– Jim Lattis: Thank you, I’d like to introduce tonight’s speaker, we’re glad to have Lena Vincent who is a third year graduate student at the Wisconsin Institute for Discovery. And she’s a pioneer in a new field. She’s come to Wisconsin from California to lead the University of Wisconsin into the field of astrobiology and she’s going to explain her role in this to us, but she’s part of a program that’s called OoLALA. One of the best acronyms anywhere, Origins of Life, Artificial Life and Astrobiology. So help me welcome Lena here. [audience applauds]
– Thank you Jim, so I just want to do a quick mic check for recording. Sounds good, okay great. Well I’m really happy to be here. So Jim invited me a couple of months ago, actually right after OoLALA came on the map. So we’ve been thinking about creating an official presence for astrobiology at UW-Madison for quite some time.
But that didn’t really actually happen until last spring, with our inaugural research showcase which I’m going to talk to you about. But when Jim invited me, I thought it’d be a really great idea to walk you through the establishment of this new initiative, the OoLALA initiative. But to do that I’m going to need to talk a little bit about what astrobiology is. You may not know. And even if you had heard about it, you may not be familiar with what the latest and greatest in astrobiology is right now. So that’s what I’m gonna start with today. I’m actually gonna start with a brief introduction to what astrobiology is as a discipline. And then I’m going to transition into what UW-Madison is doing in this context. So examples of research that are being conducted by scientists and thinkers at different levels on our campus. And then from there I hope that the OoLALA initiative will kind of justify itself and walk you through what the OoLALA initiative is, what we’re doing, and what our hopes for the future are.
So let’s start with a series of questions. So I think like any science, like any discipline, astrobiology is driven by questions. But I happen to think that the questions that underlie astrobiology are some of the most exciting and some of the grandest we can ask as human beings. And those include what is life? What are we? Why are we different from nonliving things? How did life get started here on Earth? How did that happen? It’s really difficult to conceive, and yet it keeps me up at night, it still does. Does life exist on other worlds? And if so, how can we detect it? How can we go to Mars to some of the icy moons or even beyond some of the exoplanets we’re now discovering, and figure out if life is there or has been there in the past. And then finally what is the future for life here on Earth and in space? So I think that those questions do a pretty decent job of summarizing the core of astrobiology. But if you like definitions, here is a popular definition that is often cited and is put forth by the NASA Astrobiology Institute which is the leader in coordinating and funding astrobiology research, at least here in the U. S. And it states that “Astrobiology is the study of the origins, “evolution, distribution, and future of life in the universe. ” So you can see it’s just the little questions.
So that is astrobiology. Now as you might imagine, if you try to think of what kinds of expertise you would need to try to answer those questions. You probably wouldn’t be able to come up with a single answer. And that’s because astrobiology is interdisciplinary to a degree that is rarely seen in science. So what I mean by interdisciplinary is that it requires a concerted effort from lots of different kinds of thinkers who have expertises and backgrounds in various different fields, both in the hard sciences and in the humanities, to come together and work on solving the mysteries associated with the origin of life and its distribution in the universe. And I think that’s captured there quite decently in that little figure I’ve included there, which just kind of shows all the disciplines that fall under the umbrella of astrobiology. But even that I don’t think captures quite the extent to which astrobiology is interdisciplinary. In some cases, even transdisciplinary. And also I’ll note that the humanities are not included in this figure, and I think that philosophy is a huge cornerstone of astrobiology as well. So because I’m going to spend the next 10 or 15 minutes or so going in and deconstructing that definition a little bit, and zooming in on some of the specific research themes that are currently being tackled by astrobiologists, I want to spend a couple more minutes here just dwelling on how cool this is.
