– Welcome everyone to Wednesday Nite @ the Lab. I’m Tom Zinnen, I work at the Biotechnology Center at UW-Madison, I also work for the Division of Extension and for Wisconsin 4-H, and on behalf of those folks and our other co-organizers, PBS Wisconsin, the Wisconsin Alumni Association, and the UW-Madison Science Alliance, thanks again for coming to Wednesday Nite @ the Lab. We do this every Wednesday night by Zoom, 50 times a year. Tonight, it’s my pleasure to introduce to you Rebecca Hutcheson. She’s a graduate researcher at the McArdle Center for Cancer Research here at UW-Madison. She got her undergraduate degree from Mercer University in biochemistry and molecular biology. And then she came here to study the Epstein-Barr virus and its role in causing Burkitt lymphoma. She’s also interested in any virus that has the potential for causing a global pandemic, and that’s one reason she shifted her research recently to studying the COVID-19 virus. Tonight, she’s gonna speak to us about making the COVID-19 virus safe for research. Please join me in welcoming Rebecca Hutcheson to Wednesday Nite @ the Lab.
– Thanks for that introduction, Tom. So I wanted to start today by describing the reasons that I find myself doing this research in the first place because I never thought that I would be studying a virus that’s currently causing a pandemic. So like Tom mentioned, I am in my fifth year of graduate school at Bill Sugden’s lab, and we actually study a different virus that’s called Epstein-Barr virus. And about a year ago, at the beginning of the COVID-19 outbreak in the United States, our lab collectively decided that we had the expertise to be able to make a contribution to the scientific community. And so even though the virus that the Sugden lab studies is quite different from the coronavirus that causes COVID-19, we know that the experiments that are used to study viruses in the lab are pretty similar. And so we said to each other, “You know, hey, we really know a lot about this realm “of virology and we can help from a research standpoint. ” And virology is just the study of viruses. So we happen to be experts in that area, especially in the terms of molecular biology research. And so that’s what we’re doing as a lab. We are using our skills to contribute to this public health crisis.
And the second reason that I am finding myself doing this research is actually kind of personal. And so I was a pretty weird kid, I guess, and I was captivated by viruses. And I was about 10 or so, I was super excited because I had received these giant coffee table books about disease and viruses. And I remember just poring over the pages, captivated with, you know, these microscopic entities and how they affect humankind. And so my fascination with viruses has really stuck with me through my education until now. And you know, the viruses that are listed in the book, they don’t include the virus that causes COVID-19 disease. And so to even be able to contribute to this field of knowledge that’s you know, very novel and we don’t know a lot about it historically, it’s very humbling to be able to add to the field of knowledge that is currently being built. And so myself and my lab mates are doing that right now. And so I want to describe today the project that we’re working on, and that project is trying to really make the virus that causes COVID-19 safer for researchers to study in the lab. But before I get into that project, I would like to introduce the lab who have been in and out for the last eight months.
And these are the members of the Sugden lab who are working right now on the COVID-19 project. So we have Rup, Mitch, myself, Thejas, Quincy, and Bill. And Bill is our principal investigator. And so now as you listen to me you know, describe the research that we’re doing, you now have faces to put to the work that’s being done. And I think that’s really important. And so I wanted to recognize everyone right up front so that you know that even though you’re seeing my face today, you have everyone else in your heads that has been also working on this project. And so I wanna thank them too for allowing me to present this work you know, for all of us today. So as I mentioned, the research that we’re doing involves making the coronavirus that causes COVID-19 safer for researchers to work with in the lab setting. And before I get into the details of that project, I want to kind of go over some background for three key things that we need everybody to know. So number one is the virus that causes COVID-19, which is called SARS-CoV-2.
And then we’ll talk number two, about that virus’s life cycle. And then number three, we will talk about the facilities where that virus is currently studied. So the first thing I wanna introduce is that virus that causes COVID-19, it’s called SARS-CoV-2, and we’re gonna break down the name ’cause it is a long name, but it just stands for, SARS stands for Severe Acute Respiratory Syndrome, because it’s just a respiratory illness. Coronavirus because that’s the type of virus that the virus is. It’s the virus family. And the number two because there was a very similar virus that caused a much smaller outbreak in 2003 that was given the name SARS-CoV. So in general, most viruses are made up of two parts. We can think about the outer shell and the innards. And this outer shell of SARS-CoV-2 is made up of different proteins, one of which is called spike. And these spike proteins are actually common to all coronaviruses.
