[Lori Berget, 4-H Youth Development Educator, Lafayette County, University of Wisconsin-Extension]
Good afternoon, I’d like to introduce Dr. Tom Fitz, Associate Professor of Geoscience at Northland College in Ashland, Wisconsin, who delights in the Earth’s story and sharing that story and why it matters in all of our lives.
Dr. Fitz is a dedicated professor for over 20 years and has received many awards for his teaching and research while at Northland College. When asked what drew him to Wisconsin from Massachusetts, he replied, To teach and study rocks. Wisconsin is a wonderful geologic setting to learn and teach students. Living near the Penokee Range has enabled Dr. Fitz to study the unique setting that makes up the range for the past 16 years. He loves to take students on field trips and share the natural beauty of the area. At this time, it gives me great pleasure to welcome Dr. Tom Fitz to present Geology of the Penokee Range in Wisconsin.
[applause]
[Tom Fitz, Associate Professor, Department of Geoscience, Northland College]
Thank you, Lori.
[Lori Berget]
Thank you.
[Tom Fitz, on-camera]
All right, welcome, thank you for coming. It’s a pleasure to share the fascinating story of the geology of the Penokee Range, yes. Okay, my career has really been dedicated to teaching people about the Earth and their relationship to it and also why that matters. Why should we care about the geology of a place, okay?
[slide featuring a photo of Professor Fitz and four students outside crouched over and looking at a rock formation]
And I’ve been leading geology field trips through the Penokees for about 15 years, and it never ceases to amaze me what I see there in the rocks. So, this is what we’re gonna do today –
[new slide titled – Outline]
– okay. I’m gonna take you to the Penokees the –
[the slide animates on the words – The Penokees]
– best I can. And so, first I’m gonna tell you just a little bit about the geography and the general geology of the Penokees.
[the slide animates on the words – Geologic History]
Then we’ll look at the rocks, and we’ll travel back in time billions of years and read the story in the rocks and figure out how those rocks have formed.
[the slide animates on the phrase – Why the geology matters]
And then we’ll spend some time thinking about why that matters. Why does the geology of the Penokees matter –
[under the phrase – Why the geology matters – the word – Resources animates in]
– and the geology of any place? This is – this is why geology matters –
[Tom Fitz, on-camera]
– to society.
One is the resources, okay. Minerals, energy, and water. Those are the geological resources that we all need, that we all rely on, and are used in large quantities by society. Okay, we’ll also talk about hazards. The environmental hazards associated with resource use and also the human health hazards because we live on a crazy, dynamic planet, and it’s got a lot of hazards, and we can – we need to understand those so we can protect ourselves, okay? And then also just the, how it enriches our lives to understand our surroundings. And that’s definitely true, and that’s true of the – the geological stories under our feet as well. So, we’ll – well finish with that.
Okay. So, first just a little bit about the Penokees. Okay, the Penokee Range, this is a landscape map –
[slide featuring a relief map of far northern Wisconsin, which includes the peninsula of northern Wisconsin that juts out into Lake Superior and includes the Apostle Islands and Bayfield County and makes a U shape east into Iron County]
– of northern Wisconsin. And the Penokee Range is over here [indicating the far right of the U shape which presents as parallel ridges in north central Iron County] alright. And it is basically these double ridges [indicating the parallel ridges on the map with the mouse pointer] right along there like that. It is – it stands about 400 feet – the two ridges stand about 400 feet above the surrounding landscape. It’s about 50 miles long, and then it also extends over into Michigan and goes about 50 miles into Michigan where it’s called the Gogebic Range. So, together we call it the Gogebic/Penokee Range, okay?
[Tom Fitz, on-camera]
And its really – it is there because of the hard bedrock underneath, okay? The area is sparsely populated. It’s really quite beautiful. It has – it receives about 30 – 33 inches of rain per year, and the average –
[slide featuring a landscape photo with the Penokee range in the distance]
– annual temperature is about 43 degrees, important – important numbers when we’re considering what will grow there, alright? What is – what is the ecosystem of the area?
It is mantled by a thin veneer of soil, mostly loamy soils derived from the till –
[Tom Fitz, on-camera]
– the glacial till that is underneath it. And that together, the soils and the water, support a pretty dense forest, and it’s – its quite a pristine area, okay? I should mention, this photograph, which was taken from Corrigan’s Bluff –
[return to the previous slide described above]
– sort of in the middle of the Penokees, was taken by my friend Megan, who’s a great photographer. And I have – I give credit to people whose photographs I have borrowed or diagrams I have borrow – borrowed in the lower right of each slide. And the rest of them, the cartoons and the – the photos that aren’t so good, the rest are mine, okay?
[new slide featuring a geological map of the previous relief map showing the different geological layers]
So, as I mentioned, the Penokee Range really owes its existence to the hard bedrock. And this is a geologic map, a bedrock geologic map, of the area, and you can see the different layers. And, basically, the Penokee – in the Penokee Range, there are layers and layers of rocks that are stacked up and that have been tipped to the north. So, basically, what we see on the stripes, those are the edges of rock, bedrock units, alright? And there are two layers in there that are especially hard that stand up. And so, that’s really what this story is about. It’s taking a look at – at those, the rocks within those two edges –
[new slide titled – Geologic Cross Section of the Penokee Range – featuring an illustration of what looks like two mountain peaks in the center with two parallel lines that start from the left base of each peak and flow down to the bottom of the slide]
– okay?
So, this is a geologic cross-section. Okay, if we go back –
[return to the previous slide with the layers described above]
– if we were to draw a geologic cross-section across the Penokee Range, going like that, from north to south, and take a slice of the Earth, that’s what we’d be looking at. From south on the left to north on the right, okay? So, this is my cartoon drawing of it. And what we can see down here is this is the bedrock underneath. Okay, the hard bedrock.
[the word – Bedrock – animates in-between the center of the two parallel lines]
And we can see the two ridges there, okay? So,there are two layers within the rock, within the bedrock, that’s especially hard, the southern ridge and then the northern ridge here. [using the mouse pointer to indicate the two peaks] alright? And then overlying that –
[the slide animates in a line that starts on the left and goes over the two peaks to the right side of the illustration and then labels the areas south of this line between the peaks as – Surficial deposits]
– are the surficial deposits that are of glacial age, and we’ll mention those as well. That mantles the hard bedrock ridges, and as I mentioned the soils have actually formed in that glacial material, those glacial deposits, okay? So, what we’re gonna do is we’re gonna take a look at the story within the bedrock going from this oldest stuff. [indicating with mouse the area labelled – Bedrock] There are basically three packages of bedrock within the – within the ridges here, okay? On the south [indicating with the mouse pointer the area of bedrock on the illustration that is to the left of the center area labelled – Bedrock], that’s the oldest one, the middle one, and then the northernmost one, [moving mouse pointer from left to right] alright? And we’re gonna travel back in time and look at how those –
[new slide featuring a photo of a section of bedrock that is jutting out on the landscape in the Penokee range]
– things have formed.
Okay, so there it is. There’s some – theres some hard bedrock sticking out. And the – around it is soil, the – the glacial deposits around it, okay? So, that’s some hard basalt sticking up. Basalt volcanic rock sticking up in the woods in the Penokees.
