better learning, higher slow-wave activity. But, I started out by saying that there’s a price that we pay for plasticity, right? That was the stuff leaking out slide.
So plasticity can’t go on forever, because the brain is expensive at baseline. And if you learn, and learn, and learn, and learn, at a certain point you become, the brain becomes completely saturated. And what does that look like? So what is the price of plasticity? So we know that if you keep a person up for a really long time, things go badly. But what exactly goes badly? So this is just taking a rat and sleep depriving him. And what you see is, as he’s awake, if you’re recording from his cortex, he will start to have slow-waves appearing in his brain. So, he’s wakeful, and he can even be moving and behaving. But the longer you try to keep him awake, the more difficult it becomes. And he will have intrusions of non-REM sleep during the waking state. So this is called a micro-sleep. We presume that in humans, micro-sleeps are responsible for lots of traffic accidents, for example.
And you can demonstrate a similar kind of thing in people. So again, we know that the harder you use a certain area, the greater your use, the higher your need for sleep, for these slow-waves. So this a task where we took people into the lab and had them do a task for 36 hours. They either listened to an audio book, or they did driving simulation. So this is an MRI image showing you where, so these are complex tasks, and they use lots of the brain, but I’m showing you a view of the cortex. And so, the audiobook in general, taps into this brain region, whereas the motor simulator taps into this brain region. So you keep them awake, they stay awake, and they’re doing this task for 36 hours, the driving is activating this bilateral occipital lobe area. So these tasks tap into different brain regions. And this is the important part, so we’re working this part really hard in the audiobook listeners, and this part really hard in the driving simulating task. And what happens? So even in humans, you can see that as, so what we’re doing here is we’re pushing plasticity.
We are overusing the brain. It’s extended use. And what happens is, you start to see the intrusion of slow-waves into those particular brain regions. So basically, this is the audiobook, these are slow-waves intruding here, this is where the slow-waves intrude during driving simulation. So what has happened is, we’ve pushed plasticity so far that sleep is invading wakefulness. So these people are still performing. They’re performing their task, they’re awake. But bits of the brain are basically going offline. So the parts that we pushed the hardest are going offline, they’re falling asleep in an otherwise wakeful brain. This again is that same task in the rat reaching.
So, you can also try to make rats do this task for a long time. You keep them awake, they’re motivated to do it because it’s sugar. But what you find here is the same thing. So the motor cortex that they’re using, and using, and using, gets sleepy, right? They’re still performing, they’re still engaging in this behavior, but what you see is, those slow-waves will start to intrude. And when they intrude, mistakes happen. So the rat is more likely to miss a target, miss his reach, as a slow-wave intrudes. So the idea here is that you can use the brain, but only to a certain point, right? So the brain is plastic, but it can only go so far before sleep must happen. So, what I’ve just argued is that sleep functions like, slow-waves in particular, slow-waves of non-REM sleep, function like a reset button. So, waking is associated with synaptic strengthening. And this forms the foundation of how our brain learns, how we learn.
Without sleep, the brain becomes saturated, and learning can no longer happen. Sleep downscales those synapses, leaving the important ones strong in complicated ways. And by increasing the signal-to-noise ratio in the brain, sleep improves memory and frees up space for learning the next day. So rather than, so the argument is that, rather than having it playing a really active role in say, consolidating memory, what sleep is doing is taking down all the noise and revealing, or leaving behind, takes down synaptic noise, and leaves behind the important stuff. And in that way, aids in memory consolidation. But, so the important thing is that, the important thing I’ve tried to show you is that slow-waves are necessary to both learn and to consolidate. It’s a two-way street. So, if you don’t have a good night’s sleep, and you don’t get enough, let’s say, slow-wave sleep, learning becomes a real challenge. That feeling that we have, not being able to focus, our brains are essentially saturated. So you need sleep on both sides of the coin.
You need sleep in order to take in information, and then, to consolidate what has been taken in. Why should we care about slow-wave sleep in particular, during child development? Finally, I’m getting to this part. I go back to plasticity. So, plasticity is the ability of the brain to modify its structure and function based on experience. And what do we know about children? Or early development? The brain of a child is an incredibly plastic thing. Much more so than an adult. This is a little bit depressing, I don’t have any data to support this, but the idea is that when you’re young, your brain is really plastic and open to experience. As you get older, not so much. And the effort required to learn becomes much greater. So we also know, not only is plasticity much greater during childhood, this is when most of our learning is happening.