And they actually gave the virus family its name because the word coronavirus is derived from the Latin word corona that actually means crown. And so these spike proteins are like the spikes on a crown, and they allow for the virus to enter and infect human cells. And it’s actually, the spike protein is what many of the current vaccines are targeted against. So spike and the other proteins, that orange-colored membrane protein that you’re seeing and the yellow-colored envelope protein make up the outer portion of the virus that we’re gonna collectively call the shell for our purposes here today. And the shell, inside the shell is the virus’s genetic information. So the virus genome is made of RNA, and it’s just another type of genetic material, just like humans, our genetic material is DNA. And just like humans have genes that code for things in our DNA, like what eye color you have or whether you are lactose-intolerant, the virus’s RNA genome has its own genes. And those are gonna become important for us to talk about a little later. And so I’m gonna use the word genome a lot, and I wanna make sure that everybody knows what I’m talking about when I say the word genome, so I’ll define it for you. The genome is just the instructions to make all the components of a living thing, whether that living thing is a person or a virus.
The genome can be made of both DNA or RNA. And you can kind of think of it like a cookbook. So it’s instructions to make different genes or recipes, and you can think of the genes as recipes, and the cell is gonna read these recipes or these genes, and it’s gonna make whatever the instructions say. And so with that being said, let’s move on to the second thing, which is the life cycle of this virus. So the SARS-CoV-2 life cycle sort of starts out like this. Since the virus actually can’t make more of itself, like most, actually all viruses can’t make more of themselves. They need a host to replicate. And so I’m gonna use the example of a human lung cell here. And I just want everyone to know that this diagram is absolutely not to scale. So the virus attaches to the cell using that spike protein, much like the way that a key would fit into a lock.
And doing that allows the virus to break into the cell and release its genome, which is that viral RNA. Okay, and this foreign RNA can’t really be distinguished by the cell’s machinery. So the cell actually begins to read the virus’s genes and to make the proteins that make up that outer shell. And that’s kind of happening on the top of the diagram. The virus’s newly-made proteins can then come together with the newly-copied viral RNA, and that’s happening simultaneously, kind of in the middle there of that diagram. And so now all that’s left is for that viral RNA to come together with those outer shell proteins and they’re gonna assemble into new virus particles, and then the newly-made virus can leave the cell. So the simple cartoon that I showed you is one virus particle infecting one cell and releasing one new virus particle. But in reality, what happens is it’s more like on the order of a thousand new virus particles are released from an infected cell. And all of that chaos causes the body to call on the help of the immune system. And it’s actually one of the reasons that humans can get so sick from SARS-CoV-2 infections.
And so as researchers, we’re studying this virus to help develop vaccines and treatments to treat people with COVID-19, but the researchers also have to protect themselves in the lab from infections that may occur. And so that’s gonna bring me to my third point, which is the facilities that SARS-CoV-2 is being studied in. And so you can categorize research labs into different biosafety levels, and these levels are in place to regulate what kind of organisms that scientists can work with in a lab facility. And they are designed not only to protect our, you know, us, the laboratory personnel, but also to protect the surrounding environment and the communities. And these levels are ranked one through four, and one is the lowest and four is the highest and the most stringent. And I wanna run through these pretty quickly so that everybody kind of knows what’s going on at each level. So biosafety level one, scientists can work actually directly on a benchtop with microbes that cause mild disease, things like yeast or E. coli. At level two, there are more precautions in place for scientists who are working with microbes that cause mild disease in humans or pathogens that don’t spread through the air. And those examples include the viruses that cause hepatitis, the parasite that can cause malaria, and also things like salmonella bacteria.
And so the next level up, level three, is for research involving microbes that cause serious disease and they can be infectious through inhalation. So examples of level three pathogens would include yellow fever virus, HIV, and the current plague, SARS-CoV-2. And finally, biosafety level four is reserved for only the most dangerous agents, those that cause severe or even fatal disease in humans and those that really have no cures or no treatments against them. And so examples of biosafety level four pathogens would be things like Ebola and Marburg, and those viruses are very dangerous. And so scientists working with those viruses and microbes have to be in those sort of specialized, pressurized space suits that you see in the movies. And those are real, and they also have a whole host of other precautions that you don’t see in the movies that makes these facilities incredibly rare. So there’s actually only nine biosafety level four facilities in the entire United States, compared to some odd thousands of biosafety level two facilities. And so I wanted to explain all of this because SARS-CoV-2, the virus that causes COVID-19, has to be worked with at biosafety level three. And that is a big deal for two reasons. The first reason is that it’s really hard to do research at this level.