[new slide featuring an illustration of geological time as a circular ramp that starts at the present and swirls down to the beginnings of the Earth]
Okay. So, before we get going on the details of the story, one of the things I have to mention is that –
[Tom Fitz, on-camera]
– when we’re talking about geologic stories, we have to extend our thinking of time into deep time, okay? And that’s really one of the really fun things, and I think important things, about understanding the geology and what geology brings to us, you know, as a science, any historical sciences, to stretch our minds outside of the human time frame, alright? And that’s an important thing to do, I think, to be able to see – see processes, natural processes, on very long-time scales, alright?
So, what we’re gonna do is we’re gonna travel way back in time, way back down that –
[return to the previous slide described above]
– spiral of geologic time to about halfway back to the beginning of Earth’s history, okay, to about 2 and a half billion years ago, and then move our way forward, okay?
[new slide titled – Millions of Years Ago – and featuring a strip-like vertical table of seven time periods with a y-axis of millions of years. It starts with 4600 to 3800 and an area called, Hadean; next is 3800 to 2500 called, Archean; next is 2500 to 542 called, Proterozoic; next is 542 to 251 called, Paleozoic, next is 251 to 65 called, Mesozoic; next is 65 to 2 called, Cenozoic; and last is 2 to 0 called, Pleistocene]
So, this is a geologic – geologic time scale not drawn to scale. But the olds – the beginning of the Earth, 4.6 billion years ago to today up here, alright? It’s not drawn to – drawn to scale, but the major geologic time periods are shown here, alright?
[Tom Fitz, on-camera]
And basically, the bedrock is – was created in three episodes, okay, spanning many hundreds of millions of years each, but three episodes of rock-forming processes are represented in the Penokees, okay? From 2.7 billion years –
[return to the previous slide with the geological ages table and now with three arrows pointing at where the Penokees were formed – the bottom arrow at the top of the Archean age (2700 million years ago), the middle arrow at the bottom of the Proterozoic age (around 2000 million years ago), and the top arrow about halfway through the Proterozoic age (1100 million years ago)]
– ago to 1.1 billion years ago. And that’s the – thats the old story that we’re gonna look at in the bedrock.
[a new arrow animates on at the top of the geological table pointing towards the Pleistocene (or 2 million years ago to present)]
And then there’s the more recent story, relatively recent in geologic terms, of the glacial story and the glaciation of that and the deposition of the glacial deposits and the development of the soils that support –
[new illustration titled – 2.7 billion years ago – featuring a cross-section of the ocean at that time – with sky at the top of the slide, waves representing the ocean in the middle of the slide, and a gray area representing the mantle of the earth which in this case has a plume of magma shooting up through the center and overflowing on the top of the mantle]
– the forests today.
Okay. So, let’s go back to 2.7 billion years ago, okay? And one of the really interesting things about the Penokees is that –
[Tom Fitz, on-camera]
– since it was tipped up like that, it is such a complete, it’s a really remarkable story there. I mean, these are some of the oldest rocks in Wisconsin right here. And 2.7 billion years ago, it was a time when the continents were just first starting to develop.
So, when we’re thinking about this far back in time, we can’t think about it as like the Penokees are today, right? We have to think about it almost like this is taking place on Venus or something, you know, and it evolves through all this – these billions of years of constant geologic change to be what it is today, okay?
So, 2.7 billion years ago, very few continents, and the rocks that were – were created and preserved there were created on the ocean floor in – from submarine volcanoes, okay? And this is actually the oldest, some of the oldest rock to be preserved, alright? So, there was certainly rock there before this, but the rock cycle was moving so fast, rocks were being constantly recycled, and so these are the oldest things to get preserved, okay, some of the oldest stuff in Wisconsin, alright? Yeah, a remarkable story.
Okay, there it is. These –
[slide titled – Gneiss – featuring a photo of gneiss in the Penokee range that features a multi-shaded rock formation of whites, and other shades of gray indicative of the many types of rock of which it is composed]
– these rocks are all scrambled in together. They’ve had a complex history long ago. They got very hot. They got deformed like Silly Putty. And the rock is a gneiss, okay, G-N-E-I-S-S. Complicated stuff, some really beautiful stuff there, okay?
[new slide titled – Erosion – featuring an illustration of a rock bed on the lower quarter of the illustration and the word – Erosion – above in with an arrow pointing to the right]
And then what happened after a long complex history recorded in that rock itself is a long time of erosion, alright, and much of it was stripped away. So, we lost quite a bit of record there. So, it was a long period, like hundreds of millions of years of erosion, and –
[the slide animates on an area of waves above the illustrated rock bed that represents the rising of the ocean]
– then sea level rose. And sea level rises and falls a lot, okay –
[Tom Fitz, on-camera]
– when we’re talking about these long periods of geologic time. So, sea level will rise across the continents and deposit marine rocks across all the continents, and it’ll go back down. Much of that will be eroded, and then it will rise back up again. And that has happened many times in this region, and most regions of most continents have marine sedimentary rocks preserved on them.
Okay, so sea level rises. This is going back to about 1.9 billion years ago. And sediment is deposited upon that surface, okay?
[return to the previous illustration now with a new layer above the rock bed between the rock bed and the ocean]
So, those old volcanic rocks are eroded down to a flat surface. This stuff –
[the slide animates on the word – Unconformity – that has an arrow pointing to the new layer of sediment above the rock bed]
– was deposited on top of it, and we call that gap in time right there, we call that an unconformity, alright? So, across this gap [indicating the top and the bottom of the Unconformity with the mouse pointer] there is, you know, 700 million years of time missing, alright, right there.
Okay. And deposited on that, above –
[new slide titled – Slate and Quartzite – featuring a photo of a rock formation that is made of layers of slate and quartzite]
– the unconformity, was lots of layers of sand and mud, okay, in this shallow, off-shore basin. Layers and layers of sand and mud that later were compressed and heated and turned into slate and quartzite, alright?
[return to the previous illustration with the cross-section of the ocean with the unconformity layer above the bed rock, but now with a new layer above the unconformity between the unconformity and the ocean]
And then the deposition continued where another layer laid down, but this is a little bit different. This took place, the deposition of the next layer up called the Tyler, no, called the ironwood formation, okay?
[Tom Fitz, on-camera]
The ironwood formation was deposited at a time when there was a lot of change taking place in Earth’s atmosphere. The first photosynthesis on a large scale was putting oxygen into the atmosphere in high enough abundance, still low quantities, but high enough abundance that the chemistry of the atmosphere was changing, and some of that oxygen was dissolving into the oceans. The oceans at that time were quite rich in iron, okay? So, we had oxygen, the first free oxygen in the atmosphere –
[return to the previous slide with cross-section of the ocean now with the word – Oxygen – above the illustrated waves that represent the ocean]
– in what we call the great oxygenation event, alright, which was a really big change on Earth, okay?
[the slide animates on an arrow from the Oxygen above the waves to the cross-section representing the ocean indicating the oxygenation of the ocean]
Oxygen dissolved in the water.
[the slide animates on the word – Iron – in the ocean area of the cross-section indicating the ocean was rich in iron]
The ocean was richer in ox – iron at that time. And –
[the slide animates in a new name for the layer between the ocean and the uncertainly that is now labelled, Iron oxide]
– the oxygen and the iron combined and were deposited on the ocean floor as big layers of iron oxide, alright? And that – that time period, about 1.9, 1.8 billion years ago, is –
[Tom Fitz, on-camera]
– when all of the big iron formations on Earth were deposited because of this – this – this chemical reaction that was taking place because of the – just the correct geochemical situation.
And it created these, the big, beautiful, banded iron formations, okay? And that’s it right there.
