– Welcome, everyone, to Wednesday Nite @ the Lab. I’m Tom Zinnen. I work at the University of Wisconsin-Madison Biotechnology Center. I also work for the Division of Extension, Wisconsin 4-H. And on behalf of those folks and our other co-organizers, PBS Wisconsin, the Wisconsin Alumni Association, and the UW-Madison Science Alliance, thanks again for coming to Wednesday Nite @ the Lab. We do this every Wednesday night, 50 times a year. Tonight, it’s my pleasure to introduce to you Hilary Dugan. She’s a professor in the Department of Integrative Biology here. She was born in London, Ontario, and then went to high school in Woodstock, Ontario. She studied both biology and geography at Queen’s College in Kingston, Ontario. Then she got her PhD at the University of Illinois in Chicago. In 2014, she came to UW-Madison, where she’s with the Center for Limnology. Tonight, she’s gonna speak with us about the effects of salt on Wisconsin’s freshwaters. Would you please join me in welcoming Hilary Dugan to Wednesday Nite @ the Lab?
– Thanks, Tom. So as a limnologist, I study lakes and water quality, and there’s no better place to do that than Wisconsin. And today, I’m gonna talk to you about salt and what salt means for Wisconsin’s freshwaters. So living in Wisconsin, you can’t open a news site in the winter without reading something about salt. Whether or not it’s talking about the horrible morning commute where it took you an hour to get to work or the fact that road salt is harming our freshwater lakes and our drinking water. And we’re all familiar with salt, especially road salt. We see plow trucks with beds full of salt driving around the streets in the winter. We see piles of salt on sidewalks and in parking lots. This is something we’ve all lived with for most of our lives.
And what I wanna try and convince you of today is that when we use salt on a large scale, the scale at which we’re currently using it, we really need to think of salt as an environmental pollutant. And it’s really no mystery why we see marine organisms in some of our freshwater rivers. This is in Toronto, Ontario, Canada, where they found crabs in a nearby river. And it’s because the river was so salty from road salt runoff that these crabs could happily live in that environment. And so today, I wanna talk about a few things. First is gonna be about some of the scientific terms we use. So, what do we mean when we say salinity? Then we’re gonna talk about how much salt we use, and then what are we seeing in freshwater lakes? And what does that mean for those ecosystems? So the word salinity is one we’ve probably all heard of, but you might not know how we actually measure it. So this is a scale of salinity from zero grams per liter to 35 grams per liter.
And the ocean is considered saline. It has 35 grams per liter of salt, whereas freshwater’s on the other end of that scale at about zero to one grams per liter of salt. And almost all of our surface water in Wisconsin falls beneath that one gram per liter line. So we have almost entirely freshwater in this state. And then everything in between 1 to 35 is considered to be subsaline or brackish water. So, how do we actually measure salinity? Well, the term salinity is defined as the total dissolved ions in water. So we actually have to go out and measure all the individual ions and add them up. So mostly, those are our major cations, so these are our positively charged ions. So that’s sodium, calcium, magnesium, potassium. And then our negatively charged ions, so sulfate, like carbonate and chloride.
And when we add those up, the total number is equal to the salinity of the water, or sometimes we use the term total dissolved solids. And what ions we find in which water bodies differs based on the water body and where you are. So the ocean is saline, 35 grams per liter of salt. Most of that salt is sodium and chloride ions, so 86% of the ocean. And that’s because these ions are not used by a lot of biological organisms, so they stay dissolved in the water. Whereas if we were to look at the salinity of lakes and rivers in, say, southern Wisconsin, what we see is very low total salinities, and most of that is made up of calcium and bicarbonate. And that calcium is coming from things like rock weathering. And to get that total salinity value, we have to measure all of those ions, which, unfortunately, is pretty time-consuming. We have to take a water sample. We have to bring it back to the lab.
We have to measure all of those individual ions on pretty sophisticated machinery to get that total salinity value. So it takes a lot of time and money to actually measure the salinity of water. So it’d be really nice if we had an easier way to measure salinity, and luckily we do. We use what we call electrical conductivity of water to tell us something about salinity. So because all of those individual ions are charged, the more ions you have in water, the better able water is to conduct an electrical flow. And so we’re actually just able to measure this using a sensor. And so we can take a sensor out to a lake, put it in the water, and measure the electrical conductivity. This is a picture of us using an electrical conductivity meter on Lake Mendota. So we can just stick that sensor right into the lake. And in this example, the lake is 506 microsiemens per centimeter, that’s the unit for conductivity, on this day.