Scientists have to be specially trained to work with these pathogens. They have to wear all the necessary gear and the respirators while working, they have to shower to leave the lab to decontaminate. And so it’s really a lot to get everybody working in the lab and get experiments going. And there’s a lot of regulations surrounding samples and what you can take in and out of the lab. And so that makes it really difficult to work in this space. And the second reason that working at biosafety level three is such a big hassle is because the number of facilities that are able to work at this level is really rare. So for example, at the University of Wisconsin-Madison, there are probably about 500 biosafety level two labs, but compared to fewer than 10 biosafety level three labs that have been approved to work on SARS-CoV-2. And so they’re actually have to be built to be able to contain the pathogens because they need specialized airflow systems, they need filtration systems, double doors, they need decontamination areas. And all of that has to be planned prior to the construction of a building. And so biosafety level three facilities can’t just pop up when we need them to.
And so these two things are limiting the amount of research that can be done right now with SARS-CoV-2 infectious virus. And as we all know, it’s really important to be able to study this virus and learn more about it so that we can you know, develop vaccines and treatments against this virus that causes COVID-19. And so you know, we in the lab recognize this, as everyone has. And we said, “What are we gonna do? “We have a lot of expertise, but what can we do “as a lab to help put an end to this pandemic?” So it’s not feasible for anyone to try to build more biosafety level three labs and train more scientists to work in them. But what if we tackle the other end of the problem? So what if we tame the virus itself? So our lab’s goal is to make a safer version of SARS-CoV-2 so that researchers can study the life cycle of the virus, but it’s gonna be able to be worked at in biosafety level two instead of level three. And this would mean that lots more scientists could study SARS-CoV-2 and accelerate the rate of what we learn, the treatments that we could develop, and the patients that could potentially be saved. So from here on out, I’m gonna call the safer version of SARS-CoV-2 that we’re developing CoV-2. def, where def stands for defective. And I’m gonna explain how it’s defective in a little bit. The CoV-2.
def virus that you see in the coming diagrams is gonna be green in color. And it’s different biologically from the original SARS-CoV-2 virus. So we’re going to build the safer derivative so ourselves and other researchers can add to what’s already being done. So it is possible to study smaller portions of the SARS-CoV-2 life cycle at biosafety level two and that’s being done now, but that really breaks up the biology of the entire life cycle. And it can become difficult to see the bigger picture. So if you think of the virus’s life cycle as an orchestra and think of each portion of that life cycle as an individual musical instrument in that orchestra. For example, we can examine a violin in detail. We can learn how it works. We could take it apart. We could see how it sounds, but we don’t know what that sounds like as part of an orchestra.
And so we would like to be able to study the entirety of everything together. And for example, you know, scientists have learned a lot by studying the spike protein in isolation as a little unit by itself. And that’s been really valuable, but it is impossible to study all of viral infection and the human immune response to SARS-CoV-2, or test different treatments for COVID-19 if what you’re studying is a piece of something that is actually working like a biological orchestra as a whole. And so what we can do is create this safer version, which is CoV-2. def, that’s gonna allow scientists to study most of the virus life cycle all together. So to do this, let’s go back to the life cycle of SARS-CoV-2, only this time, we have our safer derivative CoV-2. def in place. So CoV-2. def acts very similarly through the virus life cycle until the end. And if you look closely, I’ve kind of put a red X over the envelope and the membrane structural genes, those orange and the yellow bits, which indicates that those have been left out of the genome.
And what that means is that when the virus is making all of its proteins to build the outer shell, two of the crucial instructions to build those two proteins are missing. And so without those two components, that’s part of the vital proteins necessary to build the shell. And so the virus particle isn’t able to assemble into new virus particles, and that means the end of the life cycle doesn’t happen. And that means that it’s a dead end for CoV-2. def. So now if we had CoV-2. def, scientists could study the entirety of entry and infection and replication, but now, CoV-2. def virus is gonna die inside this cell. And so overall, CoV-2. def derivative is competent for replication because the CoV-2.
def RNA genome can be copied and almost all the viral proteins can be made, but it’s propagation-defective, which means it’s not going to spread to other cells and infect them because it’s not releasing new viral particles. So in the next part of the talk, I’m gonna describe how we can engineer a new virus from the original SARS-CoV-2. And the idea of developing a new derivative that’s safer to work with for a difficult to work with virus in the first place is not a new idea. It’s actually been done with many different viruses, including HIV, which if you remember from before I told you is worked at at biosafety level three, when you use infectious virus. And so back in the ’80s and ’90s, scientists were in a very similar situation that we are all in today, which is they were trying to work with a virus that was novel and it was causing outbreaks all over the world. And it had to be worked with in biosafety level three because of safety concerns. So luckily in 1998, researchers were able to develop a safer derivative of HIV that was able to be worked with at biosafety level two. And it eventually became an incredibly useful tool for experimentation and for different applications that we use, actually we use in our lab kind of on a regular basis. And so it’s been very valuable to not only study the virus itself and prevent outbreaks, but also for the scientific community. So another example of another safe derivative is another coronavirus that’s called MERS-CoV.
