– Renee Meiller: Hello, everyone. Here’s a fact that might make your brain hurt. When you receive a traumatic impact to your head, your neurons start dying off in just six hours. And over the next 45 minutes or so, today’s speaker will tell us why that’s important. I’m Renee Meiller. I’m the communications director for the College of Engineering and I’m a member of the Round Table Planning Committee. On behalf of the committee, I’d like to welcome you to today’s talk. A number of UW-Madison sponsors helped make this event possible. They include the Office of the Chancellor, the Office of the Secretary of the Academic Staff, the Wisconsin Union, and the Office of Learning and Talent Development, so we thank them for their ongoing support. Our speaker today is Christian Franck.
Christian is the Granger Institute for Engineering Associate Professor in mechanical engineering, and he joined UW-Madison in fall 2018 after several years on the faculty at Brown University. Christian is kind of a pilot at heart. He earned his undergraduate degree in aerospace engineering, and while he was working toward his PhD in mechanical engineering at the California Institute of Technology, he also earned his pilot’s license. Today, however, Christian studies the many ways that mechanical forces can affect cell biomechanics, and his work transcends disciplinary boundaries. He seeks opportunities to work closely with neuroscientists and other experts to bring his expertise in mechanics to the study of the brain. Currently, he is leading a multimillion-dollar, transdisciplinary initiative aimed at understanding how impacts affect the cells in our brain. Christian, welcome. [audience applauding]
– Thank you. Thank you Renee, and thanks everybody for coming. It’s a real pleasure and honor to be here.
But big part of what I’m gonna tell you about today is the story about how we think about, how we analyze, and how we hope to be able to prevent any cells in the brain from dying off by better understanding the origin of concussions, the origins of traumatic brain injury, and how we can use that information to think about better ways of protecting people that are at risk of these kinds of injuries, as well as new strategies of equipment or personal protective gear that we might devise. I wanna say a few things about the title and then some things that happen in the tile slide. I’ll say a few things about sort of my coming to this. I’m a little bit of a oddball in this. As Renee mentioned, I trained as an aeronautical aerospace engineer. I went to graduate school very much thinking I was gonna keep working on the next generation of passenger or military aircraft. Somehow in the process of graduate school, I lost my ways and I got very interested in cell biology, human biology, and human disease. And turns out that every day, from chewing to walking to fighting off colds and infections, mechanical forces play a significant role in the human body. And this tale and this story about head injury and concussions is yet another manifestation of that. I think it’s an obvious one; typically brain injuries, neurodegenerative conditions and diseases in these cases start with an insult to the head and it’s mechanical in nature.
And what I found when I sort of entered this field about 10 years ago was that there was a lot of wonderful work being done by the biological and neuroscience communities, but one part of it, which was this interface to the mechanical engineering communities was sort of missing. And that’s the piece where I entered. That’s part of my background, and then as I was entering this, I realized quickly that given the complexity of the brain, given where we are today with our understanding of, especially neurodegenerative diseases. A lot of the headway that we’re making now, and a lot of the headway that’s gonna be coming in the next few years has really happened because of two things: one, because of recent advances in technology, and the other thing of really people coming together and communities blending. This is certainly a problem that not a single person can attack and solve on their own. And so certainly I have benefited from the many interactions I had with my colleagues, the people in the community to work on this problem together. So I’ll talk a little bit about what we have going on now, and kind of the footprint that we have established and some of the vision, and then hopefully I can guide you through it and kind of explain some of our thinking. So to reconcile with my title, it’s Defining Neuromechanics: Concussions and Traumatic Brain Injury. This idea of neuromechanics is a subcategory of, it’s blending of neuroscience and mechanics of material, which usually falls under the purview of mechanical engineering right, so this is the interpretation of mechanical forces in the context of cell biology or the brain and neuroscience. And again, to do this in an interdisciplinary setting where we try to get as many experts as we can, work together on this problem constructively.
We have built this center now, program that lives under the acronym of PANTHER, and I’ll tell you what that stands for. We’re currently supported funding-wise by the Department of Defense, primarily the Office of Naval Research. There’s a lot of work that where the Department of Defense worries about the war fighters and making sure they are protected as good as we can, but at a basic scientific level, this extends all realms of occupational risk to the brain. Okay. So this is who we are right now, again when I started this, this was my vision was to come, you know, have a program where people can work together freely, a program that’s fully transparent and fully accountable, where anyone from the community can have access to see what people are up to, see what kind of business is being done and see how it matters to relevant communities. We started with just five of us. I’m currently the acting director of this program. We come from different backgrounds. You can see different universities are represented here. We’re about 12 in all, mostly spanning academia.
We have a couple of companies here. So Ron is working for Team Wendy, they are a helmet liner and manufacturing company. We have Tony who runs the Helmet Division at Trek Bicycles here in Wisconsin, and then we had some representation from Milwaukee Tool Company, who are very much interested in designing the next generation hard hat for construction workers to keep them safe. Funding is provided by through our program manager at the Office of Naval Research, and that’s sort of the composition right now. It’s essentially like many gears in a very complex machinery that all come together and work together. I’ll touch a little bit upon the different aspects that some of the people do; it’s gonna be a high level, but I’m accessible for after the talk or afterwards if you would like to learn more about each of us or contact us. Today, I’ll focus about the work that we do here in my laboratory at the University of Wisconsin-Madison, and it will highlight a few snippets from work that’s done by Rika Wright Carlsen at Robert Morris University. She’s helping us to try to understand what kind of motion that the head undergoes is most injurious to the brain. Okay, and this comes back to understanding situational awareness, this comes back to helping coaches and trainers on the field trying to eliminate those kind of situations, that kind of motion that then becomes most injurious to the brain. Similarly, we’re trying to use that information as well to see what kind of protective equipment, what kind of engineering solutions could we send out to protect people from that.
