– Welcome everyone to Wednesday Nite @ the Lab. I’m Tom Zinnen, I work here at the UW-Madison Biotechnology Center. I also work for UW-Extension, Cooperative Extension. And on behalf of those folks and our other co-organizers, Wisconsin Public Television, the Wisconsin Alumni Association and the UW-Madison Science Alliance, thanks again for coming to Wednesday Nite @ the Lab. We do this every Wednesday night, 50 times a year. Tonight it’s my pleasure to introduce to you, John Denu. He’s a professor here in biomolecular chemistry and at the Wisconsin Institute for discovery. John was born in Beloit, Wisconsin.
(audience cheering)(audience laughing)
– [Man] Here’s to Beloit, excellent.
– On the Bonnie Banks of the Rock River. (audience laughing) And as I pointed out to him, the water that flows out of Beloit flows into my hometown of Dixon, Illinois. And once again, I’m glad for sewage treatment plants. (audience laughing) John went to Beloit Catholic High School, which no longer exists. Then he came here to UW-Madison, which still exists. He studied biochemistry as an undergrad, then he went to Texas A&M to study biochemistry. And the most famous line about Texas A&M is:? 100 years of tradition unimpeded by progress. (audience laughing) (talking with Texan accent) Yup, an Aggie taught me that. Then he went to the University of Michigan for a postdoc, and then he was on the faculty at the Oregon Health Sciences University in Portland, Oregon. Then he came back to UW-Madison to be on the faculty here in 2003. Tonight he gets talk with us about Healthy Minds for a Lifetime: From Molecules to Mindfulness. Please join me in welcoming John Denu to Wednesday Nite @ the Lab. (applause)
– Thank you for– Can you hear me? Thank you for being here tonight, it’s a real pleasure to present some of the work that we’ve been doing.I’d Like to start off by introducing a very familiar concept, DNA. DNA is destiny. DNA is king. We hear comments about DNA all the time. It’s in our DNA, that’s what many companies try to sell you as a motto to convey a concept that is supposed to be so intrinsically linked to their brand that they’re really one and the same. An automaker may say, performance is part of our DNA, suggesting that they actually had no choice to build cars that are performance cars. It’s just what they do. We may they tell our grandson, you can’t help be as stubborn as you are (audience laughing) it’s in your DNA.
His father is stubborn as a mule, right? (audience laughing) So in actual biology, DNA is actually a polymer of genetically encoded information. Shown here is the familiar double helix with the four bases that make up the genetic code. So just a refresher on the biology of DNA. DNA encodes genetic information that’s transcribed to a single ribonucleic acid polymer, RNA. That information is translated to protein. That protein has a particular molecular function. That molecular function can give rise to the indicated phenotype. Now, here I’ve listed an example. The LCT gene, which codes for lactase, the gene when transcribed is made into a messenger RNA. That information is then translated to protein. And lactase is an enzyme, so it’s a protein enzyme which has a molecular function. It catalyzes the conversion or the hydrolysis of two of a disaccharide lactose into two molecules, glucose and galactose.
And the phenotype is essentially the ability of the organism in this case to digest lactose for energy or for other biopolymer formation. Now it turns out that there are actually polymorphisms or DNA changes in some human beings that allows them to — Well, that perhaps provides lactose intolerance or manifests as lactose intolerance. In other words, the inability to express enough lactase to deal with milk products that we ingest. It turns out that those mutations, that those changes in DNA that’s genetic. Those changes are actually not even in the gene. They’re in a subsequent gene, but they somehow affect the expression of the lactase gene. That’s somewhat understandable. But it turns out that there’s actually the expression of lactase, which actually decreases as we age. The sum of the expression of lactase has nothing to do with changes to DNA.
