It’s a pleasure to welcome you here. Thank you all for coming out. The Whitford Lecture is the Department of Astronomy’s distinguished lecture series, and it is named in honor of Albert Whitford, who was a longstanding member of our department and the director of the Washburn Observatory on campus. And this year, this semester, I’m pleased to introduce our lecturer, Professor Jacqueline van Gorkom from Columbia University. Jacqueline is a world-renowned radio astronomer. She’ll be telling us about some of her work tonight. She has been at Columbia for much of the past three decades. And so, without further ado, Jacqueline. – Thank you.
[applause] So I’m actually not going to talk about how to make a galaxy because I really don’t know how to make a galaxy. But I’m really going to talk about neutral hydrogen image of the universe. Now, why do I talk about that? That’s something in the ’80s. I was at Very Large Array in New Mexico, and I went around, I might even have come to Madison, giving colloquia saying, I want to make a neutral hydrogen image of the universe. Why not be ambitious? So why did I want to do that? So I just moved to New Mexico and there’s this Very Large Array and I was making images of neutral hydrogen around galaxies and so one thing we found was this picture here, that bright spot, that’s actually a very big galaxy just like our own. It’s really big. All the blue stuff around it, is neutral hydrogen. And so the image of the neutral hydrogen remains. And so I thought, well, wow, I thought it was a big galaxy, but actually there was much more than that.
It looks totally different when you look at neutral hydrogen. And so I thought, well, it would be really interesting to make an image of the whole universe in neutral hydrogen. And so the next three decades I’ve been trying to do that, and I’m actually just now beginning with Eric. But I’ll talk a bit about things that we found on the way there. So, first of all, what is this emission of neutral hydrogen? Neutral hydrogen is an atom. It’s the simplest atom there is. It’s an electron and proton. And normally the electron and the proton rotate in the same direction. And then about once every 10 million years, the electron decides to start spinning in a different direction, the opposite direction. Once every 10 million years.
So it doesn’t happy very often, but when it happens, it gets a little bit less energy so it emits a wave at 21 centimeter and it’s a radio wave. So you can actually observe neutral hydrogen, and since the universe is filled with it, it doesn’t matter that it happens once every 10 million years per atom because there’s how many atoms that you can observe it. So the Very Large Array is a radio telescope, and so I’ve been using that to make images of the neutral hydrogen. And I’ve had a lot of students over the years, and they make great pictures. At left you see a quilt made by my most recent students who made the field array in a quilt. And at right you see a nice sunset. So the Very Large Array is 27 dishes in the plains of St. Augustine in New Mexico. And so since you are observing at very low wavelengths, you need to have a very big dish to get enough angular resolution, right? If you want to see details, you need to have a huge dish. So instead what you do at radio, you say I don’t make one dish but I make many, many dishes and I combine the signals.
So that’s what we do at VLA. And, actually, not only that, we also use the fact that the Earth rotates. It does. So if you think about it looking from the sky, you see that the three arcs of the VLA trace out a circle in eight hours. And so if you combine all the signals, it’s like having a giant dish that gives you very good resolution. Not only that, you can actually also move out the antennae to different locations, and so you can combine data taken at different days, so you get different resolution. And so this is how you take out an antenna to a different location, it’s a circle transporter. It’s going on a rail on the plains of St. Augustine. You take up the antenna, it happens every four months, and then it gets transported to a different place. And I don’t know how many of you have been on Highway 60 going by the VLA.
It’s a beautiful spot, but if you drive by Highway 60, at some point you see a sign: “Watch out, crossing telescopes.” [laughter] And so that’s what happens. Okay, but so it’s a fantastic instrument, and I actually lived there for eight years. And so I actually lived close to the VLA, I was one of the few scientists. And this is telescope in Pie Town. And for a long time I thought I wanted to be the astronomer in Pie Town. There’s about 10 people there, but it’s very beautiful. And so when I was there, I also started– And so after I had been close to the VLA for a while I decided to move to Manhattan, so it’s a little different. [laughter] And so then I already started thinking about what does the environment do, in that case to people, but it also does something to galaxies, right? People in Datil, New Mexico, are actually very different from people in Manhattan. And for a while I went back and forth, and every time I made the transition, I had to sort of culturally adapt again.