And MERS-CoV caused a small outbreak in 2012 in the Middle East. And we know that we can be successful with SARS-CoV-2, just like scientists were successful with MERS-CoV. And we know that they had built a safe derivative and we can also do the same thing. And especially because they are both coronaviruses, we know that this can be done. And so these derivatives are safe, they make research easier, and they help accelerate the progress of discovery. So with this safer version, scientists are gonna be able to do a lot of the assays that are now performed at biosafety level three and move them down to level two. For example, one of the things you may have heard a lot about in the news are antibodies, specifically antibodies that our bodies make that work against the virus to neutralize it or to kill it. So patients that have been infected with SARS-CoV-2 and recovered have developed these little Y-shaped molecules that are called antibodies that act directly against the virus. And one of the ways to check if a patient has made antibodies to something is to take a serum sample from that patient and expose it to infectious virus in a tube inside the lab. And scientists can learn a lot about studying these neutralizing antibodies that people are making.
And if we can learn enough about a neutralizing antibody, we can actually produce it in the lab and give it as a treatment to those COVID-19 patients who are actually failing to make their own antibodies. So right now, these assays have to be done at biosafety level three because we have to use infectious SARS-CoV-2, but CoV-2. def can be used in the same way, but it’s actually safer to work with. So another thing that would be incredibly useful for the scientific community is to use CoV-2. def to search for an inhibitor of the viral replication step. And it can be used as a potential treatment to help those who are infected with SARS-CoV-2. And these are just two examples of the many applications that we could use for CoV-2. def. But right now, all these things are currently being done either with portions of the original virus or at biosafety level three, which again, is really difficult to work in. So they could be made easier using CoV-2.
def virus. Not only that, but the number of laboratories that could work on SARS-CoV-2 research would increase because we are limited right now by the need for containment level. We need to be at level three. And so imagine if some thousand more labs were able to work and study SARS-CoV-2. The research that we’re doing would accelerate and perhaps we could learn crucial information that would be needed to help stop the pandemic. So now we’re gonna switch gears. So hang on, and we’re gonna talk about how we in the Sugden lab are building the CoV-2. def virus. So remember, we want to engineer the CoV-2. def virus to be able to perform one round of infection on a cell but not get out of that cell.
So unfortunately for us, it’d be really cool if we could just throw together the viral proteins and the viral RNA in a tube in the lab and get the virus to come out, but we can’t do that. So we need all the components, but we need a more sophisticated machine to build them. So how about we use the natural virus builder, which is the host cell. So we can supply all the components of CoV-2. def to a cell and with some manipulation of biology, we can produce our virus, and it would almost be like a little virus factory for making CoV-2. def. So making synthetic virus is a really common molecular biology technique that’s done on a regular basis. And so it’s used for a lot of different applications, and these techniques of making virus in cells is not a new technique, but the virus that we’re gonna produce is gonna be new. So the cells that we’re gonna use to make CoV-2. def virus are called 293 cells, and they’re derived from human tissue that’s been immortalized in the lab.
And that means that they’re gonna continue to divide in the dish that we keep them in indefinitely. And so we grow them in Petri dishes inside of incubators that are kept at the human body temperature, and the cells adhere to the bottom of the plastic plate. And they’re supplemented with a red-colored liquid medium that supply the cells with all the necessary nutrients and growth factors that they need to grow in the dish. And so the 293 cells that I’m showing you in the image have been fluorescently colored so that you can see the nucleus of the cells in red and the cytoplasm in green. In my cartoon of the 293 cells is that blue blob that’s in the dish over there. And I’m gonna use the image of the 293 cells that’s a cartoon from here on out so that we can kind of keep track. These cells are going to house, or they’re gonna be supplied with all the components that are necessary to make CoV-2. def virus. So I’m gonna talk about the components of CoV-2. def in two parts.
So I’m gonna talk about the viral genome and then the structural proteins that are gonna make up the outer shell of that virus. And I’ll talk about them in the context of CoV-2. def, but I’m actually gonna use those colored versions of the outer shell proteins so that we can follow them and we don’t get confused with the same colors. So if you remember from earlier on, we’re gonna make CoV-2. def virus unable to make new virus in the cells that it’s infecting, but CoV-2. def has to have all the components of the original. And one important point that I really wanna clarify is that we’re using what we know already about SARS-CoV-2, which is the original virus, to build CoV-2. def, but we’re actually starting entirely from scratch. We have no starting material. And so we know the genome sequence of the original virus, and we can read those instructions and use that information to build new instructions so that we can make CoV-2.
def. So with that in mind, let’s delve into the first component, which is the viral genome that we’re gonna supply the 293 cells with. So before we get into that though, here’s a key concept that I need everyone to hang on in to their brains. So in biology, the usual order of things goes like this. We have DNA that’s read and made into RNA. And then that RNA is made into protein. And these proteins are what make up almost everything in the cell, and therefore our bodies and almost every living thing on Earth. So this is the central rule of biology for our purposes today. It goes DNA, RNA, protein. So the SARS-CoV-2 genome is made of RNA, but RNA is unstable and it’s actually really hard to work with in the lab when you compare it to DNA.