Astrobiology has got to be one of the most exciting fields, in my opinion, I’m a little bit biased because I’m an astrobiologist. But just imagine being able to convert a natural curiosity, you’ve asked these questions to yourself before, maybe you still do. Being able to turn that natural curiosity, this deep existential need to understand where we came from and why we’re here, into a scientific career where you get paid by NASA or another organization to pursue this. Just how cool that is, I can’t even wrap my head around it. Another reason astrobiology to me is so exciting, especially now, is that the biggest discoveries in astrobiology are yet to be made. So we don’t have a second example of life yet, we don’t know if life exists on another world. There are a lot of optimistic people, myself included. And the other one is the mystery that is the origin of life on Earth. How did life get started on this planet? We just have no idea. You might think that that makes it kind of frustrating for astrobiologists, it totally does.
It means that we as scientists have an even steeper hill to climb in the scientific process. We don’t have more than one example of the phenomenon we’re trying to observe to draw conclusions the way you might in other fields. And then we also don’t have the luxury of being able to watch things unfold in real time. So unless you know something, I don’t know of a way of rewinding Earth’s history and watching life unfold before our eyes, right? So that makes it experimentally very different, but I also think it makes it super exciting. It means that we’re in this phase where we don’t know where we are. We’re at the frontier, we don’t know what lies ahead. But a lot of people like myself are very optimistic that we will make those discoveries and that it’s just a matter of time. And finally, I think also the fact that you get to work with all these different kinds of thinkers with different perspectives, with different backgrounds, makes it all that more exciting. So now I’m going to walk you through some of those different perspectives and show you some different research areas within the umbrella of astrobiology. And so one, of course, is when we’re trying to figure out where life came from, how life got started, whether it exists on other worlds, we have to understand how those worlds come to be in the first place, right? We need to know how the raw materials of living systems at the simplest level, even at the elemental level, how those get concentrated on what we consider to be planetary bodies.
So think of the Earth, this rocky body that has all this stuff on it, that somehow, some time in its evolution led to the appearance of life. So for that, we need to turn to this area of planetary science and astronomy that’s dedicated to understanding how planetary systems form. So when you have this cloud of gas and a star forms, how do these different clumps of matter accrete around that star to create planets. And what are the processes that drive the formation of these different kinds of planets? Some of which eventually will become hospitable to life. So that’s one area of astrobiology is this broad perspective on how planets form. How the context for the origin of life forms. A little bit more zoomed in than that, so once we understand how planetary systems form, we need to understand how a particular planet, in this case Earth formed, and what its early history was like if we hope to understand how eventually it was conducive to the origin of life. And so here what we have is an artist depiction of what we think the Earth looked like around the time when life emerged. So you may or may not recall that the Earth is about 4 1/2 billion years old, we have some arm wavy estimates for when the Earth became cool enough to actually have water on it, when you actually started to get plate tectonics, which is the process by which you have new material kind of being extruded from the center of the Earth and creating things like landmasses. So we have some arm wavy sense of what that was like, we really have no specifics beyond that.
And so a huge part of astrobiology is trying to understand the early history of the Earth. Trying to piece it together in the context of understanding when some of the major transitions that enabled life, like the appearance of water or oxygen, happened on this planet. So that’s one huge area and you tend to have people with backgrounds in geology and planetary science and geophysics and physics, all of that stuff to try to piece together this long and complex history. So now, we zoom in even further on the Earth, and we ask, so once you have this Earth, once you have water, once things start to become pleasant enough for living systems to emerge, how did the stuff that you need to make life, how did that start accumulating on a planet? So in the picture I just showed you, you didn’t see any life there, right, so we know for sure there were no living things. But what we’re starting to realize is that even though there were no living things present on Earth very very early on, that the stuff you need to make living things was accumulating very, very easily by probably a number of different ways. So one classic experiment that you may or may not have heard of in the past, maybe even in a biology course, is the Miller-Urey experiment. So what it showed is that you could make life’s building blocks, so some of the amino acids, out of simple ingredients like atmospheric gases and lightning. And when you pass this discharge through this gas mixture, you can start making life’s building blocks. And so this was a really seminal experiment to show that the building blocks of life could readily be made under early Earth conditions in the complete absence of life. Now since then, we’ve found many other ways by which this can happen.