In Datil, if you saw someone, you better stop and talk with them. In New York, you better not stop. [laughter] So it’s very, very different. And the other questions is: are galaxies different in different environments? So that is the first part of the talk. And then– So I’m first going to talk about how galaxies are nearby in different environments, and then I’ll talk a bit about how galaxies change if you look back in time. So that is what I’m working on now. So are galaxies different in different environments? So now I have to start telling you about environment, and so I’m going to tell you that in a cosmological setting. So what you see here is you see the results of a simulation. And you see four different times in the life of the universe.
Okay, this is very early in the universe, shortly after the Big Bang. So, on the top left, you see a picture that was very early in the universe. It looks very smooth. So what you see here is a simulation actually not of matter but of dark matter. So it is basically how structures go in the universe. You might not notice but most of the matter in the universe is dark matter. We don’t know what it is, but it is a lot of matter. And so this is the distribution very early in the universe. And if you go to the right, it’s somewhat later. And you see that you start forming these bright filaments and dark spots.
And if you look here, you see it’s very clear. So this is even later in the universe, and this is today, Sequence 0. That is what the universe looks like today. So you see these very bright filaments. That means that’s very high density. So lots of mass. And then in between you see these dark spots. And those are actually very empty spots. They’re called voids.
And so in the local universe, about 95% of space is really, really empty. It’s voids and they’re very interesting. So I’ll tell you a little bit about that too. But so these are the filaments. Most of the galaxies are in filaments, and when filaments connect, you might get clusters of galaxies where there’s thousands of galaxies very close together, and they’re gravitationally bound. So that’s, I’m actually first going to talk about clusters. On the lower right, you see now the distribution of the dark matter, and on the top, you see the distribution of light. And so if you look in different environments, it turns out galaxies look slightly different, which is pretty amazing. So notice if you look in groups or filaments, you see many spiral galaxies.
You see disc galaxies. Now, if you go to a higher density where these filaments connect, you get clusters of galaxies. So there are thousands of galaxies. And if you look very carefully, you see, especially at the left, that most of the galaxies are actually more elliptical shaped. They don’t have discs, they don’t have these spiral patterns. So in clusters of galaxies, you have many more early type galaxies, discs without star formation, or ellipticals than in groups where you have spirals. And so this is the nearest cluster of galaxies. It’s the Virgo cluster, and you see many, many of these early type galaxies. And so the question is: why is it that in different environments there are different kinds of galaxies? So here is a morphological sequence of what galaxies actually look like.
On the left, you have ellipticals. They are round. They have no star formation. On the right, you have the spiral galaxies. And so clusters are dominated by ellipticals and disc galaxies without star formation, without any gas. And everywhere else you see something. This is what we call the density morphology relation. Not very original. And so the question is: how do you get the density morphology relation? There are two extremes.
One is galaxies formed in different environments, and that is why they have different morphology. The other possibility is that galaxies are morphologically transformed by their environment. Right? And so you can go back to Datil and Manhattan. Are people born in New York different than people born in Datil, or do they just change every time they go back and forth? So I had a student who wrote a thesis on what can happen to galaxies in clusters. And so she had beautiful data, and then I said, well, you have to write one chapter where you describe all of this and which galaxies can actually get affected by the environment, the physical processes. So she disappeared for about a month, and then she came back with this. So she said, well, these are galaxies. So in yellow, you see the stars of galaxies. In blue, you see the interstellar medium of a galaxy. So galaxies, these galaxies have a lot of gas in the disc, and they form stars out of that gas.
In clusters between the galaxies, there is very hot gas. It’s like 10 million degrees, a hundred million degrees. It’s very, very hot. And so if you see purple, that’s the hot gas. So you see in the middle there a happy galaxy. What happens when this galaxy falls into the cluster? It can get ram pressure stripped which means that the hot gas pushes out the cold gas. So years ago, cold gas is flying out of galaxies and the hot gas pushes it especially out of the edges of the disc. So what you would see is that gas gets affected and the stars just stay the way they are. Okay, here is another possibility.