Okay. So this slide is extremely busy, it is not meant for you to actually read, but I just wanna focus your attention on the center circle. This was came from a very large-scale poster that we presented, and I just wanna highlight here the name. So it’s an acronym, but it’s a sort of a very, you know, convoluted acronym for PANTHER. It stands for [laughs] exactly, it stands for Physics-bAsed Neutralization of Threats to Human tissuE and oRgans, and you know, you may get a kick out of this, but when you work with organizations, especially within the Department of Defense, acronyms are very important, and usually you choose an acronym that depicts an animal, okay. So we went through several different ones. We settled on PANTHER. We have a logo, so the point is this is a center. This is a research hub for people to come together to address concussions and the origin of concussions, especially the cellular origins and ways we can mitigate it, ways we can learn about it, and ways we can prevent it. And this is sort of showing you in this inner circle.
So the first upper left hand quadrant is the cell-level, tissue-level work that we do here and that people do at Brown. I’ll talk about our portion here in a little bit, but there’s a larger space where people do anatomically realistic simulation of head motion, and I’ll show a couple of snippets of that, and then over here is the realm of next generation material development for head protection, okay, and this space primarily right now is centered around helmets, but in the future it may have other products as well. Okay. This gives you another sort of a more linear progression of the kind of work that we in the in the PANTHER program do. This sort of middle line right here is powers of 10. It gives you a size scale of the things that we encounter in our research. Far left here, this actually is, this little video that plays is a degenerating neuron. So that’s a brain cell. That’s real data taken in the laboratory. This is out of network of millions of neurons centered around one, and I’ll talk about this.
This is the sort of six to eight hour degeneration breakdown cascade that Renee was alluding to that can happen if the insult to the head was severe enough. Down here is sort of the next, so the next level up evolution of kind of what we can do in the laboratory, this right here, looks as a spherical cortical brain ball essentially, and so what we can do these days with tissue engineering and you know, engineering solutions, we can start to construct tissues that approach you know, some of the complexity of the native brain, okay? So we’re not quite there where we can make real brains that have same degree of anatomical realism, but we can get quite close. The caveat with any of this is that at any given day, any given experiment in the laboratory, we’re limited to how much data we can extract, okay. So we’re limited by the number of readouts. so it’s easy to increase the complexity, but it’s very hard to decipher the mechanistic and the causal relationships between every single player with the network. So what we do right now is we work mostly here. We’re trying to understand what is the root cause of traumatic brain injury? Specifically, we ask the question, What is, what are the limits on force? What are the limits on deformations, we’re gonna be phrasing it in terms of stress and strain. What is the limit at which point the cell decides, “I’m done. “I’m just shutting down chemically,” or “I’m being torn apart physically. ” We then wanna use this information and we wanna be able to put it into computer simulation algorithms that we can use that could be cloud-based.
Now these days, a lot of talk about machine learning and artificial intelligence. All these are available, and we wanna connect this back with life measurements that we could instrument players, we could instrument helmets, we could instrument vehicles, whatnot to make sort of on the fly, real-life diagnostic assessment of the state of health of the brain tissue. And then the last two pictures here are the much larger scale. Again, this is advanced material development. The idea is to, once we understand what kind of motion, what kind of forces are most injuriious to the brain tissue, we wanna come up with engineering solution that mitigate that. So that’s sort of in a nutshell of what we think about within this program, okay. So today, I’m gonna tell you a little bit. We’re gonna go inside the brain. We’re gonna look at the brain tissue, and we’re gonna talk about how we think about and how we look at the initiation of these injuries. Concussions are part of the umbrella of traumatic brain injuries, so that will be included, and I’ll talk a little bit about this as well.
Okay. So what I’m gonna have to do as we kick this off, I’m gonna have to give you a little bit of vocabulary, so you’re gonna have to just hang with me here. ‘Cause the problem is like when you blend fields together, you get a lot of language that comes with it. So I’ll try to do my best to keep it digestible. And then at the end when I’m done with my talk, you can grade me and you can tell me whether I did a good job or not. But at a very high level, there are sort of two distinctions. There’s something that we have referred to as a primary injury and secondary injury when it comes to traumatic brain injury. I should say at an even higher level before that there is a severity grading of traumatic brain injuries that go from mild to moderate to severe, and they’re typically ranked on the Glasgow Coma Scale that was developed many, many years ago. So let’s look in both neurosymptomatically, how’s a person responding after an accident, and how is the sort of the state of health of the brain assessed by something like magnetic residence imaging here, MRI or CT scanning for example, in an ER. Now in this case, when we talk about primary injury, it’s really immediate, physical damage that is a result of that strike to the head, a fall, an acceleration, an explosion, whatever the cause was.
And it’s a physical discussion, okay? The secondary injury is the one that’s much more subtle, and it’s a change in the chemistry of the cell, okay? So this is something where yes, there was a sudden mechanical change in the environment of the cell. The cell is being pulled or twisted or sheared, but not quite enough to break it apart fully, not quite enough to rupture capillaries or vessels, but enough to change the biochemistry in the cell. And when you do that, you activate a whole bunch of chemical reactions in the cell and then eventually, the cell comes to a decision point, and the decision point is simple. “Can I still repair myself, or am I past that point “and I need to shut down?” And when the cell decides to shut down, we’re following this classical cascade of what we call apoptosis, in some cases also in necrosis, it depends on exactly what chemical species are involved. And that process, typically on a cell level, takes about anywhere from 6 to 24 hours, okay? But that’s a single cell. We have billions of them in our brain. A single cell is gone, cells live within networks, networks would give us function, cognitive function, motor control, speech control, right? So we may be losing a single cell there, some rewiring of that network, restructuring, maybe we’ll never know. If you lose enough cells within a network, that network becomes compromised, and then most certainly we will see a deficit. Could be a cognitive defecit, could be a speech deficit, could be loss of motor control. We want to be very wary and aware of if there are changes in the biochemistry internally.
