– Welcome everyone to Wednesday Nite @ the Lab. I’m Tom Zinnen, I work here at the UW-Madison Biotechnology Center. I also work for UW-Extension, Cooperative Extension, and on behalf of those folks and our other co-organizers, Wisconsin Public Television, the Wisconsin Alumni Association, and the UW-Madison Science Alliance, thanks again for coming to Wednesday Nite @ the Lab. We do this every Wednesday night, 50 times a year. Tonight, it’s my pleasure to welcome back to Wednesday Nite @ the Lab, Jean-Michel An. He’s a professor in Bacteriology and Agronomy. He was born in Montpellier, in France, and went to lycee at Montpellier. He got his undergraduate degrees in Paris and Lyon, and then he got his PhD at Toulouse in plant microbiology. I have to stop now and tell my Toulouse joke. Recently, Jean-Michel said, people don’t go visit only Paris. Go to other cities. And I said, “Great, what have you got, Toulouse?” (audience laughing) That was better than I thought. (audience laughing)
Then, he went to UC Davis to postdoc in the Plant Pathology Department. And in 2004, he came here in the Agronomy Department. He’s also in the Bacteriology Department, and at the molecular, excuse me, at the Microbial Sciences Building. Tonight, he gets to talk to us about this great project, aerial roots of Mexican corn can host nitrogen-fixing bacteria. Please join me in welcoming Jean-Michel An back to Wednesday Nite @ the Lab. (audience applauding)
– Thank you. Thank you for this invitation to speak again at Wednesday Nite @ the Lab. It’s a pleasure for me to be here, and to have the chance to talk about this project we have been working on for almost 10 years. And finally, we are been able to talk about that more publicly over the last months. So I’m going to talk about a project where we basically discovered and studied maize accessions, or landraces from Central Mexico that can associate really well with bacteria that can fix nitrogen, and I’m going to explain you, too, at the beginning why that’s a big deal for us, and I think for agriculture. Before I go into the science, I would like to acknowledge that that project has been initiated and funded for many years by the Mars company, and it was a collaboration between the Mars people from the Mars company, colleagues, several colleagues at the University of California Davis, and in my lab here, at the University of Wisconsin-Madison.
So nitrogen, why nitrogen matters. Well, you probably know already that nitrogen is an essential nutrient for plants. It’s the important building block for proteins, nucleic acids, DNA, RNA, and I’m sorry for the color, which is a bit weird, but the plants can acquire nitrogen from the soil, primarily in the form of nitrate and ammonium. But there is a huge pool of nitrogen in the air. And the process called nitrogen fixation is the process of converting dinitrogen from the air into ammonium, a form that the plant can assimilate. You have different types of nitrogen fixation processes. One is what we call atmospheric nitrogen fixation. Every time you have a storm and lightning, it converts actually dinitrogen into ammonium. And actually, a large amount of nitrogen fixed that way. I have numbers later. There’s a process that I’m going to discuss of what we call industrial nitrogen fixation, also called the Haber-Bosch process, after the name of the two German scientist who discovered that system at the beginning of the 20th century. And then, you have the system of biological nitrogen fixation that I’m going to discuss a lot today, which is performed by bacteria. Only bacteria can do that, but fortunately for us, many of these bacteria can associate with plants, and transfer that nitrogen that they get from the air to the plant. And you may have heard in particular, about legumes, like soybean, able to do that.
So I talked about different processes of nitrogen fixation, and here I have numbers. As you can see, atmospheric nitrogen fixation fixes globally around the world, every year, about 10 million tons of nitrogen. Biological nitrogen fixation is the one I’m going to discuss mostly today. And as you can see, it’s 90 to 140 million tons of nitrogen fixed per year on terrestrial systems. You also have biological nitrogen fixation happening in the oceans, and it’s actually in term of numbers, even more important, but I’m not going to discuss that today. I’m going to talk about what is relevant for agriculture, and so about terrestrial systems.
Biological nitrogen fixation is allowed by, is first, occurs only in bacteria, and in bacteria that have a specific enzyme, we call the nitrogenase. That enzyme is composed of two different proteins called the iron protein here, and the MoFe protein. I’m not going to go into the details of how the nitrogenase works, but I just want to mention that UW-Madison for decades, has been a hot center of research on the biochemistry of that enzyme. So we know a lot about the biochemistry and how that enzyme works. What I want you to remember today is basically what it’s doing. It’s converting dinitrogen from the air into ammonium. And so it’s breaking the triple bonds that you have in dinitrogen. And to do that, it requires a lot of energy. So that enzymes requires about 16 molecules of ATP to break one molecule of dinitrogen, so it’s a lot of energy. So I’m going to talk about problems linked to nitrogen fixation, or challenges, and one challenge is for the bacteria that want to perform nitrogen fixation, they need to have access to a lot of energy, and typically, they often get that in the form of a source of carbon. So the nitrogenase needs a lot of energy to function. The second problem that you will need to solve in any efficient nitrogen-fixing bacteria, is oxygen, because oxygen inhibits that enzyme, which is a significant problem. Many bacteria need oxygen to do respiration, like you, and respiration is necessary to provide ATP, and at the same time, oxygen inhibits their nitrogenase. So it’s, bacteria system where we have efficient nitrogen fixation, usually operating what we call microaerobic environment. It means, just a concentration of oxygen which is lower than what you have in the air. Typically, things between 1% to 5% oxygen tension, versus the 20% that you have in the air. So these are two problems that I’m going to talk about, again, later, because again, if you want to have any efficient biological nitrogen fixation, you need to find a solution to these two problems.