So these galaxies are surrounded by this hot gas. So it can, cold gas can evaporate. It’s very unpleasant for the galaxy. And, again, only the gas will get affected. But it can also gravitationally interact. So if two galaxies get close together, they can pull the stars out from each other. So, in that case, both the stars and the gas will get affected. So you get these very long tails. Probably doesn’t happen very often in clusters because galaxies move so fast with respect to each other.
So they don’t have much time to do this interacting. Okay, and then it can be the global potential of the cluster can also sort of truncate the galaxies. That’s called tidal truncation. It would also affect the stars and the gas. And then there’s something that’s currently really popular. It’s called starvation. So what that is, is the idea is that galaxies have this reservoir of gas around them, and that gas will, slowly over the life of a galaxy, continue to fall in and supply gas to the disc so that they can continue forming stars. Now, if a galaxy falls into a cluster, that reservoir might get removed. So then all of the sudden you have a galaxy without gas around it so it just uses up the fuel in the disc and that’s it.
And it doesn’t form any stars anymore. So that’s called starvation. And this is also very popular. That’s harassment. And so I told you that, so interactions are probably not that important, but people realize that galaxies, when they fly through a cluster, they get little pulls from all the other galaxies in the cluster. So the cumulative effect of all these little pulls will be that, again, the stars and the gas too get affected. So that’s called harassment. And so the question is: what do we see in clusters? And so this student actually made an image in neutral hydrogen of the nearest cluster of galaxies, which is the Virgo cluster. So that she said life is tough for galaxies. So this is, I still think this is an amazing picture.
It is not completely the real universe but it’s pretty close. So what you see is there’s a picture of gas. So I like gas. So in the center, you see this orange. That is the very hot gas in the center of the Virgo cluster. In the center you see M87. It’s a very big elliptical. And then you see around it all these blue discs. So these are disc galaxies that are falling in.
And to make them visible, we’ve blown them all up by a factor 10. So they are at the proper location, but the sizes are much larger than they are in reality. But what you should notice is that right in the center of the cluster, these discs are very, very tiny. In the outer parts, they are huge. Now, this is something that you expect from ram pressure stripping. If a galaxy falls into the cluster, the outer parts of the gas get removed. And so you see, I think this picture tells you everything that can happen to a galaxy in a cluster. But, here are some overlays. So what you now see is you see optical images of these galaxies and the contours are the neutral hydrogen gas.
So you see on top, you see some galaxies are really in the outer parts of the cluster. They have very extended neutral hydrogen. Nothing has happened to them. And the bottom row, you see galaxies that have only some gas left, right in the center, so that’s it’s completely gone out of the disc. That is why they are so smooth. So that is very, very interesting, and that is almost due to ram pressure stripping. And then there’s this galaxy, and you see 4522. That is one of the most interesting galaxies in the cluster. So there it looks as if the gas is being pushed out right now.
That it’s being pushed out of the disc. So that was, I’ll get back to that galaxy. But another thing that was very interesting in the cluster is if you look at a certain distance from the center, you look at these gas discs, you notice that all of them have tails in each one that point away from the center of the cluster. And so you see, on the sides, you see blowups of each of these galaxies. Again, you see the optical, and then in blue you see the neutral hydrogen. And what that shows is that, so in all these cases here you see that, for example, that the disc comes through but the gas is being pushed out. That galaxy is somewhere there. So it’s falling in. It’s being pushed out. And here the same thing.
The tail points away from the center. So we think that in this case this indicates that this is sort of the region where the galaxies are beginning to be affected by the cluster gas, which, again, we think is really cool. Okay, now this is this galaxy NC4522. It’s an amazing galaxy. So you see that the gas is currently being stripped out. Maybe I’m not going to say much, but one of the interesting things is so you can actually look at the optical stars in the discs and from the spectra we can estimate when star formation happened last in the disc. And so from this we know when the gas was removed from the outer disc. Which is a really important hint. And so why is that important? So we think it started forming stars about 100 million years ago, and the galaxy is right there.