So this is something where we have to track both immediate damage given by the primary injury as well as this developing secondary injury. And there is some beautiful work that’s actually being done up at Boston University by a UW alum by the name of Ann McKee, and she has really done some pioneering contributions in this field. There is an image right here, and what you’re seeing is these are cross-sectional slices of brain tissue, there is sort of three rows. The first one is a person with no documented concussions or falls, and you can see it looks clear, and down here, are these sort of staining patterns. That’s something that would you expect from normal tissue. The second two, the second and the third column shows brown staining, and the brown staining here is indicative of accumulation of a protein called tau. Tau clinically is tied to a disease or something called tauopathy, which is involved in several neurodegenerative diseases, including dementia, including Alzheimer’s, including Parkinson’s. Some of the links are still being worked out exactly to what extent, but that is significant levels of degeneration that happened in these brains, and you can see here that the line is, so this was an NFL linebacker who had eight, and I will say eight reported concussions. That’s another really big thing, and I’ll tell you in a little bit too the problem with concussion as a metric for injury. We have another problem with that.
I’ll just say it right now. Diagnosis of concussion is neurosymptomatic, okay? What that means is it relies on a doctor on the field to run a number of tests to make an assessment whether or not a concussion has happened. That does not mean that physical brain trauma did not happen. And in fact, a study published by Ann McKee’s group a few years ago showed that almost 99, or a very high percentage of NFL players, all had significant brain trauma, which ended up manifesting itself postmortem into what was known as chronic traumatic encephalopathy, known short as CTE. The problem with that is that it’s something that at current, we don’t have the tools to diagnose on the field, on the spot, on the fly. So part of our effort here in Wisconsin with the PANTHER program is to have this kind of diagnosis. Is to say that like we don’t wanna rely on just the manifestation of a concussion to tell us that a real physical injury has happened to the brain. The implication in the military realm is even more complex, given the kind of workload that warfighters have to sustain and the decision making process they have to do. Again, there is significant brain trauma, there was significant signs of CTE, but only eight reported concussions, okay. So there’s definitely a problem with this.
It’s a problem with the reporting in itself. And so big part of what we wanna do with the research here is we wanna move away from classification of injury that’s based on the symptoms. We wanna go down and classify based on real structural changes that happen at the tissue and the cellular level. Okay, it’s hard, it’s a hard task, a challenging task, but I think we’re making good strides to get there, okay. So this just gives you an orientation, so we’re gonna be tracking this vocabulary, primary and secondary injury, as we go along. I wanted to introduce the protagonist of my story today. The first one is gonna be, so the key player that we spend most time thinking about, and that is the neuron. Again, the neuron and the neural networks in our brain are one of the main units that give us our cognitive abilities. What you see here is actually, this is a rendering of a real-life image taken with a microscope in the laboratory. It’s color-coded for what I will show you later is the level of compressive loading strain during an impact, and you can see white colors mean high values and darker colors mean lower values.
And when we looked at this, we wanted to understand what does a cell see, what does a cell experience during an impact, during a blow to the head? So I’ll come back to this, but this is one of our key players. The other thing is the notion of what we call stress, strain, and strain rate. So a very simple, in very simple terms, if you take a rope and you pull it, to pull a rope takes force that you have to exert, like a tug of war. If you divide that force that you’re applying by the cross-sectional area of that rope, you get something that’s called stress, and it’s a quantity that any mechanical engineer works with daily because that’s how we design buildings and how we design structures, cars. Turns out biology and evolution is also heavily driven by these terms of stress and strain, and strain is nothing else but the resulting change in shape that the force of the area causes, right, so if I take a rubber band and I pull it, the amount of force that I did in my hands to pull a rubber band, give an extension, and the level of extension normalized by its original length is strength. So it’s a percent of shape change essentially. And then you can imagine like in the complexity of the brain, these things get a little bit more complicated, and we try to make sure that we track that carefully. But that’s, so we wanna quantify the level of stress and strain that’s being loaded or that the neuron is subjected to, and then we wanna track the biological response of that neuron in response to that. And the last thing that we have here is time, okay. We wanna understand time, and time comes back to, really the biology, it comes back to the chemistry, the change in the internal chemistry of the cells, chemical reactions, where we find if we have some time before degeneration occurs in the brain.
Whether that’s four hours, six hours, eight hours. First of all, we wanna know about it. Second of all, if the time is long enough, it gives us a window of therapeutic intervention. And what we show in the laboratory where we have all the access in the world because we get to play with the tissue directly, we have shown that, for example, one good intervention is cooling. So if we cool these cells, we can actually like shut down a lot of those neurodegenerative cascades. How you implement cooling on a person and a human being, that’s a discussion and a question for another day, but at least like we wanna understand the role of time in the injury response of these cells. Okay. Now, the objectives here for us and again, this is just bringing back what I’ve said. It’s, we wanna understand what are the critical injury thresholds in terms of the quantities of stress and strain. In the laboratory, we like to work with strain simply because it’s a change in length, a change the dimension.