So it turns out if you go down to the bottom of the ocean at these hydrothermal vent systems, they’re basically just giant factories for these building blocks. And likewise maybe even more elegantly, I find this so exciting, we’re realizing that this stuff is being flung at us from space all the time. So that’s a comet right there, we’ve had numerous bodies impact our planet. And we go and analyze them we find so many of life’s building blocks. Amino acids, some fatty acids that could form membranes, some nuclei bases, some precursors to some of the nucleic acids that we have in our cells today. So not only are there paths by which you make the stuff on a young planet in the absence of life, space is also just loaded with it. So that is really exciting, I think it inspired a generation of scientists to really look into this question of how you now take these things, these Legos if you will, and start assembling them into living systems. Now what I just showed you was a bottom-up perspective. So how do you go from the raw materials of the Earth that are not alive and you start incrementally approaching the complexity of life? There’s another approach which is more deeply seated in the biological sciences, which looks backwards in time. So it starts with a life that we know and see and understand today, and works backwards to look at life in deep time as it may have looked like in the early history of life.
And so some of the approaches that we use as astrobiologists are based on the evolutionary relationships between organisms that we see today. And that allows us to go back further and further in time and look at common ancestry. And based on some of the attributes of those common ancestors, we can actually draw conclusions about what environments might have been present on the early Earth and how they might have been making a living in that early Earth context. Then we can also look at analogs for early environments on the early Earth. Many of which were probably much more extreme than we’d be comfortable existing in. So here’s a picture of a hot spring. So there are many astrobiologists who do fieldwork who go out in these extreme environments and look at the diversity of living systems that live there and figure out how they make a living to try to infer backwards what the earliest ancestors of life might have been doing as well. And then another approach for looking at life in deep time is actually looking at the rock record and looking for physical evidence that life left behind throughout its history. And so what you have on the right hand side there is actually a very famous microfossil, some UW-Madison researchers actually helped in elucidating around what time, when this organism might have been alive. It turns out it’s about 3 1/2 billion years ago, which is a very long time.
But you can see that that’s still fairly complex, it looks like a string of cells, almost looks like a worm. That’s a pretty complex life right there and if that was already present 3 1/2 billion years ago, we have to imagine, when was the actual origin of life? It might be anywhere between 4. 2 to 3 1/2 billion years ago. So very, very far back. And so that’s an example of physical evidence that we can look back on. Now there is one limitation with this. It turns out our rock record is limited, so I mentioned plate tectonics and the fact that it kind of recycles and creates new crust by extruding material from the interior. It turns out that that’s a giant recycling system, it basically draws down all the stuff that was at the surface and buries it. So our rock record only extends back to about 3. 8 billion years ago.
So that means that between 4 1/2 and 3. 8 billion years ago, we have no idea, we have no record, it’s basically a blind spot in our record. So those are the life in deep time approaches, but as I just mentioned they have their limitations. So then there’s a complementary approach, and I’m partial to this one because this is the area I’m in. And this is to again work from the bottom up. So as I mentioned, we don’t have a second example of life yet. And if I had to summarize artificial life in one sentence, it would be that it’s trying to make those second examples of life here on Earth. Why would we want to make second examples of life? It’s kind of a fringy area. You might associate it with weird experiments and I’m sure there are a bunch of sci-fi plots based on similar approaches. But our rationale is that by making second examples of life, we can, along with the example of life as we know it, we can come to some generalities about what makes living systems the way that they are.
And it allows us to see how exotic life can get, what, are there different ways of being alive? And if so, what characterizes them, what do they have in common? So when we go to look for life on other worlds, we’re not just constrained to our perception of life as we know it, right. We might catch forms of life that seem very exotic to us because they are very dissimilar from life as we know it, and yet are alive by our definitions. So that’s the rationale behind artificial life, and there are lots of different ways of doing artificial life. So you may have heard it associated with artificial intelligence. So a lot of artificial life is in silico, so it’s based on computer models and computer simulations. So what you have on the far right side there is an example of a cellular automata, so it’s actually a computer algorithm that creates these little cells that obey simple rules. And you can get really complex behaviors out of them like evolution. So in some ways they can imitate aspects of life, and yet be in this gray area between alive and not alive. And then you have the wet A-life or the experimental A-life, which is literally trying to go into a lab and make second examples of life. So that’s the artificial life perspective.