[new slide titled – Banded Iron Formation – featuring a photo of an outcropping of rock that has bands of iron oxide in it]
That’s the ironwood iron formation. It has layers of iron oxide in here. In this case, it’s the – its the mineral magnetite and layers of quartz, like that, okay?
The ironwood iron formation is about 700 feet thick, and it’s mostly like this. Some places it’s more quartz; some places it’s more magnetite. But –
[new slide featuring a new photo of an outcropping of rock that has iron oxide banding in it]
– it’s really a remarkable rock. I mean, there is, [laughs] it’s crazy, alright, because of – of all of the magnetism –
[Tom Fitz, on-camera]
– for one thing, okay? It’s also a really beautiful rock. I mean, it’s like the stripes. It’s like tiger-striped in red and white and black in some places, really remarkable stuff. And you can see right there, that’s my magnet.
[return to the slide with the cross-section of the ocean described above]
You can see the – whoops, I didn’t mean to do that.
[new slide titled – Breccia – featuring a photo of an outcropping of rock that has the iron oxide banding]
[return to the previous slide of an outcropping of rock that has iron oxide in it now with Tom pointing out his magnet that is stuck to the side of the rock]
Okay, you can see my magnet stuck right there on the – on the – on the rock, okay? There aren’t many places in the world where you can get it – go up to a rock and throw a magnet and it sticks, alright? And the Penokee Range is one of those places, one of the few places on Earth.
[Tom Fitz, on-camera]
It’s also one of the few places on Earth where your compass doesn’t necessarily point north, alright, which can make navigation in those woods very challenging, you know, because you don’t know whether you can – well, sometimes you don’t know whether you can trust your compass cause it’s not doing the right thing. Sometimes it’s pointing east/west along the length of the range, and sometimes it doesn’t spin at all. It’s like it pegged down, it wants to be on the rock, and the compass needle doesn’t change at all. So, anyway, just – just a really remarkable rock, okay?
Now [laughs], this is a fairly recent discovery. At the top of the iron – ironwood iron formation, there is a very interesting layer of broken rock, alright? So, I’ve represented it there by that –
[return to the slide with the illustrated cross-section of the ocean with the rock bed on the bottom, the Uncertainty above it, and the layer of iron oxide above that, now with a dotted red line above the iron oxide layer between the iron oxide and the ocean]
– dotted red line, alright?
[return to the slide titled – Breccia – featuring a photo of a layer of rock that is made of other broken up rocks still with some of the iron oxide banding]
And there it is, this – this one isn’t actually in the Penokees because this layer, it’s about 10 feet thick, this crazy, broken rock that we call breccia. It sits on top of all of the rocks, all of the iron formations in the Lake Superior region. It actually isn’t –
[Tom Fitz, on-camera]
– exposed in any outcrops in the Penokees, but we know it’s in there because there have been so many drill holes penetrated through that, so we know this rock exists.
This particular outcrop in this photograph, I took this photograph in Thunder Bay, Ontario –
[return to the previous slide titled Breccia described above]
– in the same stratigraphic position. So, a similar thing exists in the Penokee Range, Thunder Bay, Ontario, okay? And you can see that that rock is just broken. I mean, it’s just made of broken pieces. And it wasn’t really understood what this breccia, what formed this breccia, all these broken pieces? They’re all sedimentary rocks. You can see the layering in them, but they’re all spread around like that.
Well, interesting story is –
[new slide featuring a close-up photo of the Breccia showing some spherical pieces in amongst the broken rocks]
– that – oh, and then it also has, some places it has the broken pieces, but then it also has these spherules and some perfect spherical spherules in there. Very interesting stuff, alright?
And the discovery came in 2005 by –
[Tom Fitz, on-camera]
– Bill Addison, who was a science teacher in Thunder Bay, Ontario, and one of the outcrops was right up the road from his house, and he’d been studying that for a while. He had a microscope in his basement, and he found some of these, okay?
[slide featuring six microscopic photos of the spherules showing quartz grains with planar fractures]
And these are quartz grains, so these ones right here. That, that, and that, those three [indicating the top three photos with his mouse pointer] those are quartz grains, okay, and they have planar fractures in them, and quartz doesn’t have planar fractures in it, alright? Quartz does not have any – anything what we call cleavage, which is a weakness in the crystal structure. It doesn’t have that. There’s only one way that quartz can have a fracture, a planar fracture like that –
[Tom Fitz, on-camera]
– and that is if it gets hit really hard, alright; harder than anything on Earth. Actually, it has to get hit by a meteorite, okay?
So, this is the story and this evolved. That was 2005 that Bill made that discovery, and the story has been sort of evolving since then, okay? And the United States Geological Survey has done – done some really interesting studies on this as – as well. And it’s now been tied to the impact crater that was known for a long time in Sudbury, Ontario, okay-
[slide featuring an artists rendition of a large meteor hitting the Earth and causing a huge explosion]
– which is the third biggest known impact crater on Earth, alright? And what we see in this breccia layer at the top of the ironwood iron formation represents an absolutely catastrophic meteorite impact, okay? A rock about six miles in diameter slammed into the Earth, and, basically, what we see –
[return to the previous slide showing the breccia and spherules close-up]
– in this rock, okay, those are pieces of the ocean floor that were torn up by a great big tsunami, alright? It’s estimated that that tsunami may have been about a kilometer tall, okay?
[Tom Fitz, on-camera]
So, 0.6-mile-tall wave that went around the Earth, alright, and tore up the ocean floor. This was basically a global event, alright? And the spherules here, the round, the funny, round spherule pieces in there –
[return to the previous slide described above]
– those are pieces of melted Sudbury, Ontario, okay? [laughs] Sudbury, Ontario was vaporized, what is now Sudbury, Ontario, and the rock went into the atmosphere and then condensed into these –
[Tom Fitz, on-camera]
– lapilli we call them. And these lapilli came down, raining down into the ocean floor after it’d been all torn up by this – this meteorite impact, alright? [laughs] That’s a crazy story, okay.
And that layer, okay, exists at the top of all of the iron – all of the iron formations in the Lake Superior region. Okay, so that layer has now been found in about –
[slide titled – Sudbury impact debris deposits – featuring a map of the Midwest region of the United States and Canada, showing a large gray circle in Sudbury, Ontario showing the approximate radius of the meteor that hit there (160miles) and then concentric circles radiating out from the impact site. Additionally, there are 10 red dots located on the south, southwest, and northwest of Lake Superior showing areas where geologists have discovered Sudbury impact debris]
– 10 places around the Lake Superior basin. There’s the Sudbury impact crater right there, okay? [indicating the gray circle with his mouse pointer] So, this – this is a remarkable story that this layer is actually preserved, okay? The other interesting thing about this is that it’s at the top of all of the iron formations –
[Tom Fitz, on-camera]
– and it ended iron formation deposition, at least in this part of the world, okay? So, this was a global event. It was such a big event that it changed the chemistry of the oceans in such a way that iron formations were no longer deposited on this big scale. There were small ones later on, but this changed the world, this meteorite impact, okay? And the United States Geological Survey is continuing studies on that with drill core from the Penokees, okay?
So, that layer is very nicely dated. We have a nice date from the Sudbury layer at 1.85 –
[return to the illustrated cross-section of the ocean slide with the rock bed at the bottom now labelled as 2.7 billion years ago, the unconformity layer above that, the iron oxide layer above that, and the dashed red line above the iron oxide layer now labelled as 1.85 billion years ago]
– billion years ago, okay? So, we’ve got the 2.7-billion-year-old package, and we’ve got the 1.85-billion-year-old –
[the slide animates in a new layer above the dashed red line of the meteor impact and below the bottom of the blue waves that represent the ocean]
– package. And then there was more rock deposited on top of that layer, and these rocks were deeply buried and heated up enough that they will change a little bit in a process we call metamorphism. So, there – there was some recrystallization –
[Tom Fitz, on-camera]
– in an event called the Penokee orogeny, okay, at about 1,830 million, 1.83 million years ago, okay?