And so if we see that number increasing, we know the lake is getting saltier. We don’t actually know which ion is causing that. It could be calcium, it could be sodium, it could be magnesium. We just know that there’s more ions in the lake. So this is nice ’cause we can go around to any body of water, use these sensors, and get a sense of what the salinity is. You’ll also see limnologists talking about chloride. So chloride is just one ion that makes up total salinity. But chloride is unique because in Wisconsin waters, the background concentration is actually very, very low, about zero to two milligrams per liter of chloride. So if we go out and we measure chloride and see it at higher concentrations, we know that that’s probably being caused by human pollution. And so instead of measuring that whole suite of ions, we go out and we measure just chloride ’cause that can tell us something about chloride contamination.
And so these terms, salinity, electrical conductivity, chloride, they’re all ways that we measure salinity and track salinity changes over time. So why is salt such a pervasive pollutant? Well, there’s three main reasons. One is it’s really effective. So salt works by depressing the freezing point of water, and it works really well between the temperature 15 degrees Fahrenheit and 30 degrees Fahrenheit. And so if we put it on our roads in, say, 25-degree Fahrenheit weather, instead of water freezing into ice, it’s gonna stay liquid. And so we use this as a way of making our roads safer. We’ve all seen it in action. Really effective. Salt is also relatively cheap. You can go to the hardware store and buy a bag of salt for $5, not breaking the bank.
The state can buy a ton of salt for about $80. There’s not very many substances you can go and buy a ton of for $80. And that price has actually gone up pretty drastically over time, so we are spending a lot more money on salt, but for the quantity we buy, it’s still relatively inexpensive. And then the third reason is salt is natural. We eat salt every day. We have sodium chloride on our dinner tables. And so how can it be bad if we are eating it? Well, just like us consuming salt, when we have too much, it causes problems. And so salt isn’t bad at low quantities; it’s when we start using a lot that it becomes an environmental pollutant. And this is kind of like coal. It’s effective.
It’s really good at heating. It’s relatively cheap, we can mine it pretty easily. And it’s natural. And coal was not a problem when we used it in little quantities. But when we started using it at an industrial scale, it’s caused some pretty catastrophic environmental damages. So, what’s the downside? Well, every ounce of road salt that we use ends up in our environments. No one’s going up and picking up the salt that we put down. So every ounce we put on the ground ends up in our soils and in our rivers and lakes. And that’s a problem because the United States puts down over 23 million tons of road salt a year. And that number is just at the state scale.
It’s not accounting for sort of other sources of salt beyond road salt. And we need freshwater. As humans, we need freshwater to drink. We also need it for industrial applications and food processing. And aquatic organisms also need freshwater to survive. Freshwater organisms have evolved to live in freshwater habitats, and as we start adding salt, some of those organisms are gonna die. And so if we keep increasing the salinity of our freshwaters, we’re gonna have some pretty catastrophic consequences to both organisms and human health. So because of that, the government has some ecotoxicity regulations on what they think are the important limits for freshwater habitats. So this is a scale of chloride, showing zero chloride all the way up to ocean concentrations. So the ocean has about 19,000 milligrams per liter of chloride.
And the EPA has set the chronic aquatic toxicity threshold at 230 milligrams per liter. So that means that if a water body is above 230, it has sort of a threatened habitat. Now, this is a feature of environmental governance where we wanna set a threshold, saying that anything below that threshold’s okay, anything above that threshold’s a problem, which is not really true in reality. There’s some organisms that are harmed at really low concentrations and there’s other organisms that are pretty fine at quite high concentrations. The problem being that the organisms that have evolved to freshwater environments in Wisconsin, so our native organisms, are the ones that are usually more susceptible to higher salt concentrations. Invasive species like zebra mussels don’t care about higher salt concentrations. And so these environmental governance thresholds are really treating water as sort of a sink for salt. How much salt can we put into the river, flush it downstream before it becomes a problem? So 230 milligrams per liter, that is a number that doesn’t really have any relevance to us, right? We don’t really think about concentrations of salt. So let’s think about what that looks like. So if we have a five-gallon bucket, and this is full of pure water, so just H2O, think about how much salt we might have to add to this bucket to produce a concentration of 230 milligrams per liter.