Now I just mentioned that we really need to understand life as a general phenomenon to really be able to hope to detect it on other worlds. Because there is a chance that if we did find life on another world it would be very similar to life as we know it. There is also an equally strong probability that it would look completely dissimilar to life as we know it. And if we went to say Mars or Titan, or on an exoplanet when we have the capacity to do so in the future, if we went with our measures only calibrated to life as we know it, we might miss these very more exotic forms of life. So this is all part of this discussion around biosignatures. So biosignatures refers to artifacts or phenomena or objects that are smoking guns for life. And that involves sampling material from a planet for example, and deploying these measures and these observables and coming to some conclusion about whether those observables were the product of life or not. So biosignatures is very tricky, because it turns out, a lot of the biosignatures we have can be confounded by nonliving processes. And so that’s what a lot of biosignatures research is really trying to tackle, is finding smoking guns for life where we have almost 100% certainty that they must’ve been produced by life. It turns out that’s very hard to do, to my knowledge we don’t really have one of those yet.
And then if you add the layer on top of that, which is that life might exist in very exotic ways, then what do biosignatures mean in that context? So there’s a big push in astrobiology now for what are called agnostic biosignatures where you try to detect life based on some fundamental processes that life does without focusing on the specifics of what that life looks like, and that’s very hard to do as you might imagine. So that’s the biosignatures aspect. And now we look outwards again, now that we have the problem of biosignatures in mind. What do we do with it? So as you may or may not be aware, we are not alone by any means, and that may be true in terms of the life question, but we know for sure that we are not the only planetary system. And so this is a map, and I’m actually not sure how current this is, so we have a new exoplanet satellite that’s currently collecting data, TESS, and I don’t think that that data is reflected here in this map. But this right here shows 4,000 planetary systems that have been found in our surveys of the sky so far. And multiply that by the number of planets in all those planetary systems. So we have a lot of potential planets to play with here. And one of the ones that you may have heard of in the news was this Trappist system, and it kind of made headlines because it was found that some of these planets might actually be very similar to Earth in terms of their size and distance of the star that they orbit. So that’s really cool and that’s really exciting, and I think it motivates a lot of people to do astrobiology, just because when you look at this map, it’s hard to not be optimistic about the likelihood of life being somewhere else beyond Earth.
But there’s still so much we don’t understand, right? So I put the Trappist I system there not just to show you the systems that show a lot of promise, but also to caution you, because there’s a lot of hype about this habitable zone discussion, and I just want to say right now that there’s still so much we don’t understand about what makes anything habitable. And these systems look very different from the solar system we understand relatively well. And so there’s still so much we don’t understand. And we’re also limited by the fact that we can’t actually go to these places yet, so these are all observations that we have to make from very far away. And so that you can imagine that the biosignatures problem becomes even harder at that stage. So anyway, maybe I’ve dissuaded you a little bit about what can be done in astrobiology, because we’re faced with all these problems, but I think it’s really exciting. I just think it shows that we still have a lot of work to do. Like I said, it’s really hard to look at this map and not be optimistic about the chance of finding life. We just have to be smart about it. So with this in mind, I’m now going to transition over to what researchers at UW-Madison are doing to tackle some of these questions.