And then, another period of erosion, okay? [laughs] So, we lost some of that geologic record. And then the next event that created rock was another few hundred million years later, okay? Oh, so we have the development –
[return the previous slide described above with the top of the new layer having an arrow pointing at it which is again labelled – Unconformity]
– of another unconformity there, alright? And then a big gap in time, many hundreds of millions of years go by –
[return to the slide with the table of geological ages on it described above]
– and then the next event –
[the slide animates in three arrows to the right of the table, the bottom one points at the top of the Archean era, and the middle and top arrows point at the bottom and middle (respectively) of the Proterozoic era]
– is the third in our formation of bedrock units here, [uses his mouse pointer to indicate the topmost arrow], alright –
[new slide showing the illustrated cross-section of the ocean floor now with a shoot of magma coming up from below the ocean floor and exploding on the top of the ocean floor above what was labelled the second unconformity. Underneath the flowing magma is now a layer of volcanic soil above the unconformity layer]
– and that is huge eruptions, alright? And this is what we call the Midcontinent Rift. So, North America, the – the small North American continent that was growing at the time actually started to split open in –
[Tom Fitz, on-camera]
– this great big split of volcanoes, alright, that we call the Midcontinent Rift. And it was estimated – it was about a 20-million-year period or so of lots of volcanic eruptions. It was estimated that three million cubic kilometers of magma was produced along the Midcontinent Rift, okay? That’s a lot of magma, alright? At times, the lava flows spread out across the Earth and basically repaved the Earth’s surface with black lava, the – the rock that became the rock basalt, okay? And this is well-preserved in the western Lake Superior region in Wisconsin and Michigan and Minnesota, okay?
So, lots and lots, miles and miles thickness of basalt lava flows. You can see that –
[slide featuring a photo of Basalt ropes – labelled Basalt in the photo – on the upper half of the photo with a more reddish clay at the bottom of the photo]
– is actually those ropes right there. We call those pahoehoe ropes, beautiful Hawaiian name for these ropey lava flow tops, okay? So, that’s a lava flow, and then this is the base of the next lava flow up, so lava flows piled up and piled up, miles thick.
[return to the previous cross-section of the ocean floor illustration with the previous red magma flows on the illustration now turned to black to indicate the magmas change into the rock Basalt]
[Tom Fitz, on-camera]
And that event is also very nicely dated, and then it crystallized to make the basalt. This took place 1.1 billion years ago, alright, the Midcontinent Rift.
[return to the previous slide with the illustrated cross-section with the basalt layer now dated at 1.1 billion years ago and the layer below the Midcontinental Rift now labelled as 1.85 billion years ago]
So, then we’ve got the 1.85 and –
[the slide animates on the words – 2.7 billion years ago – on the bottom layer of bedrock on the cross-section illustration]
– the 2.7 billion year – those are the three, basically, the three packages of rocks –
[new slide titled – Conglomerate – featuring a photo of rocks in the area of the Penokee Range that are from all three types of rocks that are found in the range]
– within the Penokee Range, okay? But the Mid – when the Midcontinent Rift, when the volcanoes died down, then what happened is the volcanoes were eroded, and they were basically buried in their own debris, alright?
[Tom Fitz, on-camera]
So, we have gravel, and there – there’s the, what’s called the Copper Harbor Conglomerate is a layer that sits right above the lava flows, and you can see the – the –
[return to the previous slide titled – Conglomerate – described above]
– gravel in there. This is a really very photogenic rock as well because it’s like rock within rock, right, conglomerate. So, you get these rounded pieces, and those are all volcanic pieces, which is interesting. So, clearly, it had to be deposited on the side of a volcano because all of the pieces are volcanic. Yeah, and you know what? When that rock is now getting eroded, those pieces will pop out, right, and now it’s a pebble with the shape –
[Tom Fitz, on-camera]
– that it had attained a billion years ago, which is kind of an interesting thing to inherit, okay?
And then there was – the conglomerates were buried in sandstone, and these are the great big sheets of sandstone that exist in the Bayfield Peninsula and the Apostle Islands –
[slide titled – Sandstone – featuring a photo of the sandstone cliffs around Bayfield in the wintertime]
– if you’ve been up there. And the – the sea caves have – the ice caves have rec-recently become discovered and become very popular. And those are in the sandstone. [clears throat] That’s Lake Superior, [clears throat] excuse me, frozen Lake Superior. And those are the sandstones that sit above the Midcontinent Rift rocks.
[return to the illustrated cross-section slide with the layers of bedrock, iron oxide, and basalt with the dates 2.7, 1.85, and 1.1 billion years ago]
Okay, so there it is, there’s the sandstone [uses mouse pointer to indicate the layer above the basalt in the cross-section], and that’s our package of the rocks, okay? The resistant layers, the part that’s gonna be important here, is that the resistant layers are the ironwood iron formation and then those lava flows, alright? So, in recent time, when we bring this to the recent, those are gonna end up being –
[the slide animates to be at a 45-degree angle so that the layers more closely represent the actual layout of the Penokee Ridge]
– the Penokee Ridge, okay?
So then, right after the Midcontinent Rift, the whole area got compressed in a great big tectonic vice as continents ran into North America and closed that rift, and the whole thing got tipped and folded into a great big U shape like this, and basically the U shape is Wisconsin on the south side and Minnesota on the north side. So, the western Lake –
[Tom Fitz, on-camera]
– Superior region is basically a canoe, right? It’s like the gunnels of the canoe are the hard edges of Wisconsin and Minnesota like that, alright? So, this is the southern edge of that canoe. Okay.
Those are the bedrock units, and then we have an even bigger gap in time when there is a long period of erosion, oaky?
[return to the table of geological times by millions of years now with a new yellow arrow pointing at the Pleistocene era at the top of the table]
Probably there were, sea level rose and deposited rock upon it, but it has also been, those have also been eroded away. So, we skip to relatively recent geologic time –
[slide featuring an illustration of the two ridges of the Penokee Range and their corresponding geological make up. As Tom described above they make a U-shape with the older 2.7 billion-year-old bedrock on the left-hand side, the 1.85 billion-year-old ironwood formation next on the first ridge and the 1.1 billion-year-old basalt layer next on the second ridge]
– and this is what it looked like probably a few million years ago, not billion, a few million years ago when the climate started to change, and ice advanced out of the north –
[the slide animates on an ice layer on the top left of the slide covering the second ridge and a part of the first ridge]
– and overrode the Penokee Range –
[the slide animates the ice layer to cover the entirety of the Penokee Range]
– and scraped off any soft rock off of the top, alright? And then, when it melted back –
[the slide animates off the ice layer and adds in a layer of sedimentary rocks on the top layer of the Penokee Range]
– left a mantle draped over there of rock, I mean, of sediment, okay? All kinds of glacial deposits laid in there, on top of it, a thin – relatively thin veneer, some places a hundred – a few hundred feet thick. But on the Penokee Ridge itself, the two ridges, this – this right here [indicating the first peak with his mouse pointer] is the ironwood ridge, the hard ironwood ridge, and that [indicating the second peak with his mouse pointer] is the basalt ridge of the Midcontinent Rift. So, those are the two hard ridges, and the glacial deposits are relatively thin up there, thin soils. There’s a lot of rock exposed right along the top of those two ridges.