You probably have heard about, as I said, about nitrogen fixation in the context of soybeans, and soybean fixes a lot of nitrogen with bacteria called rhizobia. And in particular, in that system, you have the formation of these small structures that maybe you have seen on soybean roots, that we call nodules. These are root nodules, these are new organs that form on the soybean roots in response to these bacteria. And these are like little houses that house the bacteria, and the bacteria are not on the inside of these nodules, they are even inside of the plant cells. So the bacteria are really deep inside the plant tissue, and the fact that you have such a close association, clearly facilitates the exchange of nutrients between the two. So clearly, root nodules in legumes like soybeans are extremely efficient. So I apologize for that slide which is a bit crowded and has a lot of information, but at the same time, I think it should be interesting information. So here, I show you basically the three main type of associations between plants and nitrogen-fixing bacteria. The one that most people are familiar with is that one here, root nodule forming symbiosis, like what you can see on soybeans, on alfalfa, all these legumes. There, the bacteria that can do that are called Frankia, or rhizobia. And the root nodule solves the three, for the two problem that I mentioned, which is energy source within the nodule, the bacteria will have access to a lot of carbon from the plant, and so that clearly facilitates the nutrition of the bacteria.
Inside of the nodule, you also have these, a good oxygen protection, not only because the bacteria are deep inside of the plant tissue, but also maybe you have heard about that protein called leghemoglobin. And there’s that funny experiment that you can do, if you go to a field, and you dig up a soybean, you take a nodule, and if you pop it in the area, in your hands, you’re going to have a little drop of something that looks like blood, and we call that leghemoglobin. It’s a hemoglobin of legumes. And that leghemoglobin is actually a system that helps protecting the bacteria from oxygen, providing that microaerobic environment inside of the nodule, protecting, basically, the nitrogenase against oxygen. Inside of these nodules, the rhizobia, and the exchange of, the transfer of the nitrogen fixed by the bacteria to the plant, again, is going to be very efficient because of the close proximity between the two partners. And in the end, not surprisingly, the rates of fixation in that system are very high. You see 50 to 400 kilograms of nitrogen fixed per year. So most people are familiar with that one, but it’s not the only type of association between nitrogen-fixing bacteria and plants. You have more loose association between plants and bacteria that fix nitrogen called associative fixation, and the system I’m going to show you today with the corn falls in to that category. That’s why I’m introducing that kind of association. In that case here, you don’t have a new organ, which is formed. You simply have the bacteria colonizing the root surface.
In other cases, you have the bacteria colonizing part of the leaves, or small crypts inside of the leaves. In all cases, what you have are the bacteria in close proximity to a plant. And again, that close proximity’s going to facilitate the exchange of nutrients, it’s going to facilitate also, also it’s going to provide the oxygen protection. So these associations are usually less efficient than what you have with legumes and a new organ, the nodule, but still, they’re able to fix a good amount of nitrogen. And briefly, I want to mention the last one that we call free living fixation. You have nitrogen-fixing bacteria in any soil, and in many soils, they’re going to fix nitrogen for themselves, but then they’re going to die, and that fixation that happens in the soil without really any specific interaction with the plant provides some nitrogen to our crops. The bacteria that are able to get their energy from the sun and do photosynthesis, we call that the autotrophs, are able to have more energy, and for there are nitrogenase that needs a lot of energy, and therefore, will be able to fix more nitrogen. So also, it’s good to keep in mind that even if you don’t have a close association between these bacteria and the plant, the plant, in the end, the crops are, in the end, going to benefit a little bit from that. So that’s what my introduction for the biological nitrogen fixation.
And again, today, the one that I’m going to show you falls into the category of associative nitrogen fixation. There is no new organ in what I’m going to show you today. I wanted to take some time, also, to talk about what we call industrial nitrogen fixation, and I call that both a blessing and a curse. It’s a system that has been invented at the beginning of the 20th century, and over the years, our dependence on synthetic fertilizer increased. So what you have here, what that graph represents is the world population, and in red, it represents the fraction of the world population which is fed using synthetic fertilizers. So synthetic fertilizers that Haber-Bosch process was invented at the beginning of the 20th century, but it’s use in agriculture will increase during the ’60s and ’70s, during what we call the Green Revolution, where we started to use a lot of these chemical fertilizers. And currently, today, you see that basically, we are feeding half of the world using synthetic fertilizers. So we may criticize these fertilizers, but they are really feeding half of the world, so we need them. And so any approach that we have now to use more biological nitrogen fixation, the goal really is not to eliminate completely the synthetic fertilizers. The goal is to reduce their use, because there are some problems associated with the use of synthetic fertilizers.
One of them is where they’re coming from. So in order to make natural gas, to make synthetic fertilizers, to fix nitrogen chemically, we use natural gas, and natural gas is a fossil fuel. So currently what we are doing is that we need about one ton of natural gas to produce one ton of fertilizer. And so we use a lot of natural gas to make fertilizers. In fact, globally, around the world, we use about 2% of our energy, globally, to produce nitrogen fertilizers in the world. It’s a lot of natural gas that we’re using. So we are using natural gas to, through the Haber-Bosch process, produce fertilizers, and we are using that fertilizer to grow our crops for food, feed, and ironically, even biofuel production. So first, I think it’s a bit scary for the sustainability of our economy in our world that we are feeding half of the world on fossil fuel. It’s not a renewable energy. We know that sooner or later, it’s going to, we won’t have enough of it. Current, we have plenty of it, but that won’t last for long. So we are feeding half of the world, when you think about it, on fossil fuel, which can be scary for the sustainability of a system. Even more scary, or I would say, ironic, is that we are using fertilizer to grow crops to make biofuel. So when you think about it, we use fossil fuel to grow crops to make biofuel. So obviously, the system is not efficient. And so both for the sustainability of our agriculture, and also, in particular, if we want to make biofuel production efficient, we need to rely less, far less, on fossil fuels.