In other words, potentially, the expression of lactase among individuals might be totally dependent on the epigenome, okay? A set of instructions that helps regulate the expression of genes. It has nothing to do essentially with changes to the DNA sequence. So perhaps DNA is not king. Perhaps DNA is not our destiny. This was a Time’s article, I think about eight or 10 years ago. So this has tremendous impact for human health. The fact that perhaps DNA is not our destiny. I think this is great news. In fact I’ve always, over the last several decades, DNA has gotten a little too much attention I think. And we get our DNA sequenced, it tells us who we are, potentially tells us whether we have a disease mutation, right? We have mutation that correlates strongly or it correlates somewhat with a particular disease. Many of us have been told, one gene one function. There’s gauging, there’s an alcoholism gene. I never liked that. I think that’s bad science, but it makes life simple, doesn’t it? A lot of people want to know their DNA sequence.
And a lot of other people, I would say, don’t want to know their DNA sequence. They’d rather not know they have a mutation that gives them a higher chance of a particular disease, say cancer mutation. But I would say this. If you knew, if you had that information and you had information about your epigenome, about this information that sits on top of the genome that potentially can regulate the expression of that cancer gene, and you could find a drug or a lifestyle or a diet that influenced the expression of that gene based on your epigenome, wouldn’t you want to know that? So I come from the camp more knowledge is good, is better, okay? So let’s think about, let’s dig a little deeper into the epigenome and how it’s regulated. So at the level of control of gene expression, the epigenome essentially sits right here. The epigenetic control of gene expression sits between the encoded information in DNA and whether or not that information is transcribed into this message that ultimately will give you a functional gene. This is where the regulation occurs. This is where the epigenome plays a major role.
I like to think of, thinking about the genome and epigenome using this metaphor. The genome or the DNA sequence is like the hardware of the computer. And the epigenome is the software or the applications that you’re using at that time. So different applications give you very different functionalities, all with basically the same hardware. That I think is a reasonable way to think about the epigenome. Different functionalities but with the same framework, the same basic design. It’s the epigenome or epigenetics that helps explain the difference between two cells in our body that basically have the same DNA sequence but have very different functions. In the left we have a liver cell, hepatocyte. On the right, we have a neuron. And what makes these different? They have the same DNA. What makes them different is the differential gene expression in one cell versus the other. Not all genes are being expressed all the time. That wouldn’t work, right? So different functionalities are built-in based on what set of genes are expressed in a particular cell type. But this raises — This is a difficult challenge for a cell because it turns out that the DNA in each cell is about two meters in length. So that’s about like that, okay? So from the floor to there, not from the top of my head (audience laughing) to the hand. Just thought about that. So two meters of DNA in every single cell. And in most cells, you can’t even see without a microscope. But all that DNA has to get packaged into a little nucleus in each cell. So it’s important that the cell knows how to access the right genes at the right time. But just to give you a sense of how much DNA that is. I mean, every time I do this calculation it blows my mind.
But let’s think about — Here’s a recent example, okay? So we have enough DNA. We have a lot of DNA. We have about 37 trillion cells in our body on average. So imagine that you got to go hitch a ride on the New Horizons spacecraft and you got to take your cell phone, your smartphone and you’re heading to the edge of the solar system and with a rendezvous with Ultimate Thule. You’d have enough DNA — By the way, that’s 4,000,000,000 miles away. You’d have enough DNA in yourself in distance wise to go to Ultimate Thule, snap some pictures on your smartphone, come back to Earth, upload them on Facebook to your friends. But it turns out, the bad news is you forgot to bring your phone charger. But the good news is you have enough DNA left to come back to Earth, get your charger and then head back. The bad news is you don’t have enough DNA to come back home. (audience laughing) So that’s a lot of DNA. So there’s cell machinery in the nucleus that’s responsible for expressing a subset of those genes. And it has a difficulty or it could have difficulty trying to figure out in all that DNA what genes should I be expressing. That’s a lot of DNA. So how does it do this? It needs directions. Well, generally speaking, it’s easier to follow directions given with landmarks, which is why Google Maps has now adopted this practice. So instead of saying head north on North Southeast 23rd Street, we tend to like, “head towards the Columbia River and take a right at Taco Bell.”