[new slide titled – Glacial Till – featuring a photo taken in the Penokee Range of glacial till made up of a hodge-podge of sediment left by the retreating glaciers]
Okay, so there’s the glacial – glacial till. That’s the stuff that is just dumped by a glacier when it melts, alright? So, there – there’s a mantle of till, and then at the very top, you can see the soils that have developed on top of that.
[new slide featuring a photo of a hummocky topographic landscape near the Penokee Range]
There’s one more, okay, and there it is, the hummocky topography of a classic [clears throat] glacial – glacial deposits that surround the Penokee Ridge.
[new slide titled – 9500 years ago – featuring the same illustration of the Penokee Range and its geological U-shaped make up now with a lake of water just to the right of the second ridge]
Okay, one more event, and that was as the glaciers were melting out of this – out of the Penokee region for the last time, there was a large lake there. [laughs] That was in the western Lake Superior basin. We call it Glacial Lake Duluth, right –
[Tom Fitz, on-camera]
– and the water level was about 500 feet above the current Lake Superior water level, and it deposited lots of clay, alright? So, the north part of the Penokees, going towards Lake Superior, is the Lake Superior Clay Plain we call it. Lots of red clay deposited in that glacial lake there, okay? Which, of course, controls the soil and the way the water moves off the landscape today, alright?
[slide featuring a photo of the clay soil in the northern part of the Penokee range]
[new slide titled – Geologic Cross Section of the Penokee Range – featuring the same illustration as the previous cross-section (described above) now having Glacial Lake Duluth on the north end replaced by the Lake Superior Clay Plain]
So, there it is. There’s the geologic cross-section of the Penokee Range, alright? We – we did our quick tour through geologic time, and you can see in the south, these are the old – the old rocks, the 2.7-billion – billion-year-old package [uses mouse pointer to indicate the left-hand side of the illustration]. The ironwood package here [uses mouse pointer to indicate the middle section of the illustration]; the 1.85, that meteorite impact layer, would be in there, okay? This is the Midcontinent Rift volcanic rocks [uses mouse pointer to indicate the right-hand side of the illustration]. And then we’ve got the whole – oh, these are the sandstones [indicating a small layer between the rift volcanic rocks and the glacial deposits on the north side of the range with his mouse pointer]. And then we’ve got the whole thing draped in glacial deposits like that [indicating the glacial deposits across the top with his mouse pointer], with the two hard ridges standing up, making the Penokee Range, okay?
[new slide with the phrase – Why geology matters:]
So, that’s the story.
Now, why does it matter? Why do we care –
[Tom Fitz, on-camera]
– about this, alright? Why do we care what the geology is under our feet? And the first thing is resources. As I mentioned, minerals, energy, water, we all use that. Our society uses lots of it, and we need to understand those resources, okay? Another thing is the hazards, and hazards associated with resource use, but also the hazards that come from just living on this crazy dynamic planet, alright? And then, the last thing, as I mentioned, is understanding, alright, and it enriches your life to understand your surroundings. And I’m gonna show you some of the reasons why, okay?
First is resources –
[slide titled – Resources – featuring a photo of aluminum, steel, and iron pots and pans on an ovens stovetop]
– okay? Metals, particularly. If you’re gonna talk about the Penokee Range, you need to talk about metals, alright? And that’s my kitchen stove, alright, with a lot of iron and there’s a lot of iron there, okay? There’s stainless steel, there’s high carbon steel, there’s cast iron, alright, and I also turned all the burners on so you can see I’m using more natural resources there, okay? We’ve got some propane under the – under the – under the steel there, okay?
[the slide animates on an aerial photo of a strip mining operation to the right of the photo of the oven]
And all of that comes out of the Earth, right. All of our mineral resources, most of our energy, all of our water is somehow related to geologic processes, alright?
[new slide titled – Gneiss – featuring a photo from the Penokee range of stratified gneiss bedrock found in the southern part]
So, first let’s – lets take a look at the – lets take a look at the Penokee Range; what’s in there, okay? The ancient gneiss. That’s our friend the ancient gneiss there, the 2.7-billion-year-old rock, alright? There is a small amount of gold here and there, alright, and people have been looking at –
[Tom Fitz, on-camera]
– looking for gold in those rocks for a long time. Nobody’s made a lot of money at it, but people continue to look, alright? And I’m sure that theres – that they’ll be looking for a long time because there’s just enough to keep people interested, alright? Maybe more, okay, who knows, alright?
And then, of course, we have the banded iron formation, the ironwood –
[slide titled – Banded Iron Formation – featuring a photo of an iron infused rock cliff in the Penokee range]
– iron formation, which is, as I mentioned, 700 feet thick with lots of magnetite in there. There is a lot of iron there, okay? It is estimated that the Ironwood Iron Formation contains about –
[Tom Fitz, on-camera]
– 2.7 billion tons of economic, depending upon what the economics are, of ore, of iron ore, okay? That’s a huge deposit. And it has been – okay, it is part of the iron ranges. It’s one of the eight iron ranges in the western Lake Superior region.
[slide titled – The Iron Ranges of the Lake Superior Region – featuring a map of the western half of Lake Superior surrounded by Ontario, Minnesota, Wisconsin and the Upper Peninsula of Michigan and showing the following iron ranges on the map – the inactive Gunflint Range in southern Ontario, the inactive Vermillion Range in Minnesota near the Ontario border, the Mesabi Range of 7 active mines in northeast Minnesota, the inactive Cuyuna Ranges in central Minnesota, the inactive Gogebic Range in northern Wisconsin to the eastern part of the U.P., the inactive Iron River Chrystal Falls Range in the south-central U.P., the inactive Menominee Range in the southeastern U.P., and the Maquette Range of 2 active mines in the northern U.P.]
And most of the iron used in the United States has been mined out of the Lake Superior iron formations, okay? The Mesabi is the big one. That’s like 60% of all the iron used in the United States has come out of the Mesabi Range. Here’s the Gogebic/Penokee Range down here, which is inactive now. And then there are two mines over – still operating over in the Marquette Range. All of these rocks are related. They were all deposited in that shallow basin that I talked about, and they all have that – that, or most of them anyway, have that impact layer right at the top, okay?
[new slide featuring two photos, one an exterior shot of the Gogebic-Penokee mine in the 1800s and the second an interior shot of miners working a mine shaft in the Gogebic-Penokee mine]
So, there has been mining in the Gogebic-Penokee in the past. There was, between 1880s and the 1960s, there was 325 million tons of iron ore removed, mostly from underground mines, mostly high-grade, soft, natural ores, okay, which was mostly, the –
[Tom Fitz, on-camera]
– big pieces of it anyway were mined out, and what remains is the hard rock, okay, which is called the taconite. Its – taconite is banded iron formation that has an especially large amount of iron in it.
[slide featuring a photo of a shoe on top of an outcropping of taconite in the Penokee range]
So, less quartz and more of the magnetite or the mineral hematite. And there’s still a lot of it there, okay? So, there is still interest in mining in the Penokee Range, as you know. There was a company interested in mining it in the past, and the price of iron ore is low now, but who knows what will happen in the future? There’s still a lot of iron in the ground there, and there may continue to be interest in mining in the Penokees for the iron ore, okay?