Both RNA and DNA can be read by the cell, and the instructions are written to give the same protein products. And so we’re gonna actually use DNA to make the CoV-2. def genome instead. And this is still gonna work as the original virus would because the DNA can be turned into RNA, and then that’s gonna serve as the virus genome would naturally. So we need to rewrite the new instructions for CoV-2. def using the genome sequence of SARS-CoV-2. So let me describe how we’re gonna do that and how we design these new instructions. So we’re gonna zoom in on the genome. So instead of those sort of zigzag, the circular zigzag, I want you to think of the genome stretched out so that we can read it left to right like a sentence. And the sequence of the DNA genome is made up of four letters, A, T, C, and G, and certain combinations of these four letters are read by the cell as genes, which I’m representing as those colored blocks.
There are a lot more genes and elements in the CoV-2. def genome that we’re building, so these elements have been simplified and grayed out so that we can focus on the key genes. Not that these grayed-out bits aren’t important, though. They actually serve as the instructions for a lot of key proteins that the virus needs, one of which is actually copying the virus’s genome. And so my simple genome that I’m showing you today of the sort of rectangles is incredibly oversimplified. We only have time to talk about a few genes today, but the virus’s complexity is something to appreciate when you consider what we’ve designed. And so as the cell machinery reads these genes, we’re gonna make the DNA into RNA and then the RNA into protein from there. And so we started first by designing the sequence of CoV-2. def on paper using a computer program, where we can see all the genes of the original sequence and then decide which parts we wanna keep from the original instructions to write the instructions for CoV-2. def.
So imagine that we have copied those genes into a new document, and you’ll notice that we’ve left out the envelope and the membrane genes, those orange and the yellow-colored instructions that are eventually going to make their respective proteins in the outer shell of the virus. By not including these components from the original instructions, CoV-2. def that carries this genome is gonna be unable to make new virus particles in the cells it infects because it doesn’t have those instructions to make these two proteins. Okay, so that’s really key. In place of the envelope and the membrane genes, we’ve added back some special genes in place of the ones that we got rid of. And these genes are gonna give rise to proteins that are actually colored. One is the green fluorescent protein that was isolated from jellyfish, and one is the same enzyme that actually makes fireflies glow, and that’s called luciferase. So by adding these genes in, we can visualize anything our CoV-2. def virus infects by looking for green color and the luciferase enzyme. So another key element that we’ve added to this CoV-2.
def genome is an element that allows us to turn on and off all the elements on the entire stretch of DNA. And it’s this little blue site that I’m showing you, and it’s called a Tet/KRAB element. And it works because it’s inducible. So you can think of it like a light switch, which is usually turned off until we add a drug that’s called doxycycline. And the way this works is a really cool piece of biology. So let’s think of our string of DNA, but since we’ve included the Tet/KRAB element in the design, it acts like a signal to other proteins in the cell. And these other proteins actually sit on top of the DNA, right in the way. And these proteins prevent the DNA from being read by the cells’ reader machinery so that none of the genes that are on this piece of DNA can be read because the machinery has to start at the beginning of the genes. And so without doxycycline, these proteins are in the way and nothing is being made ’cause they’re sitting on the top of the DNA. But when doxycyclin is added, it actually binds and removes those proteins that are in the way.
And then that allows the DNA to become accessible to the cells’ reading machinery, which allows for the genes to be read and for those proteins to eventually be produced. And so this is one of the ways that we can manipulate the biology of the CoV-2. def genome so that it’s safer to use. So overall, this means that if we have the CoV-2. def genome inside 293 cells, the only way that CoV-2. def virus is gonna be made is if we add doxycycline to those cells. If we take away that doxycycline, the production of the CoV2. def virus is going to stop. And doxycycline is a really easy thing to work with. It can easily be added to the cells because it’s a drug.