An economic consequence of the fact that we are making fertilizers out of natural gas, is that every time you have a spike in the cost of natural gas, so the price of natural gas over the years is here in blue, and every time we have a spike like here, around 2001, in the price of natural gas, it goes with an increase of the cost of fertilizers. And basically, you can see that the cost of natural gas mirrors very closely– Sorry, the cost of fertilizers mirrors very closely the cost of natural gas, which makes sense. These days, as you probably know, we have plenty of natural gas, in particular, over the last decade, through the process of fracking, and because of fracking, natural gas is really cheap, and fertilizers are really cheap. So these days, the price aspect is not a problem for developed countries like here. The price of fertilizers is still a problem for developing countries, and for poor farmers in developing countries having access to fertilizers is limiting. And so, when you think about also developing new strategies using biological nitrogen fixation instead of chemical fixation, of course we need to think about farmers here, but we can also think about poor farmers in developing countries that simply cannot afford fertilizers. So relying more on biological fixation will be clearly useful for developing countries in particular.
When you think about problems here in developed countries, the problems are not so much, not so much, again, at the level of economy, it’s more at the level of ecology. And here what that pie chart is showing, is basically the different sources of greenhouse gasses, and agriculture, and the consequences of agriculture, are responsible for about 12.5% of the production of greenhouse gasses. And a very large fraction of these greenhouse gasses produced by agriculture is due to nitrous oxide. And nitrous oxide is a direct product of degradation of nitrogen fertilizers. So a large fraction of the greenhouse grasses that we produced with our agriculture is due to our intensive use of chemical fertilizers. You may have heard about another consequence which is nitrogen runoff. When we put an excess of nitrogen in the field, that nitrogen leaches into ground water, into streams and river, and in the end, for us here in the Midwest, ends up in the Mississippi River, and in the Gulf of Mexico. And all that nitrogen in the Gulf of Mexico leads to algal bloom, these algae grow. They consume oxygen when they decompose, and that creates a zone called anoxic zone, where you don’t have any oxygen. That basically is called a dead zone. And there is really good evidence that the dead zone in the Gulf of Mexico is a direct consequence of our intensive use of fertilizers here in the Midwest.
So clearly, for us, the main consequence we have of our reliance on synthetic fertilizers is an ecology problem. So in order to reduce these negative consequences of our use of fertilizers, there is the idea of using more the biological nitrogen fixation. And in particular, when we think about that, we want to improve the use of biological nitrogen fixation for cereals. So why cereals? Well, I told you first, soybeans can already, are really good at doing nitrogen fixation already. And I want you to, here, focus on that last line, that physically shows the nitrogen share of the operating costs to put use corn, cotton, soybeans, and wheat. That number here, honestly for soybeans, is very high, and even this morning, I checked with Shawn Conley, who is my soybean Agronomy colleague, and he was saying that that number is very high. It must be lower. But still, if you look at corn, or even more, wheat, about 18% of the cost of growing corn is due to the use of nitrogen fertilizers. Can we reduce that share, can we have corn that would, instead of requiring so much nitrogen synthetic fertilizers, would be able to associate with bacteria and get that nitrogen from bacteria. The problem is even worse when it comes to wheat, where you see 30% of the cost of growing wheat comes from using fertilizers. So can we rely more on nitrogen-fixing bacteria to replace at least some of that nitrogen which is currently provided by synthetic fertilizers.
So that seems a great idea, right? It’s not a novel idea at all. And here, what I’m showing you, it’s actually a book from 1917, from the University of Illinois, where basically they are discussing exactly that idea. I even have an article from 1900 where they are talking about the idea of having nitrogen-fixing cereals. So that idea of using more biological nitrogen fixation for cereals has been there for a long time, and has been a dream in my field of research for decades, and hundreds of years, more than hundred years. So what are the approaches? I summarized here in that slide, the main approaches that my community as a whole is following. It’s not only what I’m doing, I’m talking about what we, as scientists, in working on nitrogen fixation are thinking about. The first one is the best one ideally, is to have plants that would fix their own nitrogen without requiring any bacteria. The idea of having that nitrogenase enzyme instead of having it in bacteria, why don’t we make nitrogenic plants that has its own nitrogenase? That sounds a great idea. It’s on the technical basis, even if we know a lot about the nitrogenase, it’s very difficult. But I have colleagues like Luis Rubio in Spain, or here, Chris Voigt at the MIT in the U.S., who are really trying to reach that goal. From a technical standpoint, genetic standpoint, it’s extremely difficult, but if that works, that would be the perfect solution. But I would put that in the long, long term. We are clearly not there yet. The second idea is why don’t we make nodules on corn, on cereals? Why don’t we recreate the rhizobium-legume association into corn? That seems, actually, shorter term, because we know, we start now to know a lot, about how legumes associate with rhizobia, and so I have a project, myself, and with colleagues, at the University of Florida, but also have colleagues in the U.K., and in Europe, who are working really hard, we are all working hard, towards that goal.
Can we create nodules on corn, and create associations in cereals with rhizobia, just like what happens currently in soybeans. Again, it’s long-term, but I think it’s not as long-term as the first one. We are making really good progress on that. The third approach, it’s the one that I’m going to discuss today. Which is, I think, much shorter term, and could provide solution in the short-term time range. To have associate fixation, I was telling you that you need to have bacteria basically in close proximity with a plant. And there are different approaches for that. The project I’m going to talk about today, the idea is to have a really good host, a plant that can host this bacteria really well. I have other colleagues in, by the way, they’re also the private and public sector, who are trying to develop better bacteria towards that. Can we have bacteria that would grow, associate really well with our crops and would fix nitrogen. And there are both private companies, and academics or are working, also, on the bacterial side. In any case, it’s not a question of plant or bacteria, we need both fields to collaborate and work together. But the approach I’m going to talk about today is more, as you’re going to see, the trick, if you want, that we discovered, is more on the plant side.