That’s what the epigenome essentially functions, how the epigenome functions. It provides these landmark directions to the sites of genes that need to be expressed. However, they’re not quite landmarks in the sense that, I just, the example I gave. It’s different. These are small chemical landmarks. These are landmarks that are put on, these are chemical groups that are put on by cellular enzymes in our bodies, in the nucleus. So they directly modify DNA. Shown here, they can methylate these DNA bases that I showed you in the second slide. That turns out to be important information, important landmark information. The cell also modifies proteins by a whole array of modifications, chemical modifications. Again, directed modifications that are done by enzymes in ourselves. They can modify the protein. These are the amino acids that get modified on the protein, and these proteins are important because these are the proteins called histones that act as the molecular spools that sort of wind up the DNA, so it can be packaged neatly in the nucleus. That’s a set of chemical landmarks that the epigenome provides.
Okay, can the epigenome be changed? And what can change it? I’m not going to provide all the answers because we don’t know all the answers. I’m going to provide you with the kinds of things that we’re working on, the kinds of questions that keep us up at night. And I’ll provide a little bit of information about some of the results we have and some very preliminary data as well that we’re super excited about. Now there’s certainly strong evidence that environment, lifestyle and diet can have a profound effect on human health. But there’s more and more evidence that suggests that the effect is actually happening at the level of our epigenome. That we’re changing the landmarks in a way perhaps that are beneficial to us, but perhaps in ways that are not. And this can affect — Essentially this leads to potentially major alterations in our epigenome.
Two examples that I like to cite, one is from the Dutch famine near the end of World War II where pregnant mothers who suffered the famine, during the first two trimesters of the pregnancy, gave birth to children that later in life had a much higher incidence of metabolic disease that includes diabetes for instance. Some research in that area suggests this is an epigenetic phenomenon. And of course, it’s certainly not a genetic phenomenon because it happened at a fairly high prevalence. Then there’s a more controlled experiment here done in the laboratory. These two mice at the bottom of the slide here, these mice are genetically identical, which is hard to believe. The only difference is in the epigenome of the two, the difference lies in the epigenome on the two mice. Turns out there’s a genetic allele, a genetic location, that if it gets methylated, has an effect on gene expression of a series of genes that either make them lead to a mouse that’s sort of yellow and obese or a normal, brown, lean mouse. And this effect can be modulated simply by things in the diet like choline, or exposure to molecules like man-made molecules like Bisphenol A, which you guys have probably heard about.
So these are two, I think, quite good examples of the power of the epigenome and how it can impact both human and animal health. This is profoundly interesting to me in how life, how things like diet and molecules can affect the epigenome. And I’m a biochemist, so every stage of my training, I’ve been trained as a biochemist. I think in molecules, I think in mechanisms. I want to understand what’s happening at the molecular level. And so I want to briefly tell you about a study that we did looking for evidence, direct evidence that metabolism or metabolites themselves can have an influence on the epigenome. And there’s a very important reason why they do in this particular case. So we performed a number of experiments where we have genetically or nutritionally lowered the cell’s ability to make this molecule here, SAM.
Now SAM is really critical because SAM donates this little red methyl group to the epigenome. And here it’s shown as a little methyl group here on this part of chromosome, of this part of the epigenome. Interestingly, SAM comes from methionine. Methionine is an essential amino acid, and the methyl group from methionine is ultimately what gets transferred to this epigenetic information. Methionine, as I mentioned, is an essential amino acid. It comes from our diet. So this was one of the strategies that my graduate student, Spencer Hawes, PhD student in the lab did in collaboration with Dudley Lamming and Vince Cryns at the medical school to test how modulating SAM levels can affect the epigenome in both a cell culture model, as well as a mouse model, so up here at the top, I’m showing that’s a little petri dish with a million cells growing.
This is a mouse. Blue mouse. And so basically, we have altered either genetically or nutritionally the levels of SAM. So the levels of this metabolite that’s critical for methylating and modifying the epigenome. We extracted tissues from the mice after these studies, or extracted the molecules from the cell culture, both metabolites as well as epigenetic information in those histone proteins for instance. We put them through a number of experimental instruments to quantify the changes. This is the analysis, the statistical and analytics experiment analysis shown here. And ultimately, we’re quantifying how the epigenome has changed, okay, in response to that nutritional or genetic alteration of SAM levels. And the figure at the bottom is just to show you the dramatic changes that occurred, okay? I think the colors are a little muted in this projector, but this is a time course from 15 minutes all the way out to 24 hours. And on the right here, these are some of the landmarks, if you will, the chemical landmarks that I talked about that essentially can change within the epigenome. So there’s a dramatic effect of altering SAM levels. And we have shown, this has a huge effect on the expression of particular genes.