[new slide titled – Hazards and Environmental Issues – with this phrase at the top of the slide]
And if we’re gonna talk about that, then we also need to talk about the hazards associated with potentially mining or the mining that has gone on there –
[Tom Fitz, on-camera]
– in the past, okay?
Hazards and environmental issues, okay, because all resource extraction and use has some environmental impact. We need to understand the resource. We need to understand the mining. We need to understand the extraction so that we can minimize the amount of environmental impact that we have.
Okay, remember this one –
[return to the cross-section slide of the 1.1 billion-years-ago strata of the Penokee range with the bedrock layer at the bottom, the uncertainty layer above that, the iron layer above that, the dashed impact layer above that, the second uncertainty above that and the volcanic layer above that – with magma entering from below and overflowing on top of the volcanic layer]
– going back 1.1 billion years ago when we had the Midcontinent Rift and that three million cubic kilometers of magma coming up through the Earth? Well, when it did, it came up through all these preexisting layers. So, there’s the Ironwood Iron Formation, and we’ve got lots of lava coming up through it –
[the illustration animates on a yellow layer to outline the red magma coming up from the bottom of the Earth and labels this outline – Hot!]
– and it heated it, alright? So, in some places, the iron formation and all the other preexisting rocks got very hot, alright? And hot enough to change, alright? And new minerals actually grew during this what we call a metamorphic process, a –
[Tom Fitz, on-camera]
– recrystallization because of the heat. So, what we need to do is we need to take a look at these places where – this is the ironwood, where the ironwood was heated up by being in contact with the magma, or close contact, and what we see are some different minerals in certain places, where the conditions were correct, alright, and the previous mineralogy was such that it could make these new minerals when it got heated. We have that brown layer in there.
[slide titled – Banded Iron Formation – featuring a photo of some of the ironwood formation in the Penokee range where the hot magma created new minerals which is browner than the surrounding rock]
Okay, that brown layer right there has got a different mineral in it. It’s not all quartz, okay?
[new slide featuring a microscopic image of the brown layer from the previous photo with the end of a pencil at the top of the frame for scale]
You can see it here, this brown mineral. This is – this is just taken under a stereo microscope, okay? And what I have here is that, right there, is my 0.5-millimeter pencil lead for scale, okay? So, that’s my pencil. And you can see this vein right here of this brown mineral, okay? It’s elongate brown mineral. It’s an amphibole mineral. It’s a group of minerals, alright? And the concern here is that, well, there are lots of long, skinny minerals, okay? The concern is, if they’re a certain –
[Tom Fitz, on-camera]
– size and a certain shape and a certain composition, okay, they can be very big hazards if inhaled, okay, or ingested, and basically what we’re talking about here is asbestos, okay? And so, we need to understand this mineral right here, okay?
So, next what we’re gonna do is we’re gonna take a look down the petrographic microscope, okay?
[slide featuring a photo of a petrographic microscope]
And that means that we’re gonna shine light through the rock, a very thin slice of the rock, and look at that through –
[new slide featuring a photo of the microscopic image of the rock which looks like a white substance]
– the microscope, and that’s what we see, okay? First, let me see here –
[new slide featuring another microscopic image of pencil lead]
– I want to show you, we’re gonna – were gonna be talking about scale. Geology, we talk about different time scales. We gotta stretch our mind into different time scales. We also need to think about different scales of geography, okay? So, we have to think about different spatial scales as well, alright? And this, right here, and we’re about to zoom in on this mineral. So, I want to talk a little bit about scale, okay? There’s my pencil lead right there. So that’s a 0.5-millimeter pencil lead under my optical microscope. And that is a 250-micron bar, okay? 500 microns is 0.5 millimeters, oaky? So, that’s 250 microns. And what I’m gonna do in these next slides is I’m gonna take that scale bar and stretch it out appropriately or skinny it down appropriate to scale, okay?
[return to the previous slide with the microscopic image of the brown layer that appears whitish under the microscope]
So, there’s the – theres the 250-micron scale right there, alright, and this is the microscopic view looking through the rock, alright? [uses his mouse pointer to circle a black area in the image] The black here is the mineral magnetite. That’s the one that the magnet sticks on. [uses his mouse pointer to circle the large white area] A lot of this is the mineral quartz. And then you see these little, brown, grayish-brown fibers in there. [circles these fibers with the mouse pointer] That’s the amphibole, and you can see that they are long and skinny little needles of stuff, okay?
[return to the microscopic slide of the pencil lead]
And so let us go, okay, there’s for scale. Okay, for comparison, a human hair, typical human hair from your head, is about 100 microns, okay? So, 100 microns would be like that. [uses mouse pointer to circle about half the distance of the 250 micron ruler in the photo] Okay –
[new slide featuring a much closer microscopic look at the fibrous areas in the previous microscopic image]
– so, there’s our 250-micron bar, alright? And in some places, the rock is mostly this mineral, this amphibole mineral here, okay? And you can see the pattern. It’s this crazy pattern. We’ve – weve been calling them bow ties. It’s like a three-dimensional bow tie, this fan of crystals that comes out like this, alright? And in some places, we get fans going all different directions, bow ties in all different directions, and the rock is – is mostly, you know, the majority of the rock is this amphibole mineral, okay?
[new slide featuring two microscopic images – on the left is the microscopic image from the previous slide showing the bowtie-like fan of the mineral, on the right is the same image now with a polarized filter on it where the bowtie effect is more pronounced]
There it is, same view but on the right, what I have here is I’ve got the polarizing filter in there so we can – that helps us identify the mineral. It is definitely one of the amphibole minerals, okay?
[new slide featuring a microscopic image of the end of one of the bowtie formations even closer up]
There it is, okay, one more view of it here, alright? What we’re gonna do next is we’re gonna zoom in on the end of this, these fibrous ends of that little bow tie right there.
[new slide featuring a new microscopic image of the end of the bowtie formation in an extreme close-up]
Okay, and that’s about as the highest magnification I can go with my optical microscope. Okay, there’s our 250-micron bar scale, and you can see that these things are really very thin, okay? So, the concern here is that if these things get lodged in your lungs, alright?
[Tom Fitz, on-camera]
If they become airborne and they get lodged in your lungs, they stay there indefinitely and eventually in – in some people they cause disease, okay? And what we’re talking about here is mesothelioma, which is a type of lung cancer that’s very specific to, it seems to be very specific to exposure to these amphibole minerals, these little skinny fibers of the amphibole minerals, okay?
So, there it is. It’s clearly a long, skinny –
[return to the previous slide with the extreme close-up]
– amphibole there, but to measure them accurately, we need to use –
[new slide featuring a photo of the same brownish area of rock now under an electron microscope]
– electrons, oaky? So, I put some of these rocks under electron beams in the Microbeam Laboratory at the U.S. Geological Survey in Denver, okay? So, that’s one of the rocks in the scanning electron microscope. And, okay, –
[new slide featuring the resulting microscopic image of the bowtie-like area from the electron microscope]
– so, the scanning electron microscope is an amazing tool, alright, because you can just zoom in and in and in and just see a whole another universe as you go into things, alright? So, that’s what we’re about to do. Alright, here’s our bar scale, you see the – the bar scale down here? That’s 200 microns, alright? And we’ll zoom in to this. Okay, that’s one of the bow ties there you can see, alright?