To get it stripped, you would think it has to go through the center, but that would take about 700 million years. Well, actually, stars started forming about 100 million years ago, so we think, in this case, the galaxy got stripped right in the outer parts. I think we see that now very clearly happening. When a cluster forms, you have here the big cluster of galaxies and then you have smaller groups of galaxies falling into the cluster. And these groups also have a lot of hot gas. And so, actually you see it here, when a group falls in, it might stir up the hot gas in the cluster. And so that galaxy was just at a point where the hot gas from the different parts collided and affected the galaxy. And so we actually think that when you form a cluster, you actually affect the galaxies around the cluster very much, and that’s a really interesting thing to see happening. So this is of the density of the gas.
So this is a high-resolution movie of galaxies that are falling into the cluster, in the center of the cluster. And you can actually see that the gas is being stripped out and there are these long tails of gas that are left over. So we think we understand a lot now. We understand that galaxies that fall into clusters get stripped of their gas, and that is why there is no star formation in clusters. We have tons of data. We really believe it. And this is the outer parts where you see these things. So we know now that galaxies and clusters lose their gas because they come into high density regions. Now, we can also say what happens, I pointed out these voids, these very empty regions.
What happens there? And so there, actually, so these are very low-density regions. This is where the universe evolved very, very slowly. And so there we can see how galaxies grow. There’s the picture I showed you before. So there’s very large, empty regions. And so what do we know about galaxy growth? We a lot about the things we can see. We know how dark matter assembles and forms a large structure. But how you actually form a galaxy out of that, we don’t really know so well. And so people have been making a lot of simulations, especially in the last 10 years, and they sort of realized that galaxies might, so there’s two ways you can form galaxies.
Either you have a dark halo, another dark halo, they merge, gas falls in, you form a galaxy. Two galaxies merge and become bigger and bigger. It’s called hierarchical galaxy formation. But, more recently, people have realized that actually galaxies might mostly grow by slow infall of cold gas directly into the disc. And so they make very extensive predictions of how that happens. And one of the predictions is that at a current time, you should still see this in voids where there is small galaxies. And so I’m going to show you some results of that. So we’ve been looking at voids. You see the distribution of galaxies in the nearby universe as mapped out by the Sloan Digital Sky Survey.
So there’s a big survey in the local universe of all the galaxies. And so the bright orange is the destiny where all the galaxies are, and the dark spots is where you see in the nearby universe no galaxies, except for a few, and that’s these little diamonds that we have been pointing at. And so we’ve been looking at the gas in these galaxies in the largest underdensities of the voids. And here you see, so not many people have seen galaxies in voids. Here you are. You can see galaxies in voids. They’re tiny. You can’t tell that, but they are really tiny. The forming starts very actively. So these are galaxies that are just now beginning to grow. And here, this is also very interesting.
So this is now, you see the tiny galaxies on the left. That’s photographs. And then contours is the neutral hydrogen. So you have tiny galaxies in a void, but unlike in clusters, they have gas that just extends very, very far out. It’s amazing. And here you see this was the first galaxy we made an image of, and it is also the most interesting galaxy we found, which was quite interesting. So you see, on the left, you see this tiny disc of a galaxy. That galaxy is rotating like that. That’s the stars. Now the contours is neutral hydrogen. Giant envelope.
It’s rotating like this. So we call it the polar disc, for obvious reasons. So that basically we think is one of the best examples of a galaxy that is actually right now accreting this gas onto the galaxy. It’s very smooth. If it was merging, the optical disc wouldn’t look that undisturbed. And we found some filaments in voids, lots of them actually. Gaseous filaments, and in these filaments, probably, galaxies form. And this is a galaxy. I just have to show you this.
The local group, our galaxy is actually close to one of the largest voids that we know of. There’s a huge void very close to us, and there is also a little galaxy. On the left you see it. It’s tiny, has a few stars. Giant envelope of H1, and, again, the motion of the gas indicates that there’s something weird going on. So we think that’s another indication that there’s infall of gas. So the conclusion from this void survey is that by looking for it, you select a very interesting sample of galaxies. They might still be accreting gas, and they are very metal poor. So we think these are galaxies that have been very slow in forming.