So just for that reason, we could say sleep was important. But, in particular, the cortex, which is what I’ve been talking about all this time, we know that the cortex is undergoing protracted development across childhood. This is an MRI image, this is not our work. This is an MRI image of synaptic density across childhood. This here is the five-year-old child, this here. And we descend down to 20. When the cortex is blue, it means it’s sort of thinned out, and it’s made all of its synaptic connections. But this process takes a really long time. We know from monkeys that this is modeling, so what we’re seeing here is in fact synaptic density. So it makes sense, right? So in the back here, this is a five-year-old child, what’s happening most in this child’s life is posterior regions are actively developing.
Things like vision. As the child progresses, the frontal cortex is developing. And we think that the frontal cortex sort of fully develops, or comes online somewhere in the early 20s. So across, as a child is growing, his cortex is developing still actively. Some of that, much of that is based on experience. So the other thing we know is that slow-waves mirror this cortical maturation. So this is not something we’ve known for a long time. This data, well it’s been about 10 years now. But so slow-wave power is greatest in children in areas that are actively undergoing development. So it sort of mirrors.
This is slow-wave power in a five to eight-year-old child. This is a large sample of kids, broken down into these age ranges. So you’ll see here, at about 17 to 20 is when slow-wave power becomes kind of adult-like. So that when you go sleep, most of your power happens in the front. Because in this age, this is where most of the business of the brain is getting done, in the front. Which is true for adults, we hope. So plasticity is higher during development, and slow-waves, which we know are essential for learning and synaptic plasticity, mirror the progress of synaptic development across childhood. Now, I haven’t told you, and there’s also animal work to support this, that sleep and wake during development modulate synaptic spine turnover in a way that it doesn’t in an adult brain. So, why should we care? I have argued that slow-waves are essential for synaptic plasticity. We know kids are really plastic, it’s a critical time for brain development.
We also know, and you probably hear about this a lot, that sleep duration is decreasing remarkably across childhood. So this is data from a large study. This isn’t slow-wave activity, it’s just sleep duration. But since the 1990s, this dark line is now, this is the percent of adolescents who report sleeping more than seven hours a night. So it’s about 62%. An adolescent needs about 9 to 10 hours a night. This is the percent of adolescents who report regular adequate sleep. So not only is it declining, but since the ’90s, it’s been wildly too short. So during this particularly vulnerable period of development, sleep is sort of decreasing. This is data from the, this is from some government body or other, but I could show you the data from studies of teens in the United States and other countries.
The basic idea is that about 70% of teenagers and 60% of middle schoolers get remarkably insufficient sleep despite a high sleep need. Adolescence in particular, is a particularly risky period for brain development, because as I said, the frontal cortex is just coming online. And lots of psychiatric disorders emerge during this period of development. It is a potentially vulnerable period for sleep loss, but it seems that adolescents are increasingly sleeping less, and less, and less. And part of this is related to school demands. Part of it is social. But the other thing about adolescents is they have a biological propensity to delay sleep onset. So you probably, if any of you have had a teenager, you know that they want to go to bed later, and later, and later. This is a biological phenomenon. It’s fueled by social issues, by use of phones up against their face late at night, giving themselves light exposure at the wrong time.
But they’re still having to wake up early to go to school. So sleep is getting shortened, and shortened, and shortened. So you might have heard that there is a push to delay school start times. This is happening in middle schools in Madison a bit. There’s been some incredible data on positive outcomes for delaying school start times in adolescents. In particular, I mean, there’s academic performance data, SAT scores, but also some startling data from districts across the country about traffic accidents. So in Minnesota, this one district, a large district saw a 65% decline in teen car crashes in the morning when they delayed school start time one hour. So, they looked at a two-year period before the delay of the start time, and then, the two-year period following. And it also coincides with youth were sleeping, the percent of kids who were sleeping greater than eight hours a night in the early school start time was about 33%. That went up to 66% when they delayed the school start time.
So there are lots of ways that we can optimize sleep during development. This is just one of them. And there are, you know, but I’m gonna tell you a research way, of course, that we can optimize sleep. So one thing that we have been doing at the Wisconsin Institute for last decade or so, Giulio Tononi and Brady Riedner, and Gary Garcia Molina, have been working on a device that stimulates slow-wave sleep. So for many years we have known, 30 years we have known that if you play a tone during sleep, one of two things can happen. Either you arouse a person, or you put them more deeply to sleep. So this is a biological phenomenon. But we have all been working on optimizing this functionality of the brain. And so this is a child wearing the device. So the device has been developed in concert with Philips Respironics.
They are the partner who sort of put the thing together in this wearable platform. So for years, we would send people home with a giant laptop computer and all these electrodes. But now, the thing has become this lovely device with an amplifier. There’s an electrode under there that records EEG. There are headphones here that play the tone. So it’s a closed-loop system. It essentially, as you’re sleeping, it recognizes slow-wave sleep and it has an algorithm that says, “Okay, this person is asleep enough, I’m gonna play a tone and see what happens.” So I have been particularly interested in using this device in adolescents. We’ve also used it in older-aged people over 50, but I’ve been particularly interested in using it in adolescents because of this issue that adolescents have. Now keep in mind, there are healthier ways to overcome the sleep debt that these kids have.