It’s easier to measure internally. In the olden times when you step on a scale, what would happen is our weight compressed or extended a spring. And seeing that visually is much easier than knowing what the spring constant is and backing this out and calculating force and stress. So we typically work with strength. Models can help us to get the stress, and then we wanna also understand strain of rate. So that’s the loading rate. That’s slower versus fast, right? Is a slow fall or a slow blow to the head versus a really fast strike to the head, is one worse than the other? And I’ll talk about why that’s important and again, it gives us a sense of the physical scenarios that are most injurious. So we wanna look at that, and then ultimately we wanna take all of this information that we learn about the biology of the injury in the laboratory and we wanna bring it back to the real world, we wanna bring it integrated into a model that can make predictions, right, that can make predictions about when an injury might occur and it can help us to design better materials that would prevent it in the first place, okay? So again, a lot of words here. I’ll keep it short. The goal is to develop these critical injury metrics that tell us when an injury occurs, okay.
So again, a little bit more vocabulary. This is a schematic, and I’ll kinda walk you through it. Some of this, the details here are not as important as you know, the bigger pictures, but here is a schematic of a sort of a cartoon person’s being subjected either to an impact to the front head or a blast over pressure. Again, this in the civilian world is usually very rare to find, but certainly a lot of veterans were exposed to this during the wars in Iraq and Afghanistan, and there was a lot of question what happened to the brain in these cases. When we think about the initial insult, so if I were to fall off the stage and hit my head on the floor, if you could slow everything down times and had high-speed cameras to do slow motion, what you would see is there would actually be a mechanical wave entering my brain. And if we think about this wave, we can think about it in two parts. We can think about one part. So this is two potentials, so you can think of about one part of mechanical wave energy that goes in, it tends to distort the tissue. It tends to really stretch the cells, deform it, twist it, turn it. There’s another part that is the pressure, and the pressure would just tend to compact everything, and what we’ve seen in the recent literature is that the pressure itself is not really that you know, injurious until it reflects intercranially and it switches sides.
So instead of being compressive, it becomes what we call tensile. And why is tensile bad? Well, if you try, if you take a liquid and lower the pressure, you can form bubbles. And the problem with bubbles and the brain is that typically these bubbles, when they grow, they displace the tissue around them. So again, they mechanically deform the tissue and that’s not good, but it can get even worse because when these bubbles expand and when they collapse, they tend to counter a lot of their kinetic energy into a single spot. And that spot could be a spot, it could be a jet, but this high energy content can literally tear through tissue. If you ever go to, if you go to the Web and type in cavitation erosion, the first picture that you see are hard steel propellers of ships, and you can see metal being torn out by this exact process, a bubble forming and collapsing. And so we wanna do a good job and we wanna track these things. The big difference for us as far as the brain is concerned, this pressure-driven cavitation injury is very localized, okay, to specific regions in the brain that experience these tensile pressures. This deformation-driven is much more widespread. So part of the work that we do here is we’re trying to understand the pathology of these measurements and see you know, can we reconstruct an individual’s? Whether you know, one was more exposed to these pressures or one was more deformation-driven.
It’s complicated and we don’t have a full answer yet, but we do have good approaches in the laboratory to isolate this, and I just wanted to kinda put this out there. Ultimately, as I stated in my objective, we want a plot that looks something like this, right? So there’s green area, there’s this red area, there’s this curve, this curve in between. This curve in between tells you when injury occurs and when injury doesn’t occur at the cell level. We wanna take the cell level to the tissue level and then the tissue level to the whole brain level. This curve right here, which right now the way it’s plotted, it’s plotted strained magnitude right, so how much you’re changing shape, how much you’re stretching, deforming something as a function of how fast you’re doing it. So if I show you this plot the way it’s written right now, it says that, and we find this in the laboratory and we find this actually in the community too. It’s very interesting observation. That is to say like when someone gets hit in the head, you don’t necessarily have to remove all of that kinetic energy. You don’t necessarily have to shield the brain from all that energy, as long as you can let some of the energy enter the brain slow enough, the cells can respond. And the simplest analogy I have to that is if you’re thinking about stepping up to bat at a Major League game, right? If you have a pitcher and the pitcher throws you a curveball at 50, 60 miles an hour and the ball is coming right at you, you may have enough reflexes to step out of the way.
But when the fastball comes at 95, at least if I stand at the plate, there’s no chance. [audience laughing] I’m just gonna get hit. So the loading rate comes into play and when the cells have time to respond to the change in that mechanical environment, to that sudden stretch, they can react, they can react internally, and we can actually like, they can actually take a good amount of strain, and stress strain gives you energy, so they can actually absorb a good amount of energy without being injured or being destroyed. But if that happens relatively quickly down here, and then, you can see that that barrier is lowered significantly, okay? So a lot of the work that we’re doing now with the community is trying to really hone in on what that shape of the curve is, where is a crossover occurring, and then you see, there’s two color, two color demarcations. So there’s this darker red region and there’s this lighter red region, and the difference between the two is one is purely the you know, the primary physical damage to the cells, right? That’s rupture, that’s physical stretching. And this other inner region, that’s the change in the biochemistry, okay? That’s that slow progress degeneration, and we want to be able to track both, we want to know what both are. And then from an engineering prospective, what we like to do is we like to develop techniques and protective equipment that takes you know, a scenario where an individual would be out here and shifts them to the left, into the no injury zone, okay? So that’s the basic premise, I gonna show you the data that we have today later on to build this curve, but there’s a lot more work needed to really get there. Okay, so what I’m gonna show you now is a little bit of, sort of a length scale evolution, a little bit of a historical evolution here. This video was from a collaborator of mine at Brown University, who at the time had a larger study with them that was sponsored by the National Institute of Health, with several colleges that set out on a very simple mission. It was to say, let us take accelerometer-based sensors, instrument our students with it in their helmets, and track some of the collisions that they suffered during practice and during the play.