So the project I’m going to discuss was initiated by this man, Howard Shapiro, who is working for the Mars company. And Howard has been working, exploring natural diversity for a long time. I’m sorry, this slide is really dark, at least from here. It’s a picture from a TED Talk that he gave a few years ago, and I would encourage you to go and listen to his TED Talk. I took from that TED Talk, basically, the main hypothesis that he put forward during his talk, which is that maybe some indigenous landraces of corn that are grown in isolated regions of Mexico may have co-evolved with microbiomes, or microbes, that contribute to plant performance in response to abiotic stresses, such as nutrient deficiency. And even if he did not say it like that, he was thinking nitrogen deficiency. So that’s the idea, is why don’t we look for the center of diversity of corn, and maybe there is something out there that works already, that we did not see before. Let’s look for plant natural diversity. Maybe plants figured out a way to associate with these bacteria. That idea was interestingly also put forward by a colleague of mine, Eric Triplett, who in 1996, published a review, and at the time, interestingly, he was actually here. He was at UW-Madison, in my Department of Agronomy, and in that review, Eric was saying, well first, he was stating what I just told you before, that the development of nitrogen fixation in maize can be considered the holy grail of nitrogen fixation research, and as nitrogen fertilization is one of the highest costs for corn production, the development of a symbiosis between diazotrophic bacteria, it means nitrogen-fixing bacteria and corn would be of enormous economic value. That’s basically what my introduction was about.
But he also was saying in that review, it may be necessary to isolate maize, diazotrophic endophytes from many locations, including the areas of maize origin in Mexico. So in that review, Eric was thinking more microbe side. He was thinking, we should go back to the center of diversity of maize, and find bacteria. The idea of Howard Shapiro was more plant-oriented, maybe there is a corn out there that figured out a way to associate with these bacteria. But basically, the point of both of them, was we need to look at the center of origin of corn, and that’s where you have the maximum diversity of plants, and possibly, microbes. So the story I’m going to tell you today, is a story about corn, what we call landrace, which is, you can say, a sort of primitive corn, which is grown by native people over there, by indigenous people. And that, as you’re going to see, can associate really well with nitrogen-fixing bacteria. So the maize is grown. So first, the corn was found in the state of Oaxaca, in the mountains of the Sierra Mixe. And it’s these mountains, as you can see, are 4500 to 6,000 feet above sea level. And the corn is grown here– I don’t know if you can see it– on the slopes of the mountain.
These fields are extremely leached, meaning that there is, the nitrogen levels are very low. And Howard, when he was scouting for unusual corn, was surprised to see corn growing so well on the slopes with so little nitrogen. On top of that, the native people, indigenous people, in their, we call that the Indian way of growing corn, they are not using nitrogen fertilizers. So the corn grows without fertilizers, on very low nitrogen soils, and only in these mountains. And that’s really what caught Howard’s attention. So the corn I’m going to discuss today, it’s an important part, as you can imagine, of the culture, but also, a food source for these communities, and that’s the corn they eat every day in tortillas, and that’s the major part of their subsistence. I mean, it’s major support for this existence. So it’s clearly a corn which is cultivated and grown by these communities.
Something else you need to know about the environment, is that it’s a very humid environment, and that matters for the story I’m going to tell you. Humidity’s always above 80%. It rains about twice a week, so it rains frequently. They have over three meters of precipitation per year, which is a lot, and the temperature is fairly stable between 25 to 30 degrees. So very humid environment. The main thing that you notice when you see the corn for the first time, is the size. If you are seeing corn in the field here in the Midwest, what we call the tallest corn, is typically seven to eight feet high. Well, that one, you can double it. That one is 15 to 18 feet high. It’s huge. The people that you can see here in the field, they are not kids, they’re adults in the field. (audience laughing)
So it’s a huge corn, and it’s planted in April, harvested in November, so it’s growing over a really long growing season that we could not afford. When we grow it here in Wisconsin, we basically never reach maturity. And again, it’s grown over there without any nitrogen fertilizer. So that was really surprising. And the first technique that we started using over there to understand how the corn could grow with so little nitrogen inputs is a technique called 15N natural abundance. And there is a variation of that technique called 15N dilution, I’m not going to talk about that today. But the idea here is that 15N is a heavy isotope of nitrogen. So in nitrogen, you have the normal isotope, nitrogen-14, but you always have a little bit of a heavier isotope called nitrogen-15. And that’s what we are using here. Because it turns out that in the air, in the atmosphere, you have very little of that heavy isotope. You see, it’s basically 0.33 ppts. Whereas, in the soil, in almost every soil, you have much more of that heavy nitrogen. And so if you have a plant, like, let’s say here, in the example, it’s a legume that can fix nitrogen. It means that plant is getting more nitrogen from the air than the soil. But the air has very little 15N, the soil has a lot of it. And so plants that can fix nitrogen will have less 15N than the plant that gets all of its nitrogen from the soil, if that makes sense. In the soil, so a plant like here, that cannot fix nitrogen, is going to get all its nitrogen from the soil, and so the 15N content of that plant would be identical to the soil. A plant like here, a legume, that gets part of its nitrogen from the air, will have less 15N, because you have almost no 15N in the air. So that’s the technique that we are using in the field, because it’s very high through-put. We can go to the field, do leaf punctures, and see how much 15N is in each plant. So that’s a technique that we could do right away in Oaxaca, in the Sierra Mixe corn, and try to estimate if there is succession. And that’s where the first striking results came. (laughs)
We did three years of experiment in the field, and what we saw initially was shocking to us. We saw that what we evaluate here, that axis here, represent what we call NDFA, for percent of nitrogen derived from the air. It’s telling us, it’s basically, by looking at that abundance of 15N, we can estimate how much nitrogen comes from the air. If you do that kind of experiment on soybean, you’re going to see that soybean gets about 60, 70% of its nitrogen from the air through that nodule symbiosis with rhizobia. If you do that on the regular corn here in the Midwest, you’re getting about 0.1% of nitrogen from the air, just because of what I was calling, the free-living fixation in soil. The result we got that was shocking for us, was that when we did that kind of experiment on the corn from Sierra Mixe, we see that starting from the second month, but mostly after the third month after planting the corn, up to the sixth month after planting, we see that that nitrogen, the different bars just represent different years where we did the experiment, we see that the corn gets about half of its nitrogen from the air. So which is almost like a soybean.