[new slide featuring an even closer image of the fibrous bowtie ends from the electron microscope]
And we’ll zoom in, there’s a 50 micron. We’ll zoom in again –
[new slide featuring an even closer look at the fibrous ends of the bowties in the mineral]
– like this, 20 micron. We’ll zoom in again –
[new slide featuring an even closer look at the fibrous ends of the bowties in the mineral]
– like that, 10 microns. And you can still see that it’s the skinny fibers, and you can start to see the cross-section, typical cross-section of the amphibole minerals as well.
[new slide featuring an even closer look at the fibrous ends of the bowties in the mineral]
One more, like that, five microns.
[new slide titled – Asbestiform – featuring the 5 micron image of the end of the bowtie formation on the left]
Okay, so, the – one of the – I mentioned that it has to be a certain size and shape and composition, alright? So, next we need to compare this to – what does it mean to be asbestiform? Okay, asbestiform is the shape that is especially dangerous.
[the slide animates on the first part of a list – 20 to 1 aspect ratio]
Okay, 20 to one aspect ratio. In other words, it has to be 20 times longer than it is wide, alright?
[the slide animates on the next part of the list – less than half a micron wide]
It has to be less than 5 – .5 microns wide.
[the slide animates on the point – Two of]
It has to have two –
[the slide animates on – parallel fibers – under the point – Two of]
– of the following, parallel fibers –
[the slide animates on – splayed ends – under the point – Two of]
– splayed ends –
[the slide animates on the two next conditions – Matted masses and curved fibers – under the point – Two of]
– matted, curved masses, I mean, matted masses and curved fibers, and we have all of those things, okay?
[new slide title – Asbestos – featuring a new electron microscopic image of asbestos on the left and the following list on the right – Serpentine and Amphibole. Additionally, under the heading Amphibole is the following list – Actinolite, Anthophyllite, Grunerite (which is in bold type), Riebeckite, and Tremolite]
So, the next question is what mineral is it? Most asbestos – asbestos is a commercial term, okay, so it describes a commercial product, alright? So, most asbestos is the mineral serpentine, and that’s most – most commercial grade asbestos into that – it appears to be less dangerous. The dangerous ones are the amphibole asbestos. And these are the amphibole minerals that are commonly asbestiform and have been used as commercial asbestos products, alright?
What we’re talking about here is the mineral grunerite, alright? So, this is asbestiform grunerite, okay, in quite large quantities. And it is known to be a very serious health hazard when it is this composition, and it is this shape, okay?
[new slide featuring an electron microscopic image of the mineral Grunerite close-up]
Alright, just one more, I’m gonna show you these. This is an especially photogenic one. Just amazing stuff, we’re gonna zoom into this –
[new slide featuring the same electron microscopic image of Grunerite even closer]
– 50 microns, 10 microns –
[new slide featuring the same electron microscopic image of Grunerite extremely close-up]
– and then we’ll zoom in. The 10-micron scale, is that it? –
[new slide featuring two photos – on the left is the previous photo of the brownish outcropping in the Penokee range and on the right is a photo of a person holding a baggy full of the brownish outcropping rock all crushed up]
Okay, yeah, that’s it, okay.
[return to the slide with the extreme close up of Grunerite]
[Tom Fitz, on-camera]
So, this is a serious hazard, okay? There – it has – every place where rocks rich in this mineral have been mined, people have died of mesothelioma, okay? So, this is a serious hazard here. And in some places in the Penokee Range, there’s quite a lot of it, alright? There are also places where there isn’t any because it didn’t get hot enough, okay?
So, that stuff, it’s not – its not soluble. It stays in the rock, okay, unless that rock is disturbed, alright? And, yeah, so there it is. It’s – its in the rock. Okay, and – but a more immediate question, alright, so that has, you know, big, it has to be considered if we’re gonna start mining that rock, okay? But a more immediate concern is, is it in the soil? Alright? Is places where the rock is at the surface and it has been disturbed. So, I took some place on that layer that –
[return to the slide with the two photos of Grunerite described above]
– I knew was rich in the grunerite, the asbestiform grunerite. I took some – just some rubble there sitting on the road, alright –
[new slide featuring an electron microscopic image of some of the rubble that was in the plastic baggy in the previous slides photo]
– and I put that in the electron beam. And this is a sand grain right back here. It’s actually a magnetite sand grain, and you can see sitting on there is a curved fiber. And we’ll zoom in on that.
[new slide featuring the same electron microscopic image as before now zoomed into the fibrous area of the image]
So, yes, indeed, there is some loose at the surface. In at least one place in the Penokee Range there is asbestos, okay, or asbestiform grunerite, alright, in the rock.
So, the United States Geological Survey is continuing to –
[Tom Fitz, on-camera]
– do studies there on the bedrock, okay, which is very important. That study is going on right now, alright? And I’ve given them all of my information, and then I am also continuing, actually just starting, a study on the soils to see – how widespread is it? And is it a health concern just like being out there on some of those dusty roads? Okay? So, that’s something we need to know. Alright.
Okay, the – the amphibole, the asbestiform amphibole is certainly a concern, okay, but there are also other concerns when it comes to mining in the Penokees in the Ironwood Iron Formation. And that is, okay, theres – this is ironwood. There’s magnetite right there. There’s some quartz, and that right there, that’s the mineral pyrite, okay?
[slide featuring a photo of the mineral pyrite in the Penokee range with a Swiss Army knife in the photo for scale]
And pyrite is iron sulfide, alright, which isn’t a problem when it’s in the rock, like that. But when that gets ground up and comes in contact with water and oxygen, it can make acid rock drainage, okay, which if done on a large scale can become acid mine drainage, okay? And it’s, again, it’s – its one of these things where we need to understand the resource. We need to understand the rock to see and understand what would happen to it if it were dug up, and would it create acid mine drainage or not? And it’s a complicated question. I mean, it’s, like, the geochemistry of – of waste rock piles is a – is a complicated thing, okay? But there certainly is pyrite there, and so that’s –
[new slide featuring a photo of a bedrock cliff with water running around it in the Penokee range at Copper Falls State Park]
– another thing that needs to be understood.
Okay, and iron isn’t the only resource in the Penokees, okay? There are also other resources. It’s a water-rich region, 33 inches of rain a year, shallow bedrock, lots of run-off, okay? So, we’ve got some beautiful rivers. This is actually at Copper Falls State Park, alright? Where, basically, the – the – the Bad River and the Tyler Forks River meet head on right there in Copper Falls State Park. It’s a beautiful, beautiful spot, okay?
[new slide featuring an aerial photograph of the forested area in the Penokee range]
And then another resource up there is just the – the vast wilderness, okay, lots of – lots of forested land, okay, wide open land, which is an important resource in itself.
And then down – downstream at the end of –
[Tom Fitz, on-camera]
– the Bad River, is the great big Bad River Slough and Kakagon Slough, which is some of the richest wild rice beds in – in the world, probably, okay, certainly in the Lake Superior region.
Okay, so, other resources. So, here we have it. We have resource, you know, we all depend on resources. We all use resources, and we have these competing needs, right?
[slide featuring three photos – the first, the previous photo of Toms oven with the pots and pans on it, the second is a photo of rapids of the Bad River in the Penokee range, and the last is the previous aerial photo of the woodlands in the Penokee range]
We need iron. We need clean water. We need clean air. We need wilderness space. We need forest products. We have all of these competing – competing demands –
[the slide superimposes a photo of crowded traffic, buses and people in an Asian city over the top of the photos of rapids and woodland]
– and we have an increasing population, alright, and a population of people that is using more and more resources as the standard of living changes, okay? So, these are complicated issues, alright? And so many aspects of our society –
[Tom Fitz, on-camera]
– come together around resource issues, alright? And more and more they’re becoming global, complicated global issues, okay? And we need to do this right, alright? We need to supply resources, and we need to protect human health, and we need to protect the environment for future generations, okay?