In this video, what you see is there’s a Virginia Tech running back right here, and then there’s a stop, hard stop here is a head-to-head collision, and there’s an accelerometer, acceleration time profile that’s being recorded, and at the time, the simple– again this was in 2003, so this was some time ago. At the time, the thinking was if there was a correlate between these numbers here and a concussion, then we could start to make some predictions. The problem was it didn’t work. And part of it, the problem didn’t work is because this measurement was too simple. The brain is much more complex and again, as I said earlier, like if I were to fall off, there’s this wave that enters the brain. There’s the pressure part that moves rather quickly, and then there’s sort of the tissue rate that moves slowly, and you can already see these hot spots, right? And these hot spots depend on the anatomy of the brain. The hot spots depend on all the curvatures, and they depend on the different constituents within the brain and their mechanical properties. So there’s the geometry, the anatomy of the structure, and then there’s actually the mechanical properties, whether something is soft or stiff in the brain. And all those things matter in order for us to get a prediction on whether we have an injury or not. So just having a sensor out on the skull to say, “I see a linear acceleration of 20, 50, 60 G,” is not enough to tell us whether an injury has occurred, okay? So in large part, what the community has been doing since then, since 2003 and up to now is working on refinements, trying to really better capture the biology of the input of the brain and connect that with measurements that we can do on the field.
So again, if we go through this sort of cascade and we go down, again, there’s a network level that I said gives us you know, a cognitive ability, but then there’s these individual cells, and particularly, like here we focus on the neurons. The thing to keep in mind too is like, to really understand the injury response of a neuron, you have to understand the neuron’s environment. You have to understand how these cells connect and how they talk to their neighbors. And so for me, this was you know, it was funny, like I got my PhD in 2008 and then I became a postdoc, which is really when I got involved in this, and I thought I was done studying and learning and I just went right back being a student, okay? ‘Cause I ran into this problem and I said, “Look, like the way we think about the brain “is not necessarily how the cells think about the brain. ” Because the cells doesn’t know there’s all of us, it doesn’t know they’re individuals. The cells knows its neighbors. It knows signaling, it knows action potentials. It knows certain structures, and so for us to really be able to understand how a cell responds, we have to sort of go in there and understand the cell. Okay, the next few slides gonna orient you on how we think about and how we classify injury and neurodegeneration that’s due to a traumatic brain injury at the cellular level, and what again, what I’m doing here, I’m dropping you down in powers of 10 from the centimeter head scale all the way down to the sort of atomic, well, not atomic but cellular micro scale. So we’re going from centimeters all the way down to microns.
There’s a lot of pump date and color here. So this is a coronal cross section of the brain as a sort of inside focus around the corpus callosum, you can see these sort of black spots. Again, these are microbleeds, these are signs of axonal or cellular degeneration that happened post a traumatic insult to the brain. And again, you can see the sort of in a more, sort of larger scale tissue slides. People will highlight different broken structural components of the cell, depending on what disease they’re interested in, okay? And so earlier I showed you work from Ann McKee that’s looking at this protein called tau in the context of tauopathies. Here, people look at amyloid precursor protein, which is involved as sort of one of the hallmark proteins in Alzheimer’s disease. What’s interesting is like all these proteins initially belong to the structure of the neuron, and they’re in the right order. When the cell gets impacted and when the cell breaks apart, these proteins get released. They get cleaved, and they accumulate in these patterns that we understand to be hallmark features of neurodegenerative diseases. So part of it is the puzzle, trying to figure out how did we get there, and what does an impact have to do with all of this? Okay, so to do this, we go down in scale.
In the laboratory, we work with microscopes, so we tend to operate on a size scale that’s usually millimeter and all the way down to about what light can allow us to see, so that pushes us down to about submicrons, so about a hundred nanometers in scale, and when we look at injury at that scale or we typically look for these sort of round blebs that could form along an axon, then the naive axon just looks very beautifully curved and has none of these little blebs on it. Once you see these blebs, these are essentially like broken internal structures of the cell and they’re indicative of an injury. Once the cell gets to this point, there’s no, there’s no more return. This cell will die. And so this is the sort of hallmark feature that we look for in the laboratory that’s indicative of a traumatic brain injury. Okay, a little bit more about the cell because it gets more complicated, and you shouldn’t be surprised as it’s the brain and the brain is very complicated. This is a schematic again of a neuron. You have to imagine this cell lives with millions of neighbors and they connect, each cell has this long axon and it has an axon terminal, and that terminal will connect to these little spikes up here, these dendrites for other cells, and they form a large-scale network. There’s this different components, there’s these dendrites, there’s the cell nucleus that holds the DNA, and so you have electrical connectivity of signal propagation all the way through the axon into the terminals to the dendrites, and then it continues. Thanks to technology and really advanced imaging and thanks to the neuroscience community at large, we have a good understanding of actually the proteins that make up most of these structures.