A soybean, as I told you, is like 60, 70% nitrogen from the air, and normal corn is 0.1%, so here, we’re at 50%. It’s a 500 times fold higher than normal corn. So, to be honest, for the first years, we were thinking that it was an artifact. We were thinking it’s impossible, it cannot be true, because we have never seen that in any cereal, and has never been reported. It’s almost as good as a soybean. So, well, we decided to still figure out why we were getting these values. Before I continue talking about nitrogen fixation, I need to let you know a little bit more about the corn, because not only it’s a tall corn, it’s like 15 feet high, when you look at the stalk, that corn is covered with roots, and you may be familiar with what we call the brace roots on corn. Typically, corn we grow here has two or three nodes with these roots that look like fingers, and they go back to the ground, or sometimes they are called anchor roots. The corn from Sierra Mixe has roots like that, but all along the stem. It’s covered with roots on the stem. We quantified that, and we compared physically the Sierra Mixe corn to another giant corn called Hickory King, and Hickory King is like the corn we grow here.
It has two or three nodes with these roots at the base, but we quantified how many nodes with roots we have in the Sierra Mixe corn, and you can see that after about 12 weeks, 12, 14 weeks, we have about eight to ten nodes with roots. And it means that the corn is definitely covered with roots up to here. You see these roots, some of these roots here have one, two, three. I don’t, I can’t see the other ones here. We counted how many roots total we can find on the corn at tasseling time, which is around that point, and on the Sierra Mixe corn, we find more than 125 roots in average, versus about 20, 30 on the normal corn. So that’s a bit surprising. Our corn is covered with roots. (laughs)
We looked at a little bit more the physiology of root development, and what we found is that if you take a conventional corn like the one that we have here, it’s producing aerial roots at what we call the juvenile stage. In the Sierra Mixe corn, it’s producing these roots at the juvenile, but also at the adult vegetative stage. And so that’s what we think is the main difference between the conventional corn, and the Sierra Mixe corn, is the ability to keep producing aerial roots, or nodes, with aerial roots, at the adult vegetative stage, and root production stops about at flowering time, at tasseling, when the plant has start flowering, the roots stop forming on the Sierra Mixe corn. So that difference between of when the roots are produced, we think is the main reason why that corn has so many roots. And we can easily see when the transition between juvenile to adult, we are looking at a wax that you can see here on the leaves, so it’s very easy to see when that transition happens, and clearly, the Sierra Mixe corn keeps producing roots, even at the adult stage, which is really unusual. Another unusual thing, keeps being unusual with that corn, is that when it rains, the roots are produced with gel. They are literally dripping with gel, which when I started growing that corn here in Madison, my Agronomy colleagues called me several times and saying that I had a problem with my corn, that it was contaminated, or covered with a fungus, or something weird. I said, “No, that’s normal.” (laughing), And that’s, it’s a gel produced by the roots. And each root, each of these aerial roots produces between 1.5 to two milliliters of gel, a lot of gel. We took a short movie of the gel, so that happens after half an hour after rain, to maximum one hour after rain. You have the roots literally dripping with gel. And so that corn drips, has all these roots that drip with gel, that’s weird. (audience laughing)
Yeah, so we use more techniques to try to, coming back to nitrogen fixation, we were really surprised by these initial results of having the corn getting half of its nitrogen from the air. And so we used another technique. I won’t go into the details of how it works, but the technique, the goal is to evaluate the activity of the enzyme, that enzyme, called the nitrogenase. And so it’s really, I said, that we can do on different parts of a plant, and so we tested the amount of nitrogenase activity in the leaf, in the stem, in the roots underground, in these aerial roots before rain without the gel, in the aerial roots after rain, when they were covered with gel, and we see a massive activity, nitrogenase activity in the roots with the gel. And to give you an idea of what that scale is, it’s almost as much nitrogenase activity as one of my alfalfa plants with all its nodules. So when we saw these numbers, we thought that yes, there is something really unusual there. So that difference between roots with gel, with gel and without gel, prompted us to look at the gel itself, suggesting that there is something in the gel. So we looked at the nitrogenase activity in the gel coming from Sierra Mixe, from plants grown in Sierra Mixe, and so we had our collaborators over there shipping us gel across the border. And it was stopped by the customs at least three, four times, and they were asking me, what’s that? I said, gel from corn, and they do not believe me, and they destroyed the samples, but in the end, we were able to get some samples of gel to Madison in 24 hours. And with other gel that’s collected in Sierra Mixe, or in Madison, we see a very similar nitrogenase activity, indicating that it doesn’t really matter where the gel is coming from, where the plant is growing, we see very similar activity, and very likely, bacteria in that gel fixing nitrogen, whether the corn is from the field in Madison or Mexico.