Now, from a number of studies I don’t have time to show you, we learn that the cell does everything it can to help maintain epigenetic information when there’s a metabolic stress like low SAM levels. It knows that’s really important. And we’re still here, so I think — I mean, throughout human evolution, hunter gatherers, Western diets, we’ve been able to cope with really crappy diets and starvation for long periods of time. So it’s not surprising, but we’ve uncovered some really cool mechanisms that we think are critical for the cell to help maintain that epigenetic information so it doesn’t forget it’s a neuron. So perhaps you’re on a vegan diet. It turns out the methionine is quite low in a vegan diet typically. So you have to be careful. You can actually take methionine as a supplement, but if you eat a lot of meat and other things, you might be getting plenty of methionine. There’s actually some health benefits to lowering methionine. We can talk about that perhaps in the Q&A session. But the bottom line is the cell is trying really hard to maintain this epigenetic information during a metabolic stressful nutritional stress. And we add back methionine, okay? So we restricted methionine, and then we added it back. And the cool thing is the system went right back to where it was. The epigenome went right back.
Now we didn’t extend this too far, but we did do one experiment. Spencer did the experiment. I didn’t do the experiment. Spencer, during that refining restriction, he added an inhibitor-like molecule, small molecule that blocks some of the pathways that we’ve uncovered, that were critical for the response to this nutritional deprivation. So it’s like taking the other leg on the stool, right? What happened then we gave — So this inhibitor blocked some of these pathways. When we gave methionine back, the cell didn’t get back to where it was. It was altered. Its epigenome was altered, and transcriptional, some genes that were being transcribed, there was a different set of genes. And we think this will probably lead to genomic instability and bad news down the road. Okay, so we think that factors that affect the epigenome include diet, lifestyle, stress, environmental factors. But we’ve been quite interested over the last I guess four-plus years now in the microbiome, the gut microbiome around the same time that I started working in this area through collaborations. I’m pretty confident that a fecal transplant saved my mom’s life. I’m pretty sure of that.
So, I’m a believer. I think the microbiome is having a pretty huge impact on us. Maybe some of us more than others. But this is a fascinating area that I want to tell you a little bit about and how it connects to the epigenome of the host in this case, okay? So the gut microbiota, I want to talk just about the gut microbiota. So over the last, I don’t know, five to 10 years I would say, there’s really been this tremendous interest in this area. There’s been a lot of really interesting correlative studies that suggest correlations between the communities that are in our gut and whether or not they associate with say diet, diabetes and obesity. Whether they associate with anxiety, whether they associate with depression, any number of human afflictions. And it turns out you can actually transplant a microbiota from a mouse that is anxious, has anxious phenotype, and put it into a germ-free mouse and make that mouse anxious. You can also take human microbiota, gut microbiota from an obese diabetic, and you can give that fecal transplant, if you will, from a human to a mouse and you can make that mouse diabetic. I mean this is mind — There’s something really cool going on. As a basic scientist, I want to understand that. A lot of people would like to understand that. But again, I like molecules, I want to understand who is responsible and how are they responsible, okay?