So the molecule that you’re seeing is actually dissolved into a liquid. And then that liquid, a small amount can be added to the red-colored liquid media that the cells live in. And the doxycycline is a molecule. So it’s free-floating, and it can diffuse into those cells and it can go to the DNA and it can then remove those proteins that are sitting in the way on that little blue site. And then the genes can then be read by the cells’ machinery and we can get the proteins that we wanna produce. When we wanna take away the doxycycline, all we do is just suck off the old media and we put new, fresh media on that doesn’t contain the doxycycline. So now that I’ve described all of the genes and elements on our CoV-2. def genome, I wanna tell you how we are physically building this lengthy molecule. So the original SARS-CoV-2. genome is about 30,000 letters in length.
And we need to build a DNA molecule that’s actually more than 40,000 letters in length, and we need to build it from scratch. And so that’s a really big undertaking. So to do this, we can order fragments of synthetic DNA from companies, but those actually can only be built to about 2,000 letters in length. So we have to order those fragments and then piece them all together in the right order using a process called DNA ligation. And so if any of those fragments are incorrect or if any of the ligation reactions introduce an error, we have to correct that error. So the cell reads the DNA sequence letters in groups of three, and one letter change can make a really huge difference in the way that protein is produced. So for example, if you misspell the word bar to the word war, you are really changing the context of what you’re trying to say, and that’s true for the DNA sequence as well. So we’re working really hard in the lab to have a perfectly printed DNA fragment. And I will tell you right now, this has not been an easy thing to accomplish. We are actually still working on it, which I’ll tell you about a little later, but let me just describe one of the snags for you so that you kind of get an idea of how hard this has been for everyone working on it.
So as I mentioned, the fragments have to be put together using ligation, but before we get to that step, all of the fragments have to individually be put into bacteria and grown up so that they can be amplified. And so we’ve found that some of the pieces are actually poisonous to the bacteria that we’re trying to grow them in. And that took us like a month to figure out, and then we had to manipulate the biology of the bacteria so that we can put our fragment in so that we could then you know, grow up the fragments and use them to ligate to the others. And so, you know, it has been a wild ride, but we are about, I would say 60% done with building this really lengthy molecule. And we’re working really hard to finish it off right now. So now we’re gonna talk about the other part of the DNA or the other part that we need to make CoV-2. def virus, which are the structural proteins that make up the outer shell of the virus. And at this point, we’ve talked about putting the genome, the CoV-2. def genome into 293 cells. And if the genome is the only thing that we have in the 293 cells and we turn on those genes using doxycycline, we’re going to make the DNA into RNA and that’ll give us the RNA genome.
And then it’s also gonna produce the proteins and it’s gonna produce spike, that green fluorescent protein, luciferase, and all those other grayed-out proteins that we don’t have time to talk about. So the missing proteins that we need to make virus are the yellow, sort of envelope and the orange membrane proteins. And since we purposefully left out their DNA in the instructions, we need to supply those two proteins to the 293 cells if we want them to make virus for us. And so to make our CoV-2. def system safer, we’re gonna give these two proteins to the 293 cells in two different ways. And I wanna talk about the orange protein first, that membrane protein. And so the membrane protein is actually the most abundant protein in the viral particle. So we need a lot of it to be made for all the virus particles to be made for CoV-2. def. And to supply the 293 cells with orange membrane protein, we’re gonna use the same doxycycline inducible system because they, the cells already contain that system.
The membrane gene is gonna be on a different piece of DNA inside these cells, not on the CoV-2. def DNA genome. And this makes the system safer by using two pieces of DNA instead of one. And so first, we introduced this membrane gene directly into the cells using a process called retroviral transduction. So remember, these 293 cells have their own DNA. They have 46 human chromosomes because they are human cells. And so we were able to integrate this piece of DNA into the 293 cell DNA. And so on this piece of DNA is also the blue site that allows for induction using doxycycline. And so when doxycycline is added to these cells, the light switch is flipped on and then we get membrane protein made. And for simplicity, I have been calling the membrane protein orange, but in reality, it’s actually just clear, it doesn’t have a color.
And so that we could follow them in the cells, we actually included a fluorescent protein just after the membrane protein that’s called mCherry, and mCherry is going to be red. And so in the presence of doxycycline, we’re gonna make mCherry protein as well as that membrane protein. So having the mCherry component in the cells allows us to quickly check whether our doxycyclin system is working. So I’m gonna show you two images of the 293 cells that contain the integrated DNA piece that encodes the membrane gene and encodes the mCherry gene. Both of the photos of the 293 cells on the left are containing the integrated DNA piece. But on the top panel, the doxycycline has been added to those cells. And so you can see that those cells turn red because they’re making the mCherry protein, which also means that they’re making the membrane protein. And through this test, we know that our cells can be successfully making the membrane protein that we want. We do have other ways to tell if the protein is being made in these cells, but this is the most visual way to see that. And it’s the most, it’s the easiest way to show you here today.