And with their permission, I gathered some information about what they were doing, and I tried to pick a selection of researchers that really represent the breadth of astrobiology. So I’m going to highlight five research groups and before I do that, I just want to pause and just mention that for a long time here at UW-Madison, until recently, we had the Wisconsin Astrobiology Research Consortium, which was a decade-long research consortium funded by the NASA Astrobiology Institute. And it was a team of 14 investigators, many of which were here at UW Madison, mostly in the Geological Sciences Department. And their main objective was to study methods for life detection. So not just look at the history of the Earth and how life influenced different Earth processes, but then how those can be used to design life detection methods. So I’ve summarized here their goals, and if you want more information about this, you want to see a collection of papers and the findings that came out of this decade-long initiative, I’ve put a link to the website there and I can definitely share that with anyone who’s interested in learning more. So this is no longer ongoing, so the funding for this expired about a year and a half ago. However, I think it created a strong legacy at UW-Madison, especially in the geosciences department, to continue in this line of research. And that brings me to Dr. Annie Bauer, who is an assistant professor in the department of geoscience, and what she’s interested in is reconstructing key transitions in Earth’s history.
So if you recall, I showed you that picture of the early Earth, and her question is really how did we go from this really hot, inhospitable planet to this really pretty blue marble we call home? And the particular transitions she’s interested in are the appearance and the evolution of crust, so landmasses. The oxygenation of the Earth and plate tectonics. So how did those happen, when did they happen. And the key message she uses is geochronology. So she basically dates rocks. And she does that by looking at these zircons. So zircons, you may be familiar, they’re this very beautiful gemstone. But in their raw form, they are a really cool mineral, because it’s one of the hardiest minerals you can find. So that means that when these zircons formed, even 4 1/2 billion years ago, they’re going to stick around. The cool thing about zircons is that the way that they grow tends to trap material inside the crystal.
And they therefore lock in a bunch of really interesting geochemical information. So what she does she actually looks at these zircons, she collects them from different localities, so she was kind enough to share pictures of her working in the field. So this is a gneiss complex, so gneiss is a kind of rock. And she extracts zircons from those, and that formation is actually 3. 9 billion years old. So she collects these zircons, and then she uses a method called laser ablation to kind of peel back the layers of the zircon, and then uses isotopic methods to actually date those layers. So if you’re not familiar with isotopes, you basically look, in a very general sense, just look at the distribution of those isotopes and based on the ratios, you can tell when and how particular processes might have been happening. So that’s what she’s doing, so she is using these zircons and these analytical means to study these transitions and reconstruct them based on her data. So then to shift gears a little bit, we also have this question of life in the clouds of Venus. Now, this is an area that is being spearheaded by Sanjay Limaye, who’s a research scientist at the Space Science and Engineering Center at UW-Madison.
So Sanjay has been working on Venus for a long time, and he has this really cool hypothesis that the clouds of Venus might be hospitable to microbial life. So this is actually not a new idea, it’s been around for a long time, dating to the late 60s. But there’s these mysterious dark clouds, you can see them there on that image, and they’re very mysterious, they think that they’re mostly made of sulfuric material, but the height at which they are in the atmosphere and the temperature that characterizes them and the kinds of materials you find aerosolized in that layer, make it kind of hospitable to microbial life. And so Sanjay released a paper last year where he compared some of the light absorbing properties of different microbial proteins and the light absorbing properties of these clouds and they’re oddly similar. So he has this cool hypothesis about life being possible in the clouds of Venus. And this is a concept here for a platform, a maneuverable platform that would go to Venus and actually sample these cloud layers and see if there’s life there. So this is called the VAMP. And so I just thought I would note that a graduate student who is actually involved in OoLALA, Jaime Cordova, will be going to Moscow later this year to try to pitch this VAMP concept to the Venera-D mission to Venus. So that would be really cool. Okay, so then we’re going to switch gears a little bit more and now talk about the origins of biochemistry of life as we know it.
So John Yin’s group over at the Wisconsin Institute for Discovery has been doing work on this, and here I’m highlighting Hayley’s work, who is a graduate student in the chemical engineering department. And what she’s interested in is figuring out how the complex processes of life got started. So we know that cellular life today is a very complex thing. It requires this orchestration of really complex processes. And the one she’s particularly interested in is the information processing and the energy processing features of life. And she is particularly interested in those because it’s this chicken and egg problem. So you need energy processing to transfer information, but you also need that information to feed back and influence those energy harvesting processes. So she’s interested in how that got started. How could you get one or the other, was that a sequential thing, did they have to emerge simultaneously? So what she’s doing is she’s looking at a particular process in cells, which is peptide formation, so proteins are kind of a cornerstone of biology. They do everything in the cell, and the way that they form is by stringing together these amino acids, these beads if you will.