So think about that for a second. If you were to dump salt in here, when would it hit that EPA chronic toxicity threshold? Well, it only takes about a teaspoon of salt, shockingly. Not very much salt to produce 230 milligrams per liter. So it shows you that it’s really easy to pollute water with salt. Usually, we see those five-gallon buckets just full of road salt. And you can think about how easily it would be to pollute, say, a shallow lake or a stream. So, how much salt are we using? What are we talking about? Well, Wisconsin doesn’t have a total budget for how much salt the state uses, but someone in Minnesota did this a few years ago for the state of Minnesota. And Minnesota is pretty comparable. We have very similar populations, we have similar density of roads, lots of similar land use in terms of agriculture in the south and forest in the north. And most of the salt that’s used by the state is road salt.
So road salt is by far the biggest salt source in Wisconsin and Minnesota, but there’s other sources as well. One of that is water softeners. So when you put salts in your water softener, that effluent is sent to the sewage treatment plant or your septic, and that salt is not removed. So there’s no economically feasible way to remove salt from water. And so the wastewater treatment plant is just gonna be sending that salt downstream wherever the effluent is discharged, so probably your local river. And then we also see salt coming from agriculture. So there’s salt in livestock manure, excretion, and also some salts in fertilizer use. So potassium chloride is a popular one. And so all of these add up to be environmental pollutants. All of these salt sources are not being removed from the environment.
The only way they’re removed is sort of eventually flushing downstream. So think about Wisconsin. We have 34,000 lane miles of roads and we apply about half a million tons of road salt per year. And that differs a lot year to year. Depends on how snowy the winter is, how long the winter is. But in general, we spend about $80 million in total annual expenditures on road salt. So this is an environmental problem, but it’s also an economic problem. If we can use less salt, we also save a lot of money, and we can put that money towards things like fixing potholes. And salt is expensive because Wisconsin doesn’t have any natural salt supplies. We actually have to import all of the salt we use.
And so these are pictures from the Milwaukee Harbor last year, offloading salt into piles that will eventually be either put on trains or trucks and delivered around the state. And the result is that we see really high salt concentrations in our rivers and lakes. So this is data from Milwaukee. These are three rivers, urban rivers in Milwaukee, the Kinnickinnic, the Menomonee, and the Milwaukee River. And what we see is really large swings in chloride concentrations depending on the time of the year. And so this data shows these big annual swings where we see very high concentrations in the winter. So that makes sense, we’re applying road salt in the winter, that melts, flushes into our rivers, and then in the summer, it goes back to lower concentrations. And on this graph, you can see two dashed lines, and these are the EPA toxicity thresholds. So 230 milligrams per liter is the lower one, and that acute toxicity threshold of 860. And you can see that this river routinely surpasses those thresholds in the winter, going upwards of 1,000, 2,000 milligrams per liter of salt.
And so if you’re an aquatic organism trying to live in this river, you’re constantly having your habitat change salinity, and so you’re being stressed out part of the year. If you think about species trying to evolve to this, it’s hard to evolve to a system that just keeps changing. This is data from the city of Madison. This is a urban sub-watershed that drains directly into Lake Mendota. And this is conductivity data from USGS. And this is measured in microsiemens per centimeter. But these concentrations that we see in the winter are about halfway to being ocean salinity. So the ocean’s about 50,000 microsiemens, we’re hitting 20,000 in some of this urban runoff in the winter. So a very salty environment. This is the same watershed in 2022.
And we can see that there’s a few days in February where it hits 30,000 microsiemens per centimeter. So again, almost halfway to being ocean salinity in these urban environments that are draining right into a freshwater lake. And so you can think about the lake diluting that, but that habitat right where the river enters the lake is gonna be a really hard environment to live in. And so what does that mean over time? Well, over time we’re seeing the concentrations of salt increase in our lakes. So this is data from three lakes in Madison, Wisconsin, Lake Mendota, Lake Monona, and Lake Wingra. And since the 1940s, which is kind of when we started using road salt, we’ve seen this steady increase in salinity. And this data set’s unique because we actually have data that goes back to before we started using road salt. And we know the chloride concentrations in these lakes sometimes were about one or two milligrams per liter. It’s rare to have data that goes this far back in time. And we can see that that is just continually increased through time.