So, again, the H1 tells us a lot. And this is a terrible picture, but I think it’s one of the most interesting pictures I’ve ever seen. So this is made in 2014 by some people in Australia. And this is the closest that you can come to making an H1 image of the universe. So this is basically a picture of the whole sky. And what you see in the contours is the density of galaxies. And in color, you see whether the galaxies are gas poor or gas rich. So red is very gas poor and blue is gas rich. And contours are galaxy densities.
So that basically tells you that everywhere where there are high densities, all of the sudden the galaxies are very, very gas poor. And if you go to low densities, they are very gas rich. I’ve just shown you that. You know this. But I think this is a fascinating image, and I just want to make a better image eventually of this. It’s great. Okay, so now, in the last sort of 15 minutes or so, I want to tell you about CHILES. That’s the thing I’ve been working on a lot recently, together with Eric. CHILES stands for the Cosmos H1 Large Extragalactic Survey.
And it’s a thousand-hour observation with the Very Large Array, it’s ridiculous. So we’re getting lots and lots of data. We’re working very hard. And this is to show you what astronomers do. They drink beer. [laughter] And these are all people, so there’s about 26 people in this collaboration, they are spread all over the world. And the reason why it’s important to talk about this is that radio astronomy is about to undergo a revolution, I think, because there’s all these new telescopes coming online around the world and so people from all these different telescopes are all in this collaboration. So we’re all talking all the time about the great things we’re going to do. So why do we want to do this? So this is basically a survey where we are making, so now I’m not looking at one cluster or a bunch of voids, but we are staring at one point on the sky, and we are integrating for a thousand hours.
So we get a very, very deep image. Right? A thousand hours. Normally you get six hours or something. This time you get a thousand. And why is this so special? So the Very Large Array, which came online in the 1980s, has been upgraded in the last seven years or so. So it used to be the best telescope in the world, and in 2010 it was still the best telescope in the world. But now it’s a factor 10 better in every possible respect because the electron is completely renewed. And so what is now possible is that we, in this one deep observation, we probe, in distance, out to about 4.6 billion years back in time. So we make a very deep observation, and we can see the motions of the galaxies over that whole range. So we make these images of neutral hydrogen over this tremendous range.
That’s what we are trying to do. It’s a big project. So we’re going back to what we see redshift of 0.45. It’s about 4.6 billion light years. And we are basically making pictures. We are looking what the kinematics look like, what the motions are, and just what the content is of galaxies with respect to this so-called cosmic rift, the distribution of the galaxies at large. So what we’re doing now about galaxy evolution going back over that time, we know a lot about star formation. If you look at the star formation density in the universe, we know that in the last almost 10 billion years the star formation rate dropped tremendously. So the universe is slowly forming less and less stars. And certainly this range that we are looking, it’s just going down very, very fast.
Well, what do we know about the gas in the galaxies over that range? Just about nothing. So that is what this picture shows. This is sort of summary of where we are right now in our knowledge of neutral hydrogen by just doing blind surveys. So this little area here, that is ALFALFA. It’s a fantastic survey with Arecibo. We know a lot about galaxies very nearby. Beyond that, we know almost nothing. So this is, again, the structure of the cosmic rifts as seen in galaxies, so you see these kind of voids. And so there’s a number of regions that have been probed.
I have to try this now. Okay, you see these two little things sticking out to the right? That’s a survey by [inaudible]. The thing that you’re seeing there, the very tall thing, that’s the CHILES survey. So that’s the range where we are going to find all these galaxies in H1. That’s our plan. And so we’ve been observing since 2013, and we’ll continue observing until 2019. So… this is just to show you that we can do it. This was a pilot. And here you see the pilot only did half the range that we are doing now.
And what you see is, so here you see the distribution of the galaxies at different distances. And so you see, again, you see these large structures. So you see walls here and there. And these are galaxies that we detected in H1, and so, again, you see that the morphology is really different in different environments. So this is very nearby, very extended in a very empty region. If you come to a wall, you see that galaxy get distorted. It has companions. And if you go to an even denser region, you see galaxies merging. So this, it shows you this was done in only 60 hours, and that already shows you that you get very interesting results if you do this. You really see the neutral hydrogen as a function of environment.