So the next experiment we did was to take that gel, and we found a trick to kill the nitrogen-fixing bacteria inside of that gel, and at the same time, preserve the structure of the gel, its viscosity, and you will see later that it matters. So what we did, we just froze and thawed several times the gel, and that actually kills the bacteria. And so when you kill that nitrogenase activity, these nitrogen-fixing bacteria, the level you have here, we were then able to reloculate the gel here with the bacterium call herbaspirillum, or here with a bacterium called Azospirillum brasilense. In other words, we can kill the bacteria, nitrogen-fixing bacteria in the gel, and then reloculate, and then we get nitrogenase activity again. What that means is that the gel is a good environment for nitrogen-fixing bacteria. We can kill the existing ones, and then add new nitrogen fixers, and they can fix again. The gel is just a good place for the fairly broad range, at least, I show you here, two, but we tested more, nitrogen-fixing bacteria. So that suggest that the gel is a good environment for nitrogen fixation. So why is that a good environment? I told you in introduction that one of the problems that we need to solve to have efficient nitrogen fixation, is oxygen, is we need to reduce the level of oxygen. And here we have a gel which is very viscous, and when we started putting an oxygen sensor, or probe, inside of the gel, just a few millimeters inside of the gel, we are inside of the gel at four, 5% oxygen level.
If you compare that to a solution of water, where you are at 20% oxygen, clearly here, the gel provides that microaerobic environment I was talking about, basically protecting the nitrogenase enzyme against oxygen. So the gel provides the oxygen protection that I was mentioning is so important, sort of the equivalent, if you want, of the leghemoglobin I was mentioning in soybean nodules. The other thing that the gel is providing, which is more obvious, is sugar. The gel is made of polysaccharides, and we were able to determine the structure of the polysaccharide, and this polysaccharides are degraded into simple sugars, and in particular, on galactose, which is here, and fucose. And these sugars, I won’t show you the data today, but these sugars basically feed the bacteria and help feeding these bacteria, so that they can have the energy to produce the, to feed the nitrogenase with all the ATP that the nitrogenase requires. So what the gel is providing is oxygen protection, and a lot of sugars to feed the bacteria and provide energy. And the fact, also, that this gel is sitting on the roots, even if it’s not a root underground, it’s a root in the air, these roots pump the nitrogen all the time, and so the gel itself has a low nitrogen content. The roots are basically pumping that nitrogen from the gel fixed by the bacteria. The last experiment I want to mention here, is kind of, sort of the ultimate evidence for us working on nitrogen fixation, that we have an efficient system, is that it’s almost the reverse of the one I was mentioning for the field.
Here, what we do, is that we label with a heavy isotope, dinitrogen from the air. So here, what we have is nitrogen from the air, which is labeled with 15N. It’s a heavy version of dinitrogen, and then we can then follow that dinitrogen from the air, into the bacteria, into the plant, can we see that, and that’s what we did. So to do that, you need to enclose, ideally, your system into a chamber, where you can pump 15N dinitrogen. For your information, 20 liters of 15N dinitrogen costs about $5,000, so these experiments are very expensive. And so we tried to do that with the corn, but you can imagine, it’s a giant corn, 15 feet high, it needs a massive chamber, and the experiment failed. We did it a few times. We were not able to keep the corn, a 15 feet high corn happy in such a closed environment. So we turned to a much simpler system, which is based on the fact that we know that fixation happens on the roots, on these aerial roots. And so we can simply cut, we were able to cut these roots, put them in tubes like that, and then in these tubes, we can inject 15N gas, that 15N dinitrogen. And then we can track where the nitrogen is going to. So this graph here, in red, that represents what– So here, it’s 14N abundance–
The samples have been incubated in 14N, and here, the samples have been incubated in 15N dinitrogen. So here are 14N dinitrogen, kind of the normal air, or here, it’s heavily labeled dinitrogen. In red, here, these two samples, that increase that you have between that point here and that point here, that indicates 15N enrichment inside of the gel. Instead, inside of the mucilage, and that makes sense. We knew, already, that bacteria in the mucilage can fix nitrogen. That basically confirms what we said before, that fixation happens inside of the gel. We then washed these aerial roots from the gel, and then looked at enriched 15N enrichment between here and here inside of the root. Well, these, we were excited to see an increase, but it’s not a very strong increase, and on top of that, you can be concerned that even if we are doing our best to wash away all the bacteria from the root, maybe there are still some bacteria attached, and that increase could be an artifact. The real evidence that nitrogen is not only fixed inside of the gel, but even transferred to the plant, is that, here, that blue, these two blue bars between 14N and 15N, and it corresponds to that axis here, and what we did here, is that we noticed that our roots, even if they are roots in the air, they are green, and they produce chlorophyll.
And so we thought, oh, why don’t we extract chlorophyll. Only the plant makes chlorophyll, even if there are still some bacteria left, the bacteria don’t produce chlorophyll, if you wanted a plant-specific marker. And then what we did, is that we extracted chlorophyll, and we looked at 15N enrichment inside of what is called phaeophytin, it’s just acidified chlorophyll, it’s just derived from chlorophyll, and what we saw is that we saw a strong enrichment of 15N inside of the chlorophyll. What that means is that nitrogen fixed in the gel by the bacteria, is transferred by the plant, and then the plant can assimilate that nitrogen into its chlorophyll. So the trick here of using chlorophyll is really to prove that the fixed nitrogen is taken up by the plant. So after that, yes, we were convinced that a lot of fixation is happening in that corn, it happens– It’s done by bacteria inside of the gel, the gel produced by aerial roots, and then, because it’s sitting on the root, nitrogen is transferred from the gel into the plant.