In cellular life today, that’s carried out by very very complex processes, things like the ribosome. But she’s interested in how this process might have gotten started on the early Earth. So what their group is doing is they’re looking at a process that happens in natural environments as a driving force for the creation of these peptides in the absence of complex machinery like the ribosome. So what they found is that wet-drying cycle, so that repeating dehydration and re hydration that you get in something like a hydrothermal pool, might actually drive the formation of these proteins in the absence of complex life. And so I won’t go into this in detail because it’s a little bit beyond even my comprehension, but being able to show that using this wet-drying process and by adding different conditions and different energy sources, that you can create these chains and that you can actually encode information in them. So you actually get biases in the kinds of proteins you get depending on the environment that they’re formed in. That’s really cool work. And then again switching gears, so Simon Gilroy actually came to give a talk here a while ago, but I thought I would highlight his research again. So Simon is a professor of botany, and what he’s interested in is basically this question of surviving in space, right? So we have the ISS right now, but we also have all these plans to maybe go and colonize Mars and set up long-term colonies on the moon, and then eventually go farther and farther out in the solar system. But there’s this big question of how will we survive, how will we sustain ourselves? And wouldn’t it be cool if we could actually grow crop-producing plants in space? And so he’s been studying how space conditions actually affect crop- producing plant growth.
So he was kind enough to share the slide about some of the upcoming missions that they have on the ISS that are due to launch I think and he said about nine months from now. And so y’know they have all these tests where they’re actually going to analyze how cotton grows in orbit. Develop new kinds of chambers and instrumentation to enable this. So I think that’s a really cool sampler of the different ways in which UW-Madison researchers are contributing to astrobiology. And of course because I’m here and I have the opportunity to plug my own research, I’m just going to talk about our group a little bit. So I’ve shown here our PI, principal investigator David Baum, who’s also a professor in the botany department. And then our team of really talented undergraduate students. And I’ve chosen to highlight here our collective expertise. So you can see the extent to which we are interdisciplinary even within our own research group. And the question we’re trying to ask by the way is a pretty– is not a trivial one, we’re interested in the origins of evolution.
How did evolution start? So we have a model that we’ve built where we think that evolution starts very early, it’s probably one of the earliest processes to have arisen at the origin of life. And so we’re conducting laboratory experiments that, summarized very briefly, are akin to just creating mini early Earth conditions. And then looking for evidence that evolution is occurring. And so we have some sense of what that should look like. And I’m not going to spend too much time here, but if you have questions about that please feel free to ask me because I’d love to talk more about it. But all I want to say is that we’re finding really, really cool results. So we mix minerals and prebiotic soups, and we look for evidence of these cool processes happening. And we have some really exciting tantalizing results. So anyway, now with that, I hope that you get a really good sampler of different kinds of astrobiology research that are currently being done, and that is a small subset by the way. I only had time to go over five.
We currently have 22 self-identified groups that are doing astrobiology relevant research at UW-Madison, and the list keeps growing. So with that now I want to talk about the OoLALA initiative. So what is a OoLALA? So you know it stands for origin of life, artificial life, and astrobiology. So I cannot take credit for that acronym, that was actually my PI David Baum’s idea, which is a superb acronym. And so you OoLALA is an initiative that’s centered at the Wisconsin Institute for Discovery, which is a public research institute. And our goals are to organize and support this community of astrobiologists here on campus. And we are doing that by creating programming and resources to help coordinate and highlight research on campus and to draw awareness to astrobiology and the fact that there are so many people at UW-Madison doing astrobiology research. And we’re also trying to provide resources and support for students who are coming into science and who are seeking out UW-Madison because of their interest in these fields. Which is kind of a new thing. Most astrobiologists that are in business today either made careers doing something else, and this was an interest that they developed later on.