So I won’t bore you with that. But I do want to bore you with this because this is, I am actually very, very proud of this picture. So this is noise. So why do I get excited about noise? On top, you see the result of the pilot. So where we observed over this enormous frequency range, so obverse over 240 megahertz in one observation. That’s really special. Very high resolution. And you see that mostly the noise actually is flat. So that’s about the noise we should range, but then you see these peaks.
And what are they? They are due to satellites, cellphones, GPS, all the stuff you shouldn’t be using because it completely ruins our data. [laughter] Right? And so this is now the new data. So it is twice the range. This is the real survey. And it’s actually really interesting. So, first of all, you can see that this is 180 hours, we really go down in the noise. So we are still, we are doing well, by integrating longer, we get less noise. And you see that some of these peaks, they stay at exactly the same place so that the satellites keeping there. But some disappear and some appear.
It’s sort of fascinating. So it is not completely constant. In general, there is more satellites coming, which is really a big scandal, but that’s what’s happening. And another good thing is, so this is sort of real strange, if you go to higher, further distances, it actually gets better again. So we might get great results eventually. That’s what we are doing. So this is the result comparing the pilot to the first 180 hours. You see that if you integrate longer, the H1 grows. You observe more and more neutral hydrogen.
Now, this is interesting. I had a student working on this, the first phase of the series. And so she said, can we do something with the first 180 hours? So here you see the distribution of the optically long galaxies as function of, basically, distance, redshift. And you see there are these walls. Right? So there is this structure in the cosmic web. And so she said, well, let’s just have a look and see if you find something at a distance of a redshift 0.37. Nobody has ever made an H1 image at that distance. And so the first things we did was we compared in the galaxies in a wall at a redshift of 0.12 to galaxies at a redshift of 0.37. And here is the result. So that might not mean much to you but these are two spectra, and so what you see in the wall at 0.12, the mass in gas on average is about two times 10 to the nine.
At 0.37, going back in time, the mass is three times 10 to the nine. And we think that is a significant difference. This is just the thing that we wanted to see. That the gas content might actually being going up if you go back in time. And so we might have found that. So now this is one of our great discoveries so far. So that’s Ximena. That’s the student. And Hansung Gim.
He’s from UMASS. They did it together, basically. So, first of all, we detected a galaxy. And there it is. So you see a tiny galaxy. It’s actually not a tiny galaxy, a big galaxy, but it has a huge neutral hydrogen envelope. And so when we found that, we weren’t really sure whether we could believe it or not. So Hansung went to a telescope in Mexico to look for molecular gas. And he found that, actually even more amazing, there is a huge amount of molecular gas, more even than neutral hydrogen.
So this galaxy is very, very interesting. It’s one of the most gas rich galaxies we know about in the local universe. This is an optical picture, and it’s really asymmetric. It has a lot of star formation on one side. It’s forming stars very rapidly. And here you see, again, the overlay of that. So this is a complicated picture, but it shows you that on this axis you see stellar mass, on that axis you see H1 mass, and the little square is our galaxy and all these other points are other kinds of galaxies. What this tells you is that it’s rich in H1 but not uniquely so. But then, if you go to molecular gas, again the little square is our galaxy.
It actually is uniquely rich in molecular gas. It’s more gas rich than any galaxy nearby that we know. It’s pretty amazing. It’s really, really rich. And then there’s another thing that’s really pretty amazing, and that is the star formation rate in this galaxy. So what you see again, on the right, you see the stellar mass for different galaxies, and then, on the vertical axis, you see the star formation rate. And so different kinds of galaxies have been plotted here for different parts in the universe. So here you see galaxies that are really nearby, redshifts of 0.2 and 0.5. And then the bright symbols to red are between 0.5 and 1.
And our galaxy is that big start on top there. So it’s forming stars way too rapidly for its mass. Way too rapidly. It’s uniquely unique. It doesn’t exist in the local universe. And then, if you compare it to galaxies that have redshifts between 1.5 and 2, it actually fits in very, very nicely. So it looks like there’s one of these very young galaxies in the very early universe that is forming stars like crazy, maybe because gas is falling in, that we are seeing here at this redshift. So that’s our big discovery. We are very happy about that.