So now the final piece of the puzzle, or in this case the CoV-2. def viral particle is the envelope protein that’s yellow in this case. And this protein is gonna be provided through direct RNA transfection. And so what that means is that we make the 293 cells take up RNA directly. And from there, it can be translated into the envelope protein. So of course, we couldn’t just snap our fingers and make this molecule. We had to build this molecule by taking a DNA molecule and introducing that into cells, having the cells then produce RNA, and then we had to purify that RNA piece out of those cells and put it in a tube. And then, only then we could introduce this RNA molecule into the 293 cells and have them produce our envelope protein. So once we’ve done that, the RNA is actually used by the cell and it’s degraded, meaning that it’s not gonna stick around and accumulate in these cells. So at this point, you may be wondering why we’ve kind of made life really difficult for ourselves seemingly, because we have many different methods for supplying these cells with the components to make CoV-2.
def, but it was actually intentional. So by using both DNA and RNA on different molecules, it actually makes the system very safe because there’s no way that we could somehow combine these components together to make the original SARS-CoV-2 virus. Biologically, it’s just not possible for these things to come together to make the original. And that’s really important, especially because we want our system to be really safe for researchers to use. And this is true because we’re using both DNA and RNA, they’re in different molecules, but also two of the elements are inducible with doxycyclin, meaning that we’re not gonna make anything in these cells unless we give the cells doxycycline, which we can control. So now I’ve described all the pieces for you. So let’s put it all together. We have the CoV-2. def DNA genome, which is a really long piece of DNA that contains all the instructions to make virus, except for those orange and those yellow genes. So because the virus cannot be made without those two proteins, we’re gonna supply them in two different ways.
The membrane through a DNA that’s been integrated into the 293 cells, and then the envelope through direct delivery of RNA into the cells. And so we only supply that yellow envelope RNA when we’re ready to make virus. And then we’re gonna add doxycycline to the cells, which is gonna cause the switch to flip on. And then we make orange protein. We’re gonna make all the proteins encoded on the CoV-2. def DNA genome. And then we’re also gonna copy that DNA genome into RNA. And so now that RNA genome, which is the natural state, can be encapsulated by the outer shell proteins, and then voil! We have our CoV-2. def virus, and that’s gonna be secreted into the media of those cells. And so then we can actually just collect that media and put it into a tube and we have our CoV-2.
def virus produced. And this virus can now be used to infect other cells by putting the media which has the virus inside of it directly onto new cells. And if these new cells are able to be infected, CoV-2. def virus is gonna enter, it’s gonna uncoat, and the RNA genome inside is going to provide the instructions to make all the proteins, except for the orange and the yellow membrane and envelope. And instead, that’s gonna make the green fluorescent protein and the firefly luciferase. So in this way, we can actually visualize which cells are infected with CoV-2. def because we see the green fluorescent protein and the luciferase. And that’s a really useful tool for scientists to use when performing different assays because now there’s actually a direct readout in color, which can be measured with instruments or microscopes. And so here’s an example of cells that have been infected with another coronavirus that we work with in the lab. And this virus is called NL-63, and it’s another coronavirus that is much more benign.
And it only causes mild colds in humans or it causes nothing at all. And so we’re using NL-63 as a model to study infection in this case. And we can’t easily tell from the image on the left that’s using Brightfield light, which cells are infected with the virus. The picture on the right is the same. It’s the same picture, it’s the same cells, but now you’re looking at it through a fluorescence microscope, which tells us that the cells are making green fluorescent protein and thus are infected with the virus. And this is gonna let us study the cells as they grow and we can monitor the infection of these green cells. So as we come to the end, I wanna describe what we’ve accomplished so far. And I have tried to lay all of this out for you so that it’s pretty straightforward, such that you know, this protein is gonna do this and this DNA does this and we put this piece here, but in reality, biology is quite messy. And usually the first time you try something, it’s not gonna work. And most likely, the 15th time you try something, it’s not gonna work.
And so the most challenging piece of this project by far has been building that CoV2. def DNA genome from those fragments that I described, and we’re about 60% done with that guy. And so then the orange membrane and the yellow envelope proteins, getting those to be made inside of the cells with their respective systems are both at about the same level of completion, probably about 85%, and right now what we’re doing is we’re testing those components to make sure that those proteins can come together with the other proteins in the outer shell and make empty virus particles. And that just means that we aren’t putting any of the genome inside. We’re just kind of creating this empty shell and we’re optimizing the steps that we’re taking to create those shells so that when we do eventually stick the genome in in our system, we have the perfect conditions and the right settings so that we can make a lot of virus when we’re ready to do so. So all of these things are currently being worked at at biosafety level two and they’re being worked at separately, but whenever we’re ready to put all of them together, we’re actually gonna move to biosafety level three. And we’re going to test that the CoV-2. def virus that’s produced from these cells is not able to infect cells for more than one round. And that those virus particles that get in stay inside that cell and they die inside that cell. And so only then are we gonna be ready to share this research and these components with the world so that more research can be done on SARS-CoV-2.