So, one of the things that needs to be done is we need to understand it, right? We need to understand the resources. We now have good enough science that we can –
[slide featuring a photo of a male instructor and two female Northland college students in gators gathering data in a river]
– understand what all is going on in those ecosystems, what’s going on with those rock systems and the geochemistry of the system, you know, and we need to understand that before – and base our decisions about resource use on sound science, okay? And we need to have the guts to do it right, you know. To – if we’re going to extract these resources, we need to have –
[Tom Fitz, on-camera]
– the regulations and we need to have, and we need to enforce those regulations and do it right. And then we also need to, in some places, say, You know what, this is an environment that is too susceptible, okay, to damage. And it’s like, no matter what the resource is, the other resources other than the metal are –
[slide featuring a landscape photo of the Penokee range with the two peaks in the distance with a wooded area in the foreground]
– actually more important, okay? And so, we’re gonna balance our resource use and make wise decisions. That’s what we need to do, okay?
[new slide titled – Understanding – featuring a photo of a student lying prone on a rock face as she examines it closely]
Okay, and then we come to the last part, and that is why it’s important to understand the geology of any region, including the Penokees, and that is to understand our surroundings.
[Tom Fitz, on-camera]
And this is probably my favorite part of studying geology and sharing it with people, and that is to, just the enrichment that comes from understanding the rocks and understanding your relationship to them, alright? And, I mean, just think about what we have looked and what we have seen in the rocks today, okay? Submarine volcanic eruptions. The evolution of the ocean and atmosphere system. Meteorite impact that changed the world. [laughs] Volcanic eruptions that repaved the Earth’s surface. Glaciation that scraped off and buried the whole landscape in a mile of ice. You know, it all happened right there. You know, it’s like, that’s great. You couldn’t even make that stuff up, you know. It’s such a great story. And, you know, there it is. You just, you read it in the rock, you know, and that is – that’s a beautiful thing –
[return to the slide titled – Understanding – described above]
– right there, okay?
[new slide featuring a microscopic image of kyanite crystals]
It is also beautiful in itself, okay, just to see it. And what I like to say is that the more you understand something, the more you see in it, and the more you see in it, the more beautiful it becomes, okay? These are kind of some kyanite crystals, the mineral kyanite, not really common but great geologic story in the kyanite that I pulled out of some rocks just a little bit to the east of the Penokee Range, sort of at the end, the east end of the Penokees – Penokee/Gogebic Range, okay? Some beautiful –
[Tom Fitz, on-camera]
– kyanite crystals under the microscope, yeah.
Okay, and then another thing is about the connections, okay, the connections between different parts of an ecosystem, different parts of the natural systems. You know, there it is.
[slide featuring a photo of a hand holding up the loamy, tilled soil of the Penokee range]
That’s the – thats the – the – the loamy, tilled soil of the Penokees, alright? And you think about what that, what its history is. You know, it was derived from this long history, you know –
[Tom Fitz, on-camera]
– and here it is at Earth’s surface in a different role, you know. It is now part of the Earth. It’s part of the atmosphere. It’s part of the hydrosphere. It’s part of the biosphere. You know, it all comes together there in soils, okay?
And we are connected to all of this through the soil, right, because, I mean, we – we eat the food, and we basically come from the Earth through –
[slide featuring a photo of a newly harvested field in the Penokee range]
– the soil and water, and that’s – thats a cool thing to understand right there, right, where you –
[new slide featuring a photo of trees in a forest in fall with their leaves yellowing in the season]
– where you come from, alright? And then also that everything, you know, this is this whole story, you know.? [laughs] You look at the geology of a place, you look at what’s going on in any ecosystem, and basically what’s going on there has to do with the –
[Tom Fitz, on-camera]
– geologic history. Alright, what are the materials that were left there by this long history of change through billions of years? Okay? What is the current climate? You know, the 33 inches of rain and the 43-degree annual temperature, you know. And what is recent disturbance, alright? So, the history, the – the current climate, and the disturbance, pretty much everything comes back to that, right? And, yeah, so this is what we get in the Penokee Range, and this is just sort of the – the last part of the story, alright, starting from billions of years ago. And it is an ongoing story. So, we think about geology just in the past, right, but really the past –
[return to the spiral of geological time illustration from the beginning of the talk]
– sort of spiraling up to today, you know, it’s a continuing story. So, what we have right now is like one glimpse, one short little glimpse of geologic time, but it continues. Alright? It goes on and on. And you know what? It’s fun to see that story. It’s fun to know your place in that. And, you know, it’s – its – its an honor to be able to read the rock and to share it.
[new slide titled – The End]
[Tom Fitz, on-camera]
Thanks.
[applause]
Search University Place Episodes
Related Stories from PBS Wisconsin's Blog

Donate to sign up. Activate and sign in to Passport. It's that easy to help PBS Wisconsin serve your community through media that educates, inspires, and entertains.
Make your membership gift today
Only for new users: Activate Passport using your code or email address
Already a member?
Look up my account
Need some help? Go to FAQ or visit PBS Passport Help
Need help accessing PBS Wisconsin anywhere?
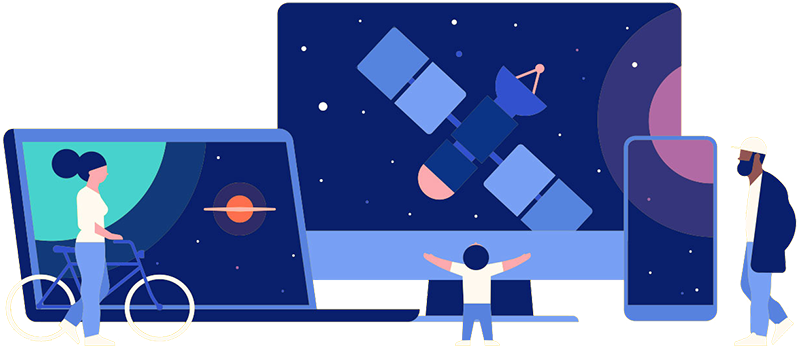
Online Access | Platform & Device Access | Cable or Satellite Access | Over-The-Air Access
Visit Access Guide
Need help accessing PBS Wisconsin anywhere?
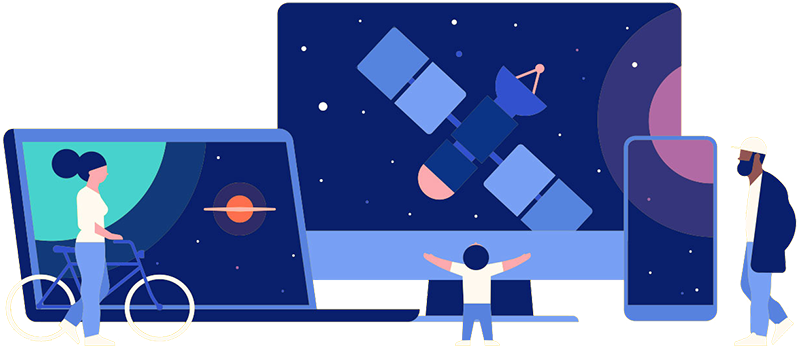
Visit Our
Live TV Access Guide
Online AccessPlatform & Device Access
Cable or Satellite Access
Over-The-Air Access
Visit Access Guide
Follow Us