So that’s the first discovery in this big, big survey. So eventually this will become very, very interesting, and so you should just ask Eric to give a talk about this in a little while. [laughter] To present all the results. It’s the first step. And so why is this so important? It is because all these other telescopes are being constructed. There’s MeerKAT in the Karoo Desert in South Africa. It’s about to start observing. There’s a telescope in western Australia, ASKAP. There’s a telescope in the Netherlands being built, and then, eventually, there will be the SKA, Square Kilometer Array, you might have heard of that, that will also be built in South Africa. So these will all get amazing results.
They will find many, many more galaxies. And you will hear a lot more about each one. So I want to finish with, since all these places are so interesting, I went to the Karoo Desert to look at this telescope there. And I thought it looked very much like New Mexico. It actually really does. If you drive through Karoo, you think just like New Mexico, but then we saw this, and I said, what is this? What is this? What is this? So… what is this? It’s a nest of sociable Weaver river birds. So these birds, they get together. They start out with a small nest, and then more and more birds come and they build a bigger and bigger nest, it’s like bees, sort of beehive. So there’s hundreds of birds making this nest.
So that by itself is a good reason to go into radio astronomy, I think. [laughter] That’s it. Okay, thank you. [applause]
Search University Place Episodes
Related Stories from PBS Wisconsin's Blog

Donate to sign up. Activate and sign in to Passport. It's that easy to help PBS Wisconsin serve your community through media that educates, inspires, and entertains.
Make your membership gift today
Only for new users: Activate Passport using your code or email address
Already a member?
Look up my account
Need some help? Go to FAQ or visit PBS Passport Help
Need help accessing PBS Wisconsin anywhere?
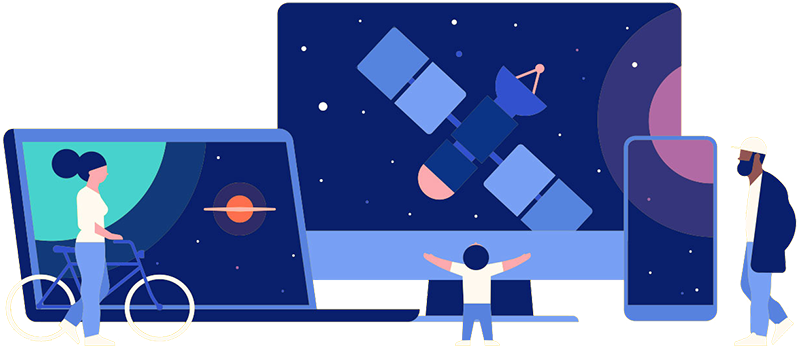
Online Access | Platform & Device Access | Cable or Satellite Access | Over-The-Air Access
Visit Access Guide
Need help accessing PBS Wisconsin anywhere?
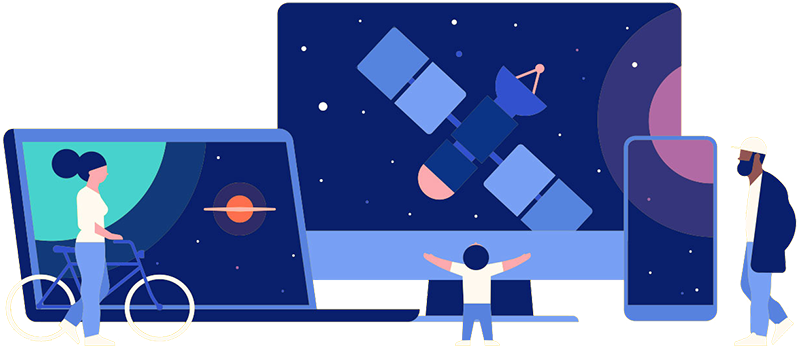
Visit Our
Live TV Access Guide
Online AccessPlatform & Device Access
Cable or Satellite Access
Over-The-Air Access
Visit Access Guide
Follow Us