So overall, we have designed a system to help accelerate research by allowing more to be done at a biosafety level two instead of three. It’s a system that is gonna protect scientists from having to work with the original SARS-CoV-2 virus. And it’s a system that can be handled by researchers to be safer biologically. And so CoV-2. def can provide a faster, safer solution to research on SARS-CoV-2, which hopefully will help to end the COVID-19 pandemic and accelerate the rate at what we learn. So with that, I’d like to close by giving a huge thank you to the University of Wisconsin-Madison, especially the Office of the Vice Chancellor for Research and Graduate Education, and the McArdle Laboratory for Cancer Research for their support. And we wanna thank our colleagues who have been working really closely with us to get this project off the ground. And so I wanna say thank you to them. I also wanna thank the National Cancer Institute and Promega for their support. Of course, I wanna thank Wednesday Nite @ the Lab and Wisconsin PBS and all of those organizers for helping me prepare and record this talk and for the opportunity to really, to present here today.
And so I sincerely hope that I have taught you a little bit about molecular biology, a little bit about SARS-CoV-2, and of course about the CoV-2. def derivative that’s gonna be safer that we’re building right now. And also why it matters, why it’s important to do so. So in the Sugden lab, we’re working really hard right now. We are day in and day out in the lab, and we’re hoping to complete the CoV-2. def project in the coming months, so that we could share this research and share these components with other people who would like to do COVID-19 research, but can’t because of containment requirements. And so we’re really excited about this work. I can’t stress that enough. If everyone were here, they would say the same thing, and we know it can make a real difference in helping us learn about SARS-CoV-2 and potentially help to end the pandemic. And so now I want to thank you for listening.
Thanks!
Search University Place Episodes
Related Stories from PBS Wisconsin's Blog

Donate to sign up. Activate and sign in to Passport. It's that easy to help PBS Wisconsin serve your community through media that educates, inspires, and entertains.
Make your membership gift today
Only for new users: Activate Passport using your code or email address
Already a member?
Look up my account
Need some help? Go to FAQ or visit PBS Passport Help
Need help accessing PBS Wisconsin anywhere?
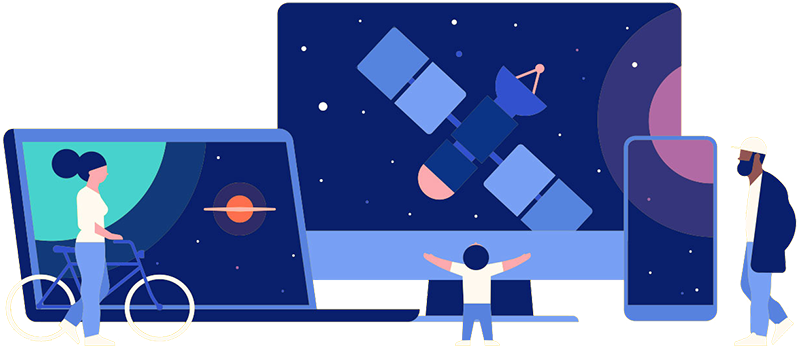
Online Access | Platform & Device Access | Cable or Satellite Access | Over-The-Air Access
Visit Access Guide
Need help accessing PBS Wisconsin anywhere?
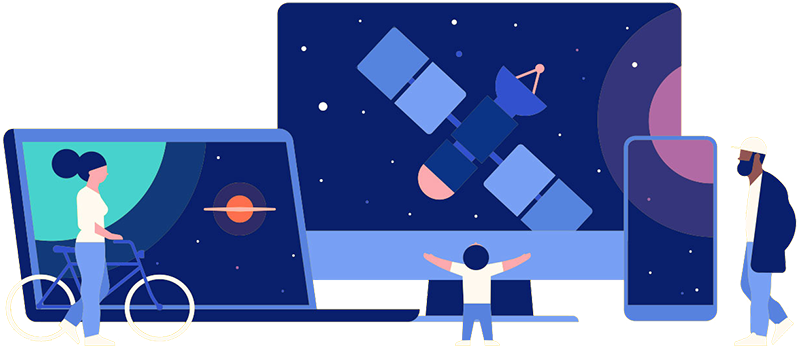
Visit Our
Live TV Access Guide
Online AccessPlatform & Device Access
Cable or Satellite Access
Over-The-Air Access
Visit Access Guide
Follow Us