So we’ve evolved with bacteria. And for the most part, they’re our buddies. I think we’re now coming to appreciate, they’re more our buddies perhaps than they’re not. And in fact we get vitamins. They help supply us with vitamins, absorption of vitamins in our gut. They actually increase our metabolic capacity by 500-fold because of all the cool metabolic reactions that they can catalyze in our guts. So they take undigested polysaccharides and use them for carb, use them for energy. And some of the consequences of that, so the fermentation in our gut, the production, if we eat a lot of fiber for sure, is the production of these three short chain fatty acids: acetate, propionate and butyrate. Just want you to remember that for a second. So we set out to ask the question, do gut microbiota influence or affect the epigenome of the host? In this case we’re using a mouse model. This is a beautiful collaboration with Federico Rey, who’s a microbiologist here on campus, and we continue to work, as I’ll tell you in a second. And the majority of work that I want to briefly touch on was performed by an MD, PhD student in my lab, Kim Krautkramer. So we basically took germ-free mice. So here they are in a bubble, that’s to suggest that they are germ-free; and conventionalized mice or even conventionally raised. Conventionalized mouse is a mouse that began life germ-free and then was inoculated, okay? So it’s sort of another control, a good control experiment. So basically compared the germ-free mouse to a conventionalized mouse either on a sort of normal mouse diet, as well as a high fat, high sucrose diet and analyzed the tissues. In this case, that’s liver, colon and adipose tissue, so fat tissue, and quantified changes to the epigenome through that chemical information that I told you about previously. But the bottom line is yes, the epigenome is changed when comparing a germ-free mouse to a conventionalized mouse or even a conventionally raised mouse.
And it turns out that the changes were hugely muted under a high fat, high sucrose diet. We’re not sure exactly why that is, but essentially on high fat, high sucrose diet, the bugs don’t have much fiber. They have much to chew on, so they’re probably not producing the kind of small molecules and effect on the host as one might expect from a child that would have as percentage more fiber. Now the really sort of more basic science question here was, as I mentioned before, short chain fatty acids are produced in quite large concentrations in the gut for fermentation. This is acetate, propionate and butyrate. So we gave a germ-free mouse just those three in the drinking water actually. A germ-free mouse. And then we asked, okay, let’s analyze the tissues from these guys and compare it to see if that mimics, or not, being colonized. Because one of the questions we’re asking is what are the molecules that the bugs are producing or having an effect on that are affecting the host, affecting the host organisms, in this case the mouse, okay? Are there molecules that are mediating these changes? Data here is just, I think, striking. Essentially these are correlation plots and the right two panels basically compare a conventionalized mouse to a mouse that was germ-free but just given three short chain fatty acids.
There’s a really strong correlation. I mean, this is really good in these kinds of experiments, both in the liver and the colon. So three short chain fatty acids could mimic to a large extent the epigenetic changes that we noted in the liver and the colon. So, these molecules– Perhaps there’s other molecules that are sufficient to alter the epigenome in the host, right? And what are they? We want to understand that. So these projects have evolved, and our interest continued to evolve and we’ve become more and more interested in understanding this connection between the brain and the gut and largely right through changes in the microbiota, which can be affected by diet, by exercise, by all kinds of things as we’re learning about. So how does the microbiota influence the gut and therefore influence or molecules it produces or effects it has, how is it affecting the brain?
There’s been some really amazing articles that have come out. In fact I’ve highlighted two here, because this is from our colleagues here at Wisconsin. Federico Rey and Barb Bendlin. We published some really– We’re trying to follow up and I’ll tell you briefly about that on some of these observations. So Barb and Federico demonstrated that in Alzheimer’s disease, there is definitely an alteration in the microbiota, the gut microbiota of folks suffering from Alzheimer’s, as well as mild cognitive impairment. These are correlations, okay? So we don’t know if they’re causal. Also more recently, Federico and Barb published this paper, which again has to do with a molecule that’s produced by — It’s derived from gut microbiota, and that is trimethylamine oxide, which is elevated in Alzheimer’s patients, as well as those suffering from mild cognitive impairment. Trimethylamine oxide is a product of certain bacteria that can convert, for instance, choline in our diet to trimethylamine. And trimethylamine then gets taken up in our liver, it’s absorbed in the gut, taken up in the liver and then actually oxidized to trimethylamine oxide, and that’s usually what’s circulating at fairly high concentrations in our bodies and has been already correlated with cardiovascular disease and a number of other human afflictions.