Of course, nitrogen fixation is done by bacteria, and very briefly, what we did, a large survey of what kind of bacteria are there, and we used many different techniques called metagenomics, which are really global techniques, and also isolation, isolating bacteria to see what kind of bacteria are there. We looked at, of course, the aerial roots, of course on the mucilage, and the many different parts of the plant. The list is actually much longer. I just want to show that graph. I know it’s maybe a bit obscure, but the color here shows the abundance of nitrogen-fixing bacteria that we found in different samples. And here, these are the samples that you are, here, that these columns here represent the sample of the mucilage. We can see that in the mucilage, we see a lot of nitrogen-fixing bacteria, and in particular, we think that the most abundant one, and probably important ones, of these bacteria called herbaspirillum. It’s not a big surprise, herbaspirillum is well known to be associated with corn. We find it everywhere in the Midwest, in Mexico. It’s a very classical nitrogen-fixing bacterium associated with corn. I won’t go into a lot of detail, but my colleague, Alan Bennett, in particular, and Bart Weimer at UC Davis, are doing a lot of isolation of the microbes, isolating many microbes from the gel, in particular, and testing their ability to fix nitrogen, of course, but also, to degrade the polysaccharide of the gel into simple sugars. So a lot of characterization and detail of these bacteria. The time I have left, I want to talk about two things. I want to talk about ongoing research, what’s the follow up on that work, and also more legal and societal aspects.
Ongoing research. I told you briefly that we are looking at the microbes. What kind of microbes are in that gel? What are the microbes that fix nitrogen? Can we find microbes that fix better than others by isolating gel from Mexico, but actually, also from here. We do experiments at West Madison, in particular. And by the way, a lot of experiments also during winter are done at the Biotron, (laughs) on campus, and we are looking at the molecular and cellular mechanisms of root production and gel production. Basically looking at mechanisms, looking at what are the genes that are involved. And along these lines of genetics, something that many people may, or growers, may wonder, is yeah, it’s great to have nitrogen fixation in that landrace of corn, but can I get that in my corn? And the answer is very likely, yes. ‘Cause we have started doing crosses between classical corn, commercial varieties of corn grown here in the Midwest, and what we call these Sierra Mixe landraces.
And so here, the technique that we used to evaluate nitrogen fixation, is the one that actually I showed you at the beginning called 15N natural abundance, or 15N dilution, very similar techniques. And what you are looking at here is that 15N abundance, and as I told you at the very beginning, in plants that can fix nitrogen in that technique, they have less 15N, because they get more nitrogen from the air. And so the Sierra Mixe accessions of landraces are here, these are different accessions, commercial varieties grown here, and we started crossing them. And here, these are the initial data of what we call the F1 generation, meaning the first progeny of a cross between the Sierra Mixe corn, and a commercial variety, and we can see that basically, we get a nice segregation of the phenotype. We have some individuals in the progeny that are like the commercial parents here, but you have some individuals, also, that acquired the traits of nitrogen fixation from the Sierra Mixe. And so that work, basically, the breeding work, the introduction of that trait of nitrogen fixation into corn that we can grow here in the Midwest, is still in progress.
As I told you, just because of the size of the growing season, we cannot take that corn directly from Mexico here, we need to do some breeding work. And once the breeding will be done, then we have lots of really interesting agronomy questions about how much nitrogen can we really save? At this point, I do not answer, when people ask me, how much nitrogen are we going to save with that technology? I say, I don’t know, because I’m comparing a giant corn to a normal corn, and these are really different beasts, and are so different that trying to evaluate at this point how much nitrogen we are going to save, is really too difficult. But once we have lines that are more similar to the kind of corn we are growing here, and have the trait, there we can start really having more practical agronomic questions. I want also to, other things that we are doing, we are looking at the evolution of the trait. I won’t have time to talk about that, but we have evidence that the trait is already present in a progenitor of corn called teosinte mexicana. Most of the corn that we grow here in the U.S. has material from teosinte parviglumis, a different species. But the trait of nitrogen fixation is not in parviglumis, is in teosinte mexicana. And so, we are looking at basically where this trait is coming from, and how it evolved.
And the last aspect is, can we apply that research to other cereals? Is that something specific to corn? And the answer is probably no. In fact, I’m showing you here, in that image here, is a cover page form a conference held in India in 1984, with a title, the conference was about nitrogen fixation in cereals, and they are showing a sorghum plant, with all its aerial roots, producing mucilage, just like here my corn here, and with the title, Nitrogen Fixation. Surprisingly, when you open that book, or when you look in the literature, there is strictly nothing about nitrogen fixation in cereals. So we contacted the author, or the organizer of the conference, and asked them, why did you have that image on the cover page, and why there is nothing published? And it turns out that they had, in fact, in 1984, data showing that they had a lot of fixation happening in these aerial roots of sorghum, but at the time, nobody believed them, and that project stopped in 1984. So it turns out that now we, seeing that, we came back to sorghum, and did similar experiments in sorghum, again, with the ICRISAT, because they have a wide diversity of sorghum, and while, indeed, you have a very similar system happening in sorghum, where these, you have a lot of fixation happening in these aerial roots. So the system that we discovered is not unique to that weird corn in Mexico. It’s also there in sorghum, and possibly, also in other cereals. And so that’s something that we are trying to explore.