There’s been a few times where it seems to level off and then continuing its pace. And the size of the lake matters too. So in this graph, Lake Wingra is the smallest lake, so it’s the most easy to pollute, so it has the highest salt concentrations. Lake Mendota is the largest lake, has the lowest concentrations because it just takes more salt to pollute it. And we can look at data across the state of Wisconsin and across the Midwest. So this is data from Michigan, Wisconsin, Minnesota, and we can see urban hotspots standing out as places with really problematic chloride concentrations. So the dots that are in sort of the green to yellow color are ones where we see really high chloride. And we can see the Detroit metro area stand out. We can see Milwaukee, Madison, Twin Cities as places where you can routinely get really high salt concentrations in our freshwaters. And then if we go to the Northwoods, we see a lot lower concentrations.
That makes sense, there’s less roads. Often it’s too cold for road salt to be effective, and so we’re still seeing sort of natural background concentrations in many places in the northern regions of these states. That being said, it’s not like it’s immune to problems. There’s certainly cases of lakes or rivers right beside a road that have high concentrations. There’s been instances where people’s shallow groundwater wells have also been polluted with salt. And groundwater is not immune either. So as we put salt onto the surface, some of that’s gonna percolate down into our groundwater, and that’s scary when we think about freshwater for drinking. So this is data from the City of Madison where they track chloride concentrations in their high-capacity groundwater wells, and they’re seeing some of their wells increasing in chloride over time. And this is a direct result of road salt runoff of the surface, sort of draining down into these aquifers. And these are really deep wells, these are high-capacity municipal wells, but if you have a shallow well, you’re even more at risk of having chloride contamination in your groundwater.
And the University of Wisconsin-Stevens Point actually has a great groundwater well viewer that you can look up online and see private well data for chloride across the state. And there’s a huge variability because it’s really spatially different. If you happen to be by a road, you happen to have groundwater flowing a certain direction, you might have a saline well versus someone on the other side of the street, their well might not be affected at all. So, how widespread of a problem is this? Well, there’s been a lot of studies done. This is one that we did a few years ago where we tried to look at long-term data sets of lakes across North America, and what we found is that lakes in urban environments were all increasing in salt, and lakes in sort of rural, forested environments were staying about the same. So it was very clear that this sort of urban land use is what’s leading to salinization. We also looked at the Lake Michigan water basin. So we had a postdoc here at the Center of Limnology who drove around Lake Michigan, sampling every stream that flows into Lake Michigan, measuring water quality. And we measured chloride in all of those samples, trying to predict what was gonna happen to the Great Lakes. And what we found was that, again, these urban environments had really high salt concentrations.
So especially around the Milwaukee area, Chicagoland, Green Bay, and then the northern part of the watershed had really low concentrations. And so what we’re seeing in the Great Lakes is that there’s been sort of a slow increase in chloride over time, but it’s relatively low because the Great Lakes are just a huge body of water that to actually increase the salinity of Lake Michigan, in this case, just takes a lot of salt. So luckily, the Great Lakes are staying relatively fresh, even though they are increasing slightly in chloride. So if we look across the Midwest and Northeast, this is a region that uses a lot of road salt. This is a climate belt where we have those temperatures between 20 and 30 degrees Fahrenheit a lot in the winter, and road salt is really effective. And so we’ve become dependent on using road salt in the winter to keep our roads safe. So we spend about $370 million for materials, so buying salt, and we spend over a billion dollars for actual application. So that includes trucks and people hours to actually keep our roads clear of snow and ice in the winter. So this is a very costly enterprise. This is also a region with a lot of freshwater.