Or they’re like me, and they’re joining astrobiology relatively early in their careers but started out doing something fundamentally different, in my case I was a stem cell biologist before I did this. But we now have undergraduate students and young students who are coming into science because of their interest in these areas. And we find that not just on this campus, but in general, there are a lot of resources lacking to support them. So those really were the three missions that we were trying to accomplish with the establishment of OoLALA. So the first concrete way that we decided to do this is by launching a website. So this is our website, astrobio. wisc. edu. It’s hosted by the Wisconsin Institute for Discovery and it’s still under construction, so we’re still trying to find different kinds of content to put on there, so if you have any suggestions that you feel would be helpful, I’d be very open to hearing those. So this is our website here.
And our goal, very generally, was to consolidate all of this amazing research that’s being done related to OoLALA under one roof. So when I was a graduate student, when I knew I wanted to pursue grad school, I knew I wanted to do astrobiology. But there are very few places, there are very few institutions where there are dedicated astrobiology departments. In fact, I don’t know of any. So that makes it very hard to find out who is doing astrobiology research. And so what we thought would be really useful is to create one place where you could go and find that information, even if there was no formal department to which it was associated. So that was a main motivation. And so one of the things that we decided to do was create an about page. So you can’t read anything here but I encourage you to go check it out. It just has a summary of what astrobiology is, what astrobiology at UW-Madison means, and how to get involved.
So giving some pointers to young students who are maybe interested in pursuing astrobiology research, but aren’t sure how to do that. This would be a place to start. And then of course we have our research page. So we have compiled a list of research profiles of UW-Madison faculty and research groups that are doing astrobiology relevant research. And we have organized them based on the kind of discipline that they belong to. As I said, we currently have 22 listed on there, but the list keeps growing. And so this is a really great place for someone who has a particular area that they’re interested in, say atmospheric science. Who wants to find what researchers at UW-Madison are doing atmospheric science with an interest in astrobiology relevant questions. And so then one of the other concrete ways in which we are trying to accomplish the goals of you OoLALA is through programming. So this is a research showcase that took place last spring.
It was funded by the Women in Science and Engineering Leadership Institute, and the Wisconsin Institute for Discovery. And our goal was to highlight advances in astrobiology research through the lens of these four researchers who came from off-campus, and who gave hour-long public lectures at the Wisconsin Institute for Discovery and who stayed here two to three days to interact with members of the UW-Madison community. And so that was extremely successful, again we try to have speakers come in who represented the diversity of different fields and perspectives in astrobiology. So we had Dr. Sarah Walker from ASU come and give a really awesome talk on the physics of life and trying to elucidate the general physical rules that underlie life and its emergence. Then we had Dr. Amanda Garcia who is a postdoc at the University of Arizona, who uses reconstruction methods to study ancient genes in bacteria and microorganisms to study the co-evolution of life on Earth, particularly in the early stages of life’s history. Then we had Dr. Kate Adamala come, who does really exciting work on building basically fake cells, synthetic cells for a number of different applications, including studying what the first cells might have looked like. So trying to make artificial life.
And then finally we had Dr. Sarah Horst come, from Johns Hopkins University, who gave a fantastic talk on her research on Titan’s atmosphere and some of the really cool prebiotic chemistry that happens on Titan. And as a special bonus, Sarah is actually a co-investigator on the recently selected NASA Dragonfly mission, and so she talked about that even before it was selected by NASA, it was actually a few months before that. So that was really exciting, I’ll get back to that in a second. So this is just to show that the showcase was really successful in terms of its reach, so we actually got a lot of attention including from the NASA Astrobiology Institute, who took great interest in this series. And overall, we thought it was a really successful and useful way to connect these pioneers in their fields, interact, and make contact with the UW-Madison community. And hopefully inspire some young students to consider pursuing careers in this area. So then again I just want to boast this, because we had the privilege of having Sarah Horst come talk about the Dragonfly mission before it was even selected. So the fact that it was selected is even more exciting. So this is basically this quadcopter that they’re going to send to Titan, and it’s going to travel to a dozen different locations and sample different areas for pre-biotically relevant compounds.