With the time I have left, I have just a few slides about something that I think is also important, which is I would say, behind science, or beyond science, but really important, too. And it’s the social and legal aspects of the work that has been conducted. And that work was clearly in collaboration with, while working in Sierra Mixe, really required us to have a commitment to the community, and we could not just go there and take their corn. That would have been horrible. And I was really impressed and excited to work with Howard Shapiro, in particular, form the Mars company, on that aspect, that he really developed a relationship with that community, and in particular, making sure that the results, that the scientific we were getting here at UW-Madison, but also at UC Davis, were shared with the community, and explained to the community to show them, basically, the value of their corn, because it’s really their corn. And so, we are sharing the knowledge of the research, and basically sharing how their corn is really amazing, and could have a global impact in the world, in reducing nitrogen fertilizers, worth actually, it’s something that Howard Shapiro, but also Alan Bennett at UC Davis, did a lot. I was not involved in that part, but I was really proud that they were really, I mean, they were taking care of that. I think it’s a really important part of the project. And of course, informed consent agreement, so the community knows that we are working with their corn, and they know exactly what kind of experiments that we are doing on their corn, and also, of course, agreement making sure that they would benefit financially also from any discoveries.
The long-term relationship that was built, in particular, again, by Howard Shapiro, was built over decades. Howard had been working with that community for really, for several decades, and I think it’s a really important component. Connected to that, and making sure that the indigenous communities– Oh, I just want to mention that we were getting, I was getting here in Madison, samples of, so the bags I was getting, were labeled with the names of these people, and with their names. So when I was getting the corn here, it was not a random accession number with letters and number, it was really the name of the person, of the farmer. And so even if I do not go there personally, it was really an amazing experience. Very different from all the other projects I’m working on. I also want to mention that final aspect of the legal aspect. You may have heard that over the last year, the Nagoya Protocol was developed. And it’s a protocol that basically, the goal is to make sure that we are going to share the benefits of using genetic resources, from other countries, in particular, in a fair, equitable way, again, with the idea of returning the benefits of our research, using the genetic resources that people identified in Mexico, and bred in Mexico, in a fair way. So we developed, in order to get that corn here, we got the first international recognized certificate of compliance, IRCC, granted by the Mexican government, the very first one. And so it was, honestly, a difficult process that was a lot of work. And we also got the first one issued to the U.S., to a U.S. entity by any country. The Mars company in particular, and people in the group, were really proactive at making sure that the benefits of all the research is going to benefit the community in Mexico.
All right, I would like to acknowledge my colleagues, and of course, Howard Shapiro, who is here, in the middle, and it’s a pleasure to work with him. Again, he works for the Mars Company, and of course, had that amazing relationship with the Sierra Mixe farmers. Here, these are the people in my lab who worked on this project over the last 10 years, and this project, as I said, is a collaboration with several colleagues at UC Davis, Alan Bennett, in particular, and to also Jonathan Eisen, for all the metagenomics and study of the communities, Allen Van Deynze, and Bart Weimer. With that, I would be glad to take your questions.
(audience applauding)
Search University Place Episodes
Related Stories from PBS Wisconsin's Blog

Donate to sign up. Activate and sign in to Passport. It's that easy to help PBS Wisconsin serve your community through media that educates, inspires, and entertains.
Make your membership gift today
Only for new users: Activate Passport using your code or email address
Already a member?
Look up my account
Need some help? Go to FAQ or visit PBS Passport Help
Need help accessing PBS Wisconsin anywhere?
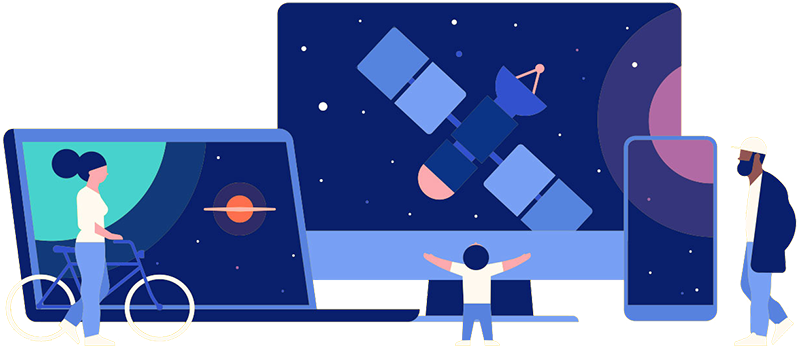
Online Access | Platform & Device Access | Cable or Satellite Access | Over-The-Air Access
Visit Access Guide
Need help accessing PBS Wisconsin anywhere?
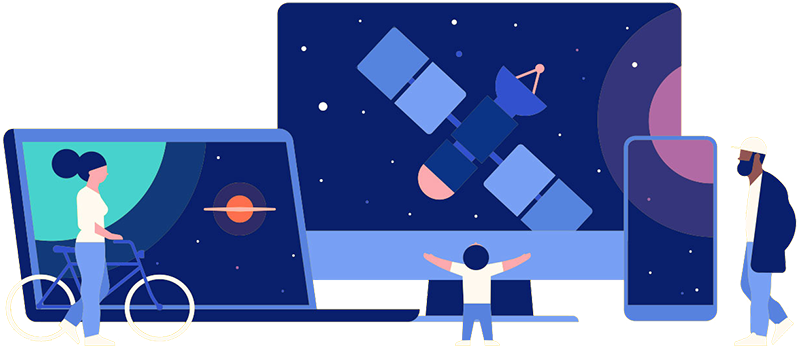
Visit Our
Live TV Access Guide
Online AccessPlatform & Device Access
Cable or Satellite Access
Over-The-Air Access
Visit Access Guide
Follow Us