In a somewhat related experiment, we wonder whether the epigenome could be altered in a model of Alzheimer’s disease. So we found that, and this is work from James Dowell, a scientist in my group, who showed that in two different models of Alzheimer’s disease, there are alterations in the epigenome. And those are highlighted by these two differences here, these two different models. So this shows the expression of a particular spooler, if you will, of DNA that is altered, as well as changes to these epigenetic chemical landmarks, if you will, on specific histone proteins within the AD model. So this suggests that there is an alteration at least in two mouse models of Alzheimer’s disease. There’s a change in the epigenome. And this is actually a hippocampal section, hippocampus, where most of the issues arise in Alzheimer’s. So I should mention we’re working with Federico on trying to establish what is trimethylamine oxide do. And we’re setting up an experiment where we’re going to look at mice, germ-free mice — Well it doesn’t matter the details — but we’re going to set up a mouse experiment so we can determine directly does trimethylamine oxide directly affect the epigenome and how? And that’s an experiment, a set of experiments, that’s to be done in collaboration with Federico Rey and a graduate student in my lab, Sidney Thomas. So stay tuned, we’re super jazzed about this. It’s like all of the planets are — to use another planetary or a space body — the planets are aligning I think. We’re very excited about the outcomes of that study. So continuing on with this idea of the gut brain access and something that we continue to be fascinated with is another pilot study that we’ve done with Ryan Herringa who is MD, PhD researcher. He’s a professor in psychiatry. Jo Handelsman, who’s our WID Director; and David Page, who’s from Biostats and Medical Informatics. We’re basically asking questions about the effect of the microbiome on adolescent depression.
What are the associated metabolic and epigenetic abnormalities in kids with, adolescents suffering from depression? Can a microbiome, metabolites and epigenetic profiles predict those that would respond to antidepressants, the SSRIs for instance? This is in some ways a path to personalized medicine, which I think we need still a lot of good basic science and the kinds of analyses that I’m describing for you today that are not routinely being done to do personal medicine. I prefer to think of it as precision wellness as opposed to precision medicine, because I think let’s stop it before it becomes medicine, okay, before medicine is required. So this is a pilot study we’re very excited about that again links these things we’re very interested in: the microbiome, epigenetics and metabolism that can link those two together. We’re also… This is the third study that has a connection to the gut brain access, so my lab and Jo Handelsman lab is partnering with the Center for Healthy Minds.
And we’re partnering with them on their Mindful Police study, where they’re basically asking the impact of a training program in mindfulness practices on the stress and stress-related health outcomes of police officers. So this is a fun study that involves the Madison police, university police and the Dane County Sheriff’s Department. And this is a grant originally funded, a grant to Dan Grupe from the Center for Healthy Minds. What we’re trying to do is partner with them and interrogate the alterations to the microbiome and the epigenome, and try to — These are really just beginnings, these are discovery experiments. But we’re in position where we can do it. We’re in a position that, what kind of new insight might we get from initially just correlations, right? Is the microbiome altered under three months of mindful practices, for instance? Does that alter the epigenome of immune cells, of our circulating immune cells, which can be responsible for initiating and accelerating some of the immune response and stress response under stressful conditions, okay?
So collectively at the Wisconsin Institute for Discovery, we’re sort of taking this group of projects, these pilot projects, and trying to build essentially a molecular pipeline where we do interrogate the microbiome, we do interrogate the epigenome. We can interrogate the metabolome, as well, so the metabolites that give us a sense of what’s changed. There’s other clinical markers that we could also integrate into this study. So this our depression, mindfulness and AD study. But what else can we fit into this pipeline? That is something we hope to build, a pipeline where folks can come to us and say we’ve got this really interesting cohort of folks that we’d like to understand the molecular basis of something that we don’t understand, okay? And so integration of multiomics is something we’re very keen on. Integrating that with clinical and epidemiological data. And imagine what can come out of this, right? Predict health outcomes, obviously. The possibility of diagnostics. Because there’s a lot of– This is very heavy data science, as well. We think there’ll be new innovation that comes out of the data science component of this. And this really builds on the strengths at Wisconsin Institute for Discovery where have strengths in epigenetics, we have strengths in the microbiome and we have strengths in data science, in addition to metabolism and stem cell biology. As I mentioned before, a lot of these studies are first-pass discovery experiments. And as you know, I’m a biochemist, we like mechanism. This is a starting point, right? And this might be okay for diagnostics, but ultimately one of the strengths of Wisconsin, one of many strengths of the University of Wisconsin is how many basic researchers we have on this campus. How many people like to dig into the weeds and understand how this thing works.