So this is truly an astrobiology mission and it’s due to launch in 2026 I believe, and we’ll be getting hopefully the first stuff back in 2034. So more than a decade away, but still really exciting to think that we’re going back to Titan and so very high hopes for that. And here’s just a closer look at what that quadcopter is going to look like. So it’s basically going to fly around, so it’s going to take advantage of the fact that Titan’s atmosphere is much much thicker, it’s basically just going to glide around and move about and find all these really interesting sites and then sample them. And then I also want to mention that if you want to learn more about Dragonfly and more about all the talks that happened as part of the showcase, you can go to our website and all of the recordings are posted there. So even if you couldn’t make it, or if you know of someone who might be interested, please feel free to navigate there. All the talks are for a general audience and they were all really cool, and I think they did a really good job just representing just how broad astrobiology is. So that’s actually all I have. But I just want to plug again our website, and I just wanted to put our email addresses here. So that’s me, and that’s Jaime Cordova, so that’s his email there.
So he’s our social media director, we have a Twitter account, and he is also a great resource. He is also a JPL Solar System Ambassador, so he knows a lot about upcoming space missions, so if you’re interested in that, he’s a great contact. And yeah, so I’m happy to take questions now. [audience applauds] of those common ancestors,
Search University Place Episodes
Related Stories from PBS Wisconsin's Blog

Donate to sign up. Activate and sign in to Passport. It's that easy to help PBS Wisconsin serve your community through media that educates, inspires, and entertains.
Make your membership gift today
Only for new users: Activate Passport using your code or email address
Already a member?
Look up my account
Need some help? Go to FAQ or visit PBS Passport Help
Need help accessing PBS Wisconsin anywhere?
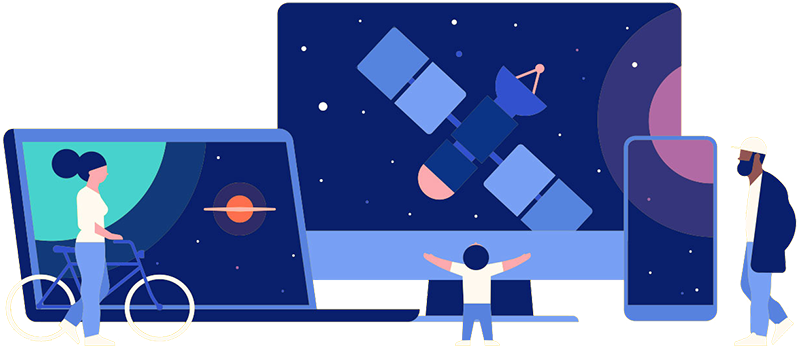
Online Access | Platform & Device Access | Cable or Satellite Access | Over-The-Air Access
Visit Access Guide
Need help accessing PBS Wisconsin anywhere?
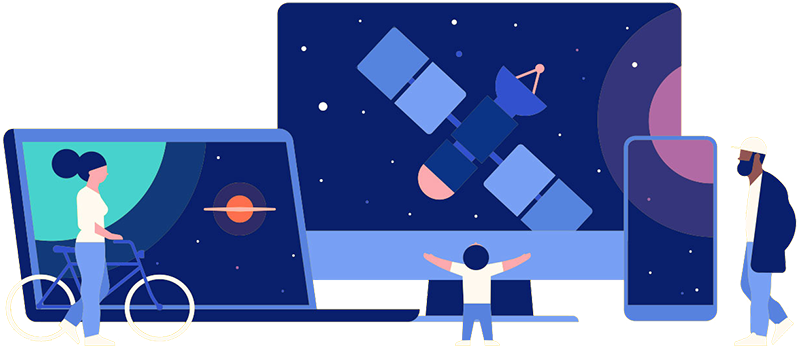
Visit Our
Live TV Access Guide
Online AccessPlatform & Device Access
Cable or Satellite Access
Over-The-Air Access
Visit Access Guide
Follow Us