A lot of regions in the United States have water scarcity, and luckily the Midwest and Northeast is not one of those. And so this is a region where we have to balance being dependent on road salt, but also protecting our freshwater environments. So we’ve done some work looking at how many lakes might be at risk, and so we were able to find a lot of data from certain states. There’s also other states that don’t have a lot of water quality monitoring for chloride. And we were able to build a predictive map where we actually could pinpoint what lakes we thought were at risk. And so there’s a number of lakes where we saw pretty high chloride concentrations and lots of lakes where we predicted that they would have high chloride concentrations. So we were able to predict this for about 50,000 lakes. And this helps managers go out and assess lakes that they might be responsible for and where they should be monitoring, and then potentially implement some better management practices for protecting those environments. So we’ve learned about what salinity is, how much salt we’re using, what we’re seeing in lakes and rivers, but what does this actually mean for lakes themselves? So I’m gonna hit on three points. We’re gonna talk about physics, chemistry, and biology.
So chemistry is kind of inherent. We’re adding chemicals to our lake, salt is a chemical, but what does that mean for lake physics? Well, lakes in Wisconsin, so especially deep lakes, go through this really interesting sort of annual cycle of mixing. So when the lake ice comes off in the spring, the lake is all about the same temperature, and the wind sort of easily mixes the water body. And this mixing of the water body is really important for the lake habitat ’cause it sort of redistributes nutrients and gases throughout the whole lake. So nutrients are brought up from the bottom, mixed to the surface. Oxygen from the surface is brought down to the bottom, and it’s sort of this reset in the spring. Then in the summer what happens is as the surface waters start to warm, that warming water is actually less dense. And so warm water will sort of float on top of colder, denser water in the bottom. And the lake actually stratifies into two layers, and we call this stratification. And so those two layers don’t really mix.
And what happens is we see buildup of nutrients in the bottom of lakes, but sometimes the bottom of lakes also lose oxygen because they’re not able to get new oxygen from the surface. And then in the fall when the lakes cool, what happens is the lake’s losing heat, the water becomes all the same density, and it mixes in the fall. And again, that mixing is really important. It’s bringing nutrients from the bottom back to the surface, those nutrients feed phytoplankton and zooplankton, and then gases are brought back to the bottom. So we want our lakes to have oxygen, we want that mixing to take place. And then the lakes actually stratify again in the winter under ice. And so we call these lakes dimictic, they mix twice a year. And this is an annual pattern that we see in all deep lakes in Wisconsin. Shallow lakes tend to mix just kind of all the time. And so this mixing process is sort of crucial for lake chemistry and also lake biology.
But what happens when you add salt? Well, when you add salt to water, it actually becomes more dense. And so saline water is denser than freshwater. So if we add salty, inflowing water into a lake, it’s gonna want to pool at the bottom of the lake ’cause it’s denser. So it’s gonna flow in and pool at the bottom. And this becomes a problem for that mixing phenomenon in the spring. So because there’s really dense water in the bottom, it takes a lot of energy to be able to sort of mix that salt out. And sometimes if you have enough salt at the bottom of the lake, it just won’t mix; you don’t have enough wind energy to really mix that salt back to the surface. And there’s examples of lakes in Michigan where they’ve had so much road salt runoff come in that they’ve stopped mixing. We call these lakes meromictic; they just don’t mix. And that causes consequences for chemistry because you lose oxygen and you have different chemical feedbacks.
And it also has a huge problem for biology, because if you’re creating these layers that don’t have oxygen, you can’t have any organisms living there. And so what we see is that in between this sort of mixing to no mixing stage, we’re gonna go through a phase where we delay spring mixing. So again, going back to our normal mixing patterns where we have a freshwater lake, when the lake ice comes off in the spring, we’re usually gonna mix that lake right away. So this is data that some students collected in Lake Monona in Madison, Wisconsin. They put sensors in the lake, measuring temperature and also conductivity. So they were able to measure both temperature and say something about salinity. And what they saw was that the bottom of Lake Monona gets pretty salty in the winter. And when the lake ice comes off in the spring, they actually didn’t find that the lake was mixing. They saw there was a 20-day delay in that spring mixing. And so that’s important.