And that’s kind of shown here schematically, so we can zoom in on one of these structures and it’s actually just not a solid structure, but it has many, many proteins that have a very complex hierarchy that come into play. The reason I’m telling you all of this without going more in detail on that is to say that when we apply a mechanical load of force on this, we have to be aware of this. We have to understand how these proteins get loaded how to talk to one another. It’s the same thing when you build a house and you all of a sudden see a hurricane sweep over it. Whether your house falls or not depends on how the house is built and depends on what materials you used to build the house. And the same is true for these cells. And right now, what we do in large part, we’re trying to understand if different types of deformation compression, which is you take something and compress it, shear, which is something that you would you know, take and you sort of shear it along, or tension and straight-up pulling, whether any of these tend to be more injurious to the naive structure of the cell or not. And again, the reason why this is all important is because there’s alignment in the brain, there is structure, and if we can understand this, maybe we can make a prediction that certain parts of the brain are more vulnerable or more susceptible to injury, or that certain parts for a given scenario are more susceptible than not. So there’s a lot of things that we have to track, okay. What I’m gonna do is I’m gonna take you in the laboratory.
This is gonna be so, to give you just an overview, what I’m showing you here is these are sort of the gel-like constructs that we make, we use a mechanical scaffold. That’s extracted collagen. We build these neural networks here in green. You can see these networks that form in three dimension. We have three-dimensional imaging modalities in the lab that we can image them and we can track individual cells. We can look biologically of all the different cell types that are in these networks from neurons to astrocytes to stem cells, progenitors, again, a lot of details that we go through. And then what we do is here. So we mimic an impact that we can devise from the literature. Again, this is typically for blunt, head-to-head collisions on the order of milliseconds, and if I play this again, which you can– So over here is a real impact. So in green are the neurons.
This is a small window, imaging window of a much larger sample. You can see there’s a compression, compressive impact top-down. In blue, I actually track a particles that we can track in three dimensions to map out the entire loading scenario around the cell. We can take this information in high resolution, we can reconstruct it, we can look and see how the cell is connected to neighbors, how it’s connected to the tissue, and essentially what we’re going for is we’re trying to understand how the mechanical forces are being transferred from the environment onto the cell, okay? And so there’s, and then what we do is there’s a lot of imaging involved that we have to go through. It’s interesting, like I showed this to you in one slide, this took about six years to build, it took a lot of people and it surprisingly took a lot of detailed information from different communities. So like I said, our background is in mechanics, we did backgrounds in neuroscience, but a lot of this imaging actually came from computer science, computer vision, like there’s a lot of things that we had to do, to implement to get to this point, but ultimately what you end up with is, and I’m gonna skip some of these details, is a fully reconstructed depiction of every single cell in this like thousands of millions of network-sized artificial gel that we grow where we can now do an analysis and we can understand how these cells are being mechanically loaded, okay? And remember, we do this all on the microscope live, so we can do the scanning. This is a quantification of the injury metrics that we do. Again, I’m not gonna go through all the details. Just suffice to say that if you can use all of what you’ve learned in mechanical engineering to predict failure in buildings and cars and planes and you can apply it to this problem to understand when these cells show damage and injury, and then we can make correlations and predictions. Again, I told you this is the sign of a traumatic brain injury at the cellular level, and we can ask questions now.
When you see this, what was the critical strain? What was the critical stretch at that point, right? So we can make these correlations. The interesting result that we found is that shear seem to be very critical for these cells. And it seems to be a critical threshold to generate this kind of varicosities, blebs that we find. It was a bottom number of 14%, again, there’s a lot of questions that we’re addressing and refining, but what was interesting was not all cells showed this sort of feature. So we said, like “Okay, this cannot be the full story. ” And so after a lot more other experiments, what we found is like it turns out that for these impact-like rates that we generate in the laboratory, there seems to be a critical sheer strain magnitude, about 10%. Above, once you hit that mark, then cells start to display this classical injury of traumatic brain injury that you would see that was documented postmortem in humans as well as in animals. The interesting thing is like, this level of strain was actually like, was the average strain across the entire set of cells. So what this says in a nutshell is that cells are very good at regulating their own chemistry, but they’re also very good at regulating their own physics. And it is like you know, when you exceed this sort of threshold, when a cell says you know, “This is too much force. I can’t take this anymore. ”
That’s when it needs to shut down, okay? We looked at the time window here. There’s a time of cell death as a function of the mechanical loading. We did some analysis here. The big part is here that like we started to see that there is a critical onset of strain when the cells become injured. We track the pathology over time points to 0 to 10 hours. We can see, we can follow the degeneration of individual cells within these networks live. We can look, we actually discovered a new pathology of injury, which is at much slower loading rates. So these are like really fast hits, these are small hits, and I will say we can reconstruct head-to-head collisions from the playing field very accurately. It’s still a jump to understand once the wave enters the brain, what is the exact loading rate that the cells see, okay? So that’s something that we’re still working on, but for us it was we wanted to pick the whole of you know, pathology atlas of what the injury may look like, so we found some interesting things there.
We can look at high-rate loading. Again, this is now much, much faster, and this comes back to the piece I mentioned about cavitation and little bubbles in the brain. This is a bubble right here that expands and collapses, and the color coding is the level of strain that this bubble is exerting the surrounding material. Suffice to say this requires a whole ‘nother set of experiments and apparatus. This event is typically over in about a hundred microseconds. So a hundred billionth of a second. It happens extremely fast and it’s highly localized. So my students right now are looking at what the pathology looks like to this. Here is sort of one final example and then I’m just gonna wrap up. So here, what you see in green, star-like, this is a neural network projected all the way down into a two-dimensional slice.