And we can test causality, right? That’s a key, testing causality. And I think what we’re going to end up with is a ton of hypotheses that we can test. Like oh, this molecule is upregulated under this condition. This leads to this epigenetic change. Are they connected, and how are they connected? Those are the kinds of things that we want to understand. And so having an autobiotic facility we have now on campus, we can use genetic mouse models. And I’ll show you just in the last slide or two examples of how we want to adapt and utilize organoids to study. We can study humans the way we can study mice because we can get tissues out and do genetic experiments, but there’s a lot we can do using organoids in vitro, okay? So these are the examples of the kinds of things that can be done to test causality. And that’s an important consideration. So one of the things I want to leave you with tonight is something that we’re excited about that’s really, I would say, a consequence of all of these experiments and interest in the gut brain access. And we’re putting together a grand challenge. So what is that? Well, that’s like an audacious goal, right? We’re calling it BrainSPAN 100. The idea is this. People are living longer, but are our minds keeping up?
So a hundred-year lifespans are not that crazy. I mean, the predictions are pretty amazing in terms of what human life expectancy is and is going to be. So can we have a full life, a hundred-year lifespan without issues of the brain, okay? Whether that’s autism, whether that’s depression, whether that’s dementia and Alzheimer’s, anxiety, okay? So can we have hundred-year lifespans without, or, sorry, I should say, with a healthy brain? So we want to characterize treatable brain disorders with this multiomic analysis. We want to see commonalities among these various human afflictions or disease or conditions. Alzheimer’s, PTSD for instance, depression, maybe autism, anxiety. And pursue treatments, really precision medicine, precision wellness really that’s based on this new data and this new understanding. We want to unite diverse researchers across campus to tackle this problem. So this is sort of the hundred, yeah. This is just sort of more indication of areas that we try to connect. Do you want to live to be a hundred? Maybe. If your brain, if the system was okay, you’d probably say, “Yes.” How much do we need to think about our epigenome? And how much is our microbiome playing a role in this process? Those are some of the questions. So this is almost science fiction, but these are real images from several colleagues that have been trying to get together to jumpstart a project that’s really… It’s really almost science fiction, but I think we can do it, okay? And that is, can we build in vitro a gut brain model?
We have colleagues, Randy Ashton, who’s building, who’s making from human stems, adult stem cells, making little organoids on a slide in a uniform way, little brain organoids. Our colleague, Laura Knoll, is building intestinal organoids and she’s co-culturing these with bacteria. So in vitro and using microfluidics devices. So wouldn’t it be cool, and other folks like Eric Shusta, has built a very beautiful blood-brain barrier so we could make a brain organoid, right? We can make an intestinal organoid here that’s co-cultured with the bacteria that we say, okay, let’s put these strains of bacteria in and measure these metabolites out. Let’s fuse through microfluidics, perhaps even — This is also an attempt to vascularize the whole thing, which would be super cool. But basically, can we connect them and ask in a very controlled, quantifiable way, how is the gut talking to the brain? And how can we understand some of the molecular events that lead to dysfunction in the brain through molecules, through — And ultimately, we want to understand how gene expression and development are affected in, for instance, the brain organoids sort of as an end point. But of course, these two are talking to each other so you can imagine connecting it through the other direction. Okay, as most grant challenges are supposed to be, it’s audacious, it’s bold. But why not? Right?