That salt at the bottom of the lake is delaying that spring mixing, which then has feedbacks for things like the spring algal bloom and zooplankton populations, which then are important fish foods. So we have sort of food web effects from this changing lake physics because these lakes are getting saltier. So there’s a lot of really interesting feedbacks that sort of go beyond salt just being toxic to organisms. But salt is toxic to organisms, so it will change biodiversity. So one of the fears is that we’re not gonna kill all the organisms in a lake, but we’re gonna change what communities are present. And so we’re gonna change our phytoplankton communities. So we might shift towards more cyanobacteria instead of green algae. And cyanobacteria are a problem because they’re the ones that cause these harmful algal blooms with toxins. They’re also not as tasty to zooplankton. And we also might change our zooplankton populations.
So there’s a lot of research going on right now thinking about how community dynamics are gonna change as we increase salinity. And this is gonna differ between lakes and rivers. Going back to that data that I showed earlier, lakes are sort of slowly increasing in salinity, and there’s some thought that actually some of these short-lived organisms might be evolving to withstand some of those changes. But if you think about that river data, that salinity was sort of going up and down throughout the year to really high concentrations. And so you’ve gotta be an organism that’s able to withstand these really big swings. And so we’re gonna see different pressures based on the environment that these organisms are living in. So as I said, there’s lots of research going on in this area. So this was a study that was conducted with daphnia. So daphnia are zooplankton. They’re sort of the middle of the food chain.
We consider them keystone species. They’re really important for grazing on algae. And this study looked at daphnia in a soft water culture medium, and they added chloride to different sort of tanks to see what would happen to the zooplankton populations. And what they saw was that there was a big decrease in daphnia, the number of daphnia, at chloride concentrations between 5 and 40 milligrams per liter. So why this is important is that EPA chloride toxicity threshold is 230 milligrams per liter. So the EPA says that water bodies are fine if they’re below 230 milligrams per liter, even though the natural background concentrations in Wisconsin are 0 to 10 milligrams per liter. So what this study shows is that these thresholds might be way too high to protect certain organisms. In this case, concentrations as low as 40 milligrams per liter, we’re seeing big shifts in the food web, and so we might actually be really overestimating damages based on these thresholds. So this study was recreated using water from 16 different lakes around the world. So they were interested in, is this result transferable to other systems? Because all lakes have different background concentrations of ions.
And what they found was that pretty consistently in these 16 lakes, you took water from that lake and added chloride, you would see declines in zooplankton populations. But at the same time, you would see increases in algal populations. Because if you have less zooplankton, they’re not gonna be eating the algae, so the algae can grow better. And that’s a problem. We don’t want our lakes to be full of algal blooms. Zooplanktons are our friends, we’d like them to be healthy, eating algae. And so this study shows that as you increase salinity, you might actually risk having more algal blooms on lakes. So let’s think about what’s next, how can we solve this problem? My philosophy is this is a problem we can solve. There’s a lot of environmental problems that are really tricky to manage. We think about things like mercury in our lakes.
Well, mercury is bound up in organisms, it stays in the food web, it’s really hard to get rid of. We think of things like PFAS, these forever chemicals. How do we get rid of them? Well, salt is very easy. Salt stays dissolved in water, it will flow downstream, eventually reaching the ocean where it’s not a problem. So, what do we need to do? We need to use less salt. We need to put less salt into our lakes and our lakes and rivers over time will eventually become healthier again. So, how do we do that? So we have to think about management practices and education. So for a long time, we haven’t thought about salt as a pollutant. You can go to the store, buy a bag, throw it in your driveway, there’s no consequences. But there are consequences.
There’s consequences to the environment, there’s consequences to infrastructure. Our cars all get rusty. So, how do we manage that? Well, the state and some municipalities are starting to undergo trainings. They’re starting to calibrate their salt application, and a lot of private applicators are gonna be trained as well. So there’s lots of different levels at which you can start using less salt. You can start using less salt at your house. If you’re a business owner, you can request your applicators to use less salt. And a lot of this goes back to just education. We have to start thinking about salt as a pollutant. We can use a lot less and still maintain safe roads.
So one thing that the state of Wisconsin is doing is they’re really investing in liquid brine application. So instead of filling a truck up with salt, so solid minerals of sodium chloride, instead they take that salt and they dissolve it in water and they create a really salty, briny solution that then they spray on roads. And that brine solution is about 23% salt. So it’s still much saltier than ocean water, it’s very salty, but because it can be sprayed on, you can actually use a lot less salt. And so one way of maintaining good road safety and using less salt is this liquid application. So this is a map of the state of Wisconsin, county by county. So different counties take care of their own roads in Wisconsin. And this is from the 2021 Department of Transportation Annual Report. And on the left, we see a heat map of how much brine, so liquid application, different counties are using. So the counties in blue use a lot of liquid application and the counties in red use very little.