But at the same time, it’s usually a phase. So during high school, early college, kids are actively restricting sleep. Usually we outgrow this. But what I’ve wanted to do is use this device to see if we can improve slow-wave sleep and cognitive function. So what I’m showing you here is, this is just a baseline, this is a hypnogram. This is, like the image I showed you at the beginning of the talk. This is a person descending into sleep. Here he is in his deepest sleep. Comes back up. This is from an in lab study.
This is data from the device at home. Just showing you that it does exactly what, it records sleep in exactly the way we would think it would do. Here is slow-waves in one child. When I am doing a stimulation, the tones are playing, versus, so this a block of on stimulation, this is no stimulation. So what I remarkably have seen is that we can increase slow-wave sleep from anywhere from 10 to 25% in a night of sleep in kids. Which is remarkable. I mean, these particular kids weren’t necessarily sleep-deprived, they’re doing their normal short sleeping. And this is data from two kids, an 18 year-old man and a 15 year-old boy, a week at home. So I had the kids wear the device at home, Sham is they’re wearing it, it’s just recording their sleep. Stimulation is I’m playing the tones while they’re asleep.
And you can see here, I can increase slow-waves by about 26% one week relative to the other. And in this child, 16%. So it’s I think, an exciting technology with a lot of potential. Particularly in this sort of population where they are actively restricting sleep. It’s not a tool that is effective in people with sleep disorders because you have to be, you have to have enough slow-wave sleep for the device to even stimulate you. But in adolescents, or in people who essentially work too hard, don’t sleep enough by choice, I think the device has a lot of potential. So in summary, what I’ve said basically is that sleep is essential, and it basically serves as both a reset button and a save button. You need sleep to learn and to consolidate. And that’s it.
[audience applauding]
Search University Place Episodes
Related Stories from PBS Wisconsin's Blog

Donate to sign up. Activate and sign in to Passport. It's that easy to help PBS Wisconsin serve your community through media that educates, inspires, and entertains.
Make your membership gift today
Only for new users: Activate Passport using your code or email address
Already a member?
Look up my account
Need some help? Go to FAQ or visit PBS Passport Help
Need help accessing PBS Wisconsin anywhere?
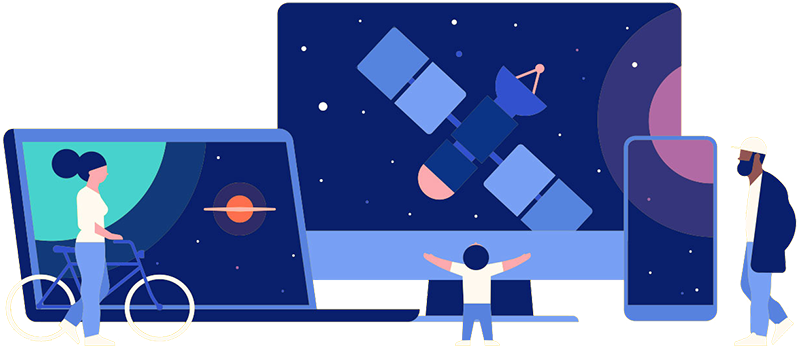
Online Access | Platform & Device Access | Cable or Satellite Access | Over-The-Air Access
Visit Access Guide
Need help accessing PBS Wisconsin anywhere?
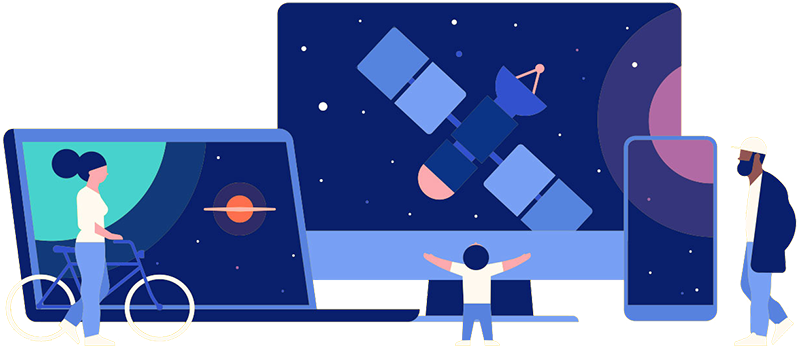
Visit Our
Live TV Access Guide
Online AccessPlatform & Device Access
Cable or Satellite Access
Over-The-Air Access
Visit Access Guide
Follow Us