And right here is where a bubble formed. It expanded and collapsed, and it was very violent because all the cells are gone in the center. And what was interesting is we can then go in and use high resolution imaging, and we can then look at individual breakdown of these cells. And with you know, working with other collaborators that are very good at making models and theories, we can actually map out what the strain is as a function of this field. We can map out what the strain is that each individual cell sees. In this case because you have a bubble here, the strains for the bubble are very high. The strains far away from the bubble are very low. And you can see in the far field cells are healthy, and very close cells are completely destroyed, and this right here by the way, this is all primary injury, okay? So this is all physical, direct injury because the bubble was pulling the material as it was off splitting around. And so what we’re looking now is like are is there any other activations that happens you know, 4, 6, 8, 12, 24 hours afterwards? In other models, tissue-level models, you can look at inflammatory response, so there’s other things we can look at. And just to give you a sense, again, We’re looking for pathologies right, because when you do a postmortem analysis, one thing that we already saw with the pictures I showed you earlier, the fact that we discovered a new pathology that was not centered around finding these little ring-like spots was to say that if you look, if you do a postmortem analysis on a human being and you have to look for something, for some features, right? If you say “I wanna look for these rounded features,” you may miss actually a significant amount of damage that that individual sustained.
And so part of this what we’re trying to do is develop more complete pathology maps that we can map that out and then map that back, okay. And again, this is sort of like you can travel around. Beautiful thing is that at this point in time, we have a lot of imaging power at our disposal to do this. These are not easy experiments. And if my students were here, they’d probably laugh at me because I’m making this sound so simple when their everyday life is actually very difficult in the laboratory, so. [laughing] But I will acknowledge them later because they really carry all the heavy load. We can make predictions of how the strain, this blue curve, how this strain drops off again to identify critical injury thresholds. What was interesting here is the threshold here was also around 10 percent, so it was very similar. So here I’m bringing back this curve as my sort of final slide. I can tell you kind of where we are with our injury prediction based on the data we go on that we take from the laboratory.
The green, this ciruclar point is our data points right, so we can we can see here at very slow rating, loading the cells to remain healthy and non-injured. Once you move into this realm of falls, motor vehicle accidents, collisions on the football field. You enter the injury realm, your strain level drops, and then there is data points from the literature that we could find, whatever we could find that people reported well. So this was another side note of what my students found too, that often times we all, and by we I mean we as scientists can do a better job in reporting what we actually do when we write papers. So often times it was hard to do an apples to apples comparisons, but there was some pivotal studies that we found and we could put on this curve. So you can see that’s the curve. That’s the data points. You can say like, “Well, you don’t have nearly enough “data points to make this curve,” and you are absolutely correct. So we have a lot more work ahead of us to really figure out what the shape is, but the hope is that we’ll get there. I think we’ll get there.
I think we do have a good approach. I’m gonna call us very much a work in progress. We’ll see what this brings, but the good thing is we’re very– This lends itself into a form that it can be integrated relatively quickly into a computer model and make some predictions. I wanted to talk about Rika’s work a little bit. I’m not really gonna have time to do this, but maybe I’ll share with you one slide. So she’s been looking at doing simulations of rotations on the head. We know rotations are very injurious. She’s been making connections to use some of this information. She’s been looking at rotations around different planes of the head, coronal, axial, seeing what’s most injurious, and I’d kind of just like give you a sense here These plots where you sees it off the top levels here. This is tissue-level strain, which we know can be injurious, so that’s what we wanna look for as a function of angular acceleration and angular velocity.
So there is this question of like you know, is it more injurious if something happens really, really, accelerates really, really quickly, or if the velocity is larger, so we’re still trying to understand that. One thing that I can tell you is that rotations to the head that happen quickly and that are sustained are really bad, okay? So part of it is, and again, this comes back to how we think about it, any situation where the head rotates, and we’ve seen this now evidence from playing field from various sports, so take football, take boxing. And then we did, we looked at a study with some collaborators at Stanford, who followed a cohort of mixed martial arts fighters, and they looked at them as well, and again, it comes all back to rotation. Rotation, rotation, rotation. There’s critical rates at which rotations really become injurious, there’s rates at which it’s not. I don’t have final answers for you yet, but this information is coming. So if you’re interested, ping us, let us know, connect with us. We’re hoping to get some of these results out into the literature within the next six months to a year. So I’ll leave it there. So you can kinda see color coding here is that you can see the red is, so that is predicted injury based on the tissue-derived injury model that I just presented to you, okay? So we have to do a lot of fact-checking refinements and this is coming, but the foundation is there, okay? The foundation is there to start to make this predictions, and a lot of this what Rika is doing now with her work is trying to just like get an intuition of like what kind of motion is most injurious to the brain.
The great thing is with simulations and computers, you can do that, you can explore that space. So I’ll leave it at that. I’m gonna leave the summaries here. I talked about them. This is our program, please visit us. We do have a news outlet page. We’re trying to get information pushed out. We always welcome any input, any feedback from anyone in the community, and I really, really– This is the most important slide here for me is to acknowledge all the great students, everybody that’s been involved, all my former students, my current students, and then my program manager at the Office of Naval Research, who is a strong proponent for basic science and basic research to really, to move this ahead. And with that, I’d like to thank you.
– Woman: Hi, thank you, that was really interesting. My question is not related to impact injury, but to the injury caused by stroke. And I was wondering if that lighter red curve, if that’s an area where the biochemistry of cell damage and death could be applied to a stroke model? Can you learn something from that to help people with stroke?
– That’s a really good question. It’s possible. I will say that the premise of that curve is based on a mechanical origin, right? So it’s a deformation-based origin. So in the short model, it just depends on how the stroke is being induced at the level of the cells. So you know, whether that’s hypoxic or, it just depends on the exact condition of that. So maybe. Which is– [all laughing]
– Man: Is it known if the deployment of an airbag in an accident has enough force, the time and force is large enough to mimic a blast injury?