So those counties in red are still using that traditional rock salt. And on the right is a graph of how much salt that county used that year versus the five-year, long-term average. So this is actually a pretty dry year. So overall, most counties used less salt than average, but we see the biggest reductions in those counties that are using liquid application. And so we see counties like Jefferson County in the southeast, it’s a blue county for brine, but it’s also a blue county for its salt reduction. So Jefferson County uses a lot of liquid brine and they’ve actually been able to reduce their salt use by about 50% to 60%. So this is a major shift in how much salt we’re using. And if we think about how much salt the state of Wisconsin is using every year, so 500,000 tons of salt at $80 a ton, if we can reduce salt use by 50%, the state can save millions of dollars and we can also protect our freshwater rivers and lakes. So there’s lots of incentive to reduce our salt use. There’s also a lot of effort on the legislation side to try to pass limited liability legislation to protect people who use best salting practices from things like slip and fall lawsuits.
If you’re using best management practices, you shouldn’t be able to be sued as a landowner. So there’s lots of effort in the state going towards trying to reduce salt use and trying to protect people who make environmentally informed decisions when it comes to salt. So, what can you do to reduce your salt use? Well, here’s a great video made by Wisconsin Sea Grant on UW-Madison’s campus, focused on how you can reduce your salt use. We apply de-icer over a lot of the different impervious surfaces in the winter, so parking lots, roads, sidewalks. And as soon as that salt usually is hit with water, it dissolves and it goes anywhere the water goes. So in this case, it runs into storm sewers and straight into our lakes. In Wisconsin, we have a lot of freshwater. And as we start to have salt runoff from de-icers pollute those lakes, the concentration of salt starts increasing. And you can think of it like you think of yourself. If you eat too much salt, it’s bad for you.
We need freshwater to drink and to survive, and so do the organisms that live in the lake. So as we start increasing those salt concentrations, it starts hurting anything that lives and has evolved to live in freshwater. And the problem for humans really is that the lake is connected to our groundwater and we’re seeing our groundwater concentrations of salt increase as well, which means our drinking water is getting saltier. So the good thing about chloride and sort of salt pollution in general is that it’s definitely a solvable problem. It dissolves in water, and so as the lake flushes out, the concentrations will decrease. And so for us to lower the concentration of salt in our water, all we need to do is stop putting it in. It’s really that simple. – The key to keeping sidewalks clear in the winter is to manually remove as much snow and ice as you can using shovels and brooms before you think about applying salt. Using salt on top of snow just creates ice or slush, which you’ll have to clean up later. Another challenge is clearing snow during a snowfall or snowstorm.
When people walk on the sidewalks, the snow can become compacted and is harder to remove. The trick is to start mechanical snow removal early, right when it starts snowing, then work all through the storm. Clearing snow throughout the storm will stop snow from compacting. Temperatures usually drop after a storm, and sidewalks can become so cold that salt cannot work properly. Using more salt when it is cold isn’t going to work. If you need to clear compacted snow or ice, use an ice chisel or an ice scraper. – So often, if we get out early, we can shovel and remove ice and that takes care of a lot of the problem. Obviously, salt is necessary in certain circumstances, when it’s really icy out, but a little salt goes a long way. – So, how much salt should you use? Here’s a simple way to look at it. A coffee mug of salt is enough to treat an entire 20-foot driveway or 10 sidewalk squares.
So if your area is about five sidewalk squares, use a half a coffee cup. If it is more like 20 sidewalk squares, use two coffee cups of salt. If you can’t estimate the size of a certain space, make sure that you are spreading the salt so that there are no overlapping pieces. The pieces should be no more than three inches apart. You rarely need the salt spread more densely than this. A little salt goes a long way, so you only need to reapply in special circumstances, such as when snow is continually melting and refreezing. Other weather conditions will affect how and when you apply salt. If it’s a warm day and the sidewalk or steps look wet, you don’t need to apply salt because the sun is doing the melting. If it is an average winter day, salt will work fine to melt ice. But on a very cold, below-zero day, salt may not work.