We are trying to crowdsource some of these solutions from both researchers and the public. Ideally, we want to accelerate the progress on this project and get input from diverse groups of folks that includes the public and includes researchers. This is a large undertaking. It’s going to take a lot of folks with a lot of diverse skills. We will be planning a town hall where we would love both researchers on campus that want to get involved, as well as the public, to see what can be done to have input into the kinds of solutions that we may come up with. So if you’re interested, please contact Nolan to stay connected. And get on our email list and we’ll keep you informed on events and when this town hall will be scheduled. It hasn’t been scheduled yet. How do we connect people? Perhaps that’s — I mean, just engaging with you tonight is a way to connect with and let you know what we’re trying to accomplish, okay? And you may say, “Oh John, I know this researcher at the Marshfield Clinic who’s doing something that overlaps with stuff you’re doing. Do you know this person?” And I’ll probably say, “No, I don’t know that person. Can you connect us; can you make the email introduction?”
I can’t tell you how many times that happens. And just by engaging in people, you realize there’s some connections, there’s ways in which we can help each other solve these really important questions for human health. So other things perhaps that can be done through sort of crowdsourcing solutions. Raise awareness of this goal to inspire others. It could be within community groups, it could be our elected officials. Can we volunteer, perhaps volunteering, organizing events and fundraising. You could be a participant. We could all be participants in some of these studies. Donate funds and so forth, and even engage in the entrepreneurial side of things because I think if we’re successful, there’s going to be lots of aspects of success here, which could be data science packages, it could be diagnostics, it could be opportunities for entrepreneurialship that perhaps even folks in this crowd, that’s your area and you want to get engaged and be involved. So, I think I will leave it at that and hopefully have plenty of time for questions. So thank you all for coming tonight. It was a pleasure.
(applause)
Search University Place Episodes
Related Stories from PBS Wisconsin's Blog

Donate to sign up. Activate and sign in to Passport. It's that easy to help PBS Wisconsin serve your community through media that educates, inspires, and entertains.
Make your membership gift today
Only for new users: Activate Passport using your code or email address
Already a member?
Look up my account
Need some help? Go to FAQ or visit PBS Passport Help
Need help accessing PBS Wisconsin anywhere?
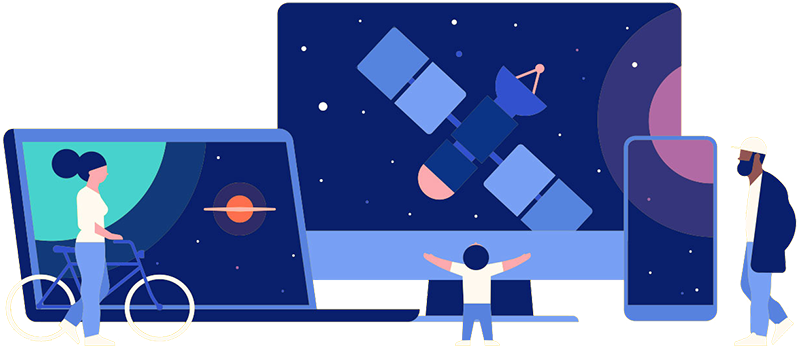
Online Access | Platform & Device Access | Cable or Satellite Access | Over-The-Air Access
Visit Access Guide
Need help accessing PBS Wisconsin anywhere?
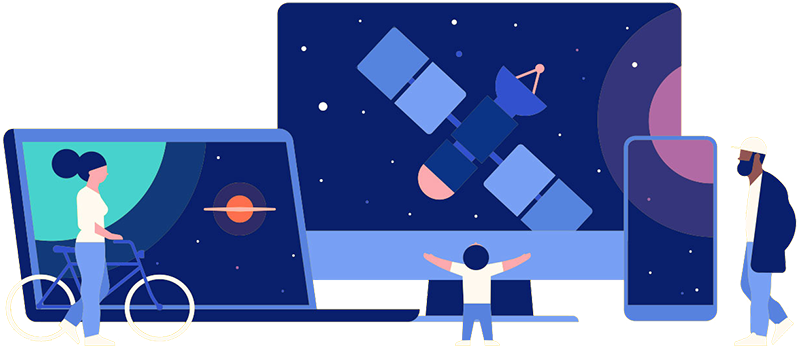
Visit Our
Live TV Access Guide
Online AccessPlatform & Device Access
Cable or Satellite Access
Over-The-Air Access
Visit Access Guide
Follow Us