– Christian: It, as far
– Man: So that you might be, your life might be saved, but you might have an unknown brain injury?
– Christian: Right, to my knowledge, it’s not known. And I think it’s a very pertinent question, and you know, you sort of touched on a very interesting point right, like in the beginning, a lot of safeguards that we have now were put in place to you know, stop people from dying. But then the second thing is like now that people live, do they live with healthy brains or do we actually induce damage by having too crude of a protection mechanism? With what I think what you have is a good question.
So we’re hoping that like once we have a better sense of what kind of motion induces that, we can come and look at that.
– Man: Excellent presentation, and my question is related to soccer.
– Christian: Okay.
– They do the heading, which is becoming so popular. Has anybody tried to study it and what– well, people should be studying it because they could start playing when they’re very young and they start heading when they’re about 10 or 11 years old, and I think yeah, what do you know about it? So thank you.
– So there are several groups that have looked at this. The studies, it’s had to draw conclusions from some of these studies, in part because we still don’t know what really causes the injury. So once we know what causes the injury and what level of acceleration and strain causes the injury, we’ll be able to make better interpretation of some of those studies. I will say that like within the world of soccer, the fact that the ball itself is fairly deformable, it helps the cause. So usually like as far as the studies that I’ve read, where we find the highest concussion risk in soccer is actually player to player collision and player to goal post collisions.
Okay, so in those cases you have rigid skull against rigid skull or rigid skull against you know, an aluminum post. The heading question is a good question, and I think, I am hoping that at some, I’m hoping that within the next few years we’ll be able to really get to that question. In terms of like, how much attention is the community paying to it, it sort of comes and goes. There’s a couple of studies that pop up and then it sort of goes away. It’s marginally small compared to the studies that focus on heavy-duty contact sports like football or like boxing.
– Woman: My question is whether your model for the neuron in the laboratory is assumed to be applicable equally to adults and to children and to men and to women.
– That is a great question. And the truthful answer is we don’t know yet, right? We have to keep in mind that the laboratory model is still a very big simplification of the real brain. And I think that’s a level of refinement, that’s a level of community engagement that we will have to see. I think part of the effort is that we come and work on these problems together.
One of the things that we have sort of taken here is to say that every cell is precious, right? And so maybe initially, the thought is that we over-engineer the protective solutions and then we can refine. In children, certainly the regenerative potential is much higher. So there’s that aspect to it too. People look at the state of myelination in the brain, which also comes into play. I have not touched upon this in this talk. The point being is this is something that we will look at, and we will look at it more closely. So it’s on our to-do list, on our very long to-do list. [all laughing]
– Man: We’re out of time for today, but I wanna thank everybody for coming out on a very cold day.
– Yes.
– And thanks to Christian for a wonderful presentation.
– Thank you. [audience applauding]
Search University Place Episodes
Related Stories from PBS Wisconsin's Blog

Donate to sign up. Activate and sign in to Passport. It's that easy to help PBS Wisconsin serve your community through media that educates, inspires, and entertains.
Make your membership gift today
Only for new users: Activate Passport using your code or email address
Already a member?
Look up my account
Need some help? Go to FAQ or visit PBS Passport Help
Need help accessing PBS Wisconsin anywhere?
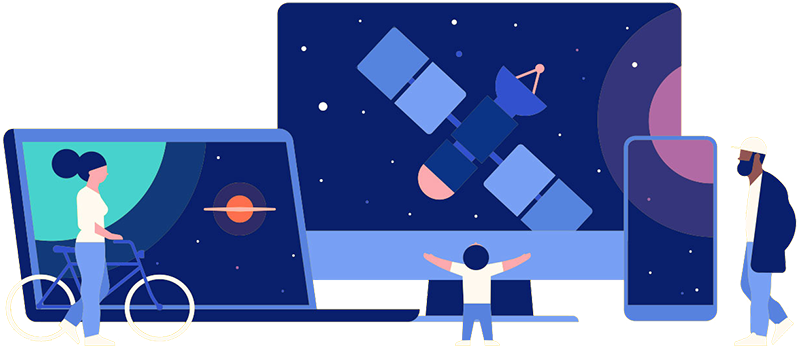
Online Access | Platform & Device Access | Cable or Satellite Access | Over-The-Air Access
Visit Access Guide
Need help accessing PBS Wisconsin anywhere?
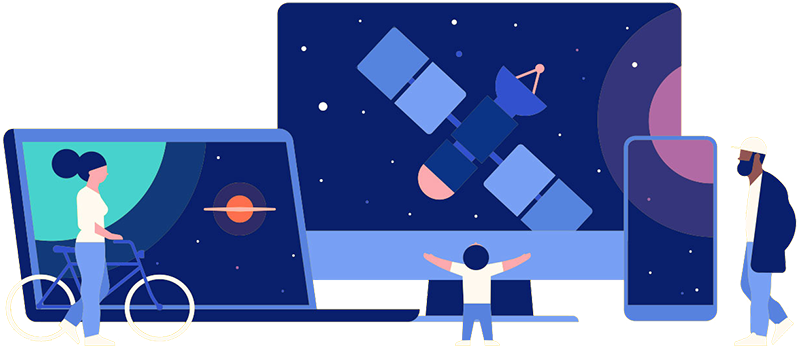
Visit Our
Live TV Access Guide
Online AccessPlatform & Device Access
Cable or Satellite Access
Over-The-Air Access
Visit Access Guide
Follow Us