To decide what salt to use, you need to consider the temperature of the air and the temperature of the pavement. Sodium chloride or rock salt works for an average day, but will not melt the ice if it is below 15 degrees Fahrenheit. Magnesium or calcium chloride works at colder temperatures, but won’t work below negative five degrees Fahrenheit. If you are using a blended de-icer, you will need to read the bag for application instructions. Wisconsin Salt Wise provides an easy-to-use application calculator that allows you to enter the pavement temperature and the type of salt you are using. Then it’ll tell you how much to use. Make sure to keep the salt fresh and spreadable. Store it in a tightly covered container because many de-icers attract moisture and turn into a solid lump if we keep them uncovered. If you have applied too much salt or if you spilled some, take a minute and sweep it up and reapply it to a different slippery area. – This problem of chloride pollution into water is really at this nexus of trying to maintain public safety while at the same time being really environmentally friendly.
And so there’s probably some middle ground where we can use a lot less salt and maintain public safety while still trying to protect our freshwater resources. [gentle music] So I hope you enjoyed that video. And if you wanna learn more about how you can do things at home to reduce your salt use, I would recommend checking out wisconsinsaltwise. com. It’s a statewide organization aimed at reducing salt use and there’s lots of great information on their website. So thank you for listening to my talk today, and I hope you learned something interesting. And your Wisconsin lakes and rivers thank you.
Search University Place Episodes
Related Stories from PBS Wisconsin's Blog

Donate to sign up. Activate and sign in to Passport. It's that easy to help PBS Wisconsin serve your community through media that educates, inspires, and entertains.
Make your membership gift today
Only for new users: Activate Passport using your code or email address
Already a member?
Look up my account
Need some help? Go to FAQ or visit PBS Passport Help
Need help accessing PBS Wisconsin anywhere?
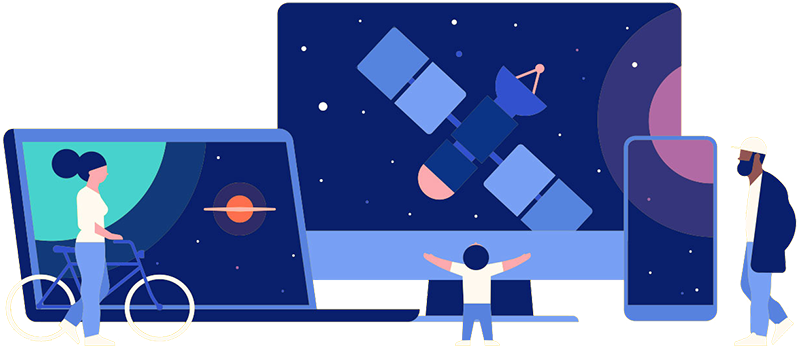
Online Access | Platform & Device Access | Cable or Satellite Access | Over-The-Air Access
Visit Access Guide
Need help accessing PBS Wisconsin anywhere?
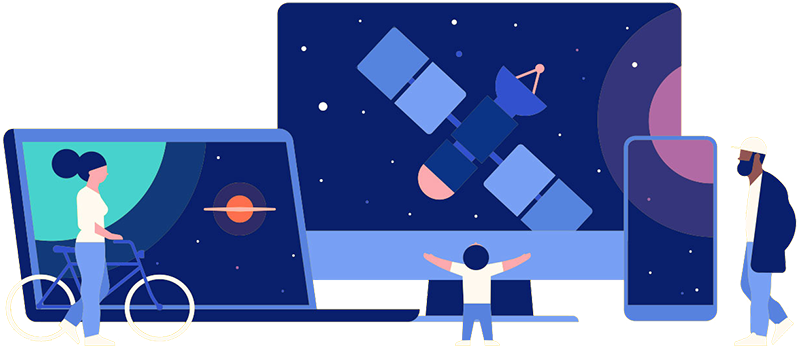
Visit Our
Live TV Access Guide
Online AccessPlatform & Device Access
Cable or Satellite Access
Over-The-Air Access
Visit Access Guide
Follow Us