– Welcome everyone to Wednesday Nite @ the Lab.
My name is Liz Jesse, and I’m a science outreach specialist at the UW-Madison Biotechnology Center.
On behalf of the Biotech Center, UW Extension, Wisconsin Public Television, the Wisconsin Alumni Association, and the UW-Madison Science Alliance, I would like to thank you for coming out tonight on a rainy night for Wednesday Nite @ the Lab.
We do this every Wednesday night, 50 times a year.
Tonight, it is my pleasure to introduce Dr. Andrea Strzelec, all right.
Dr. Strzelec is the program director for the Master of Engineering in Engine Systems online degree program in the College of Engineering.
She’s also an associate research scientist in the Departments of Mechanical Engineering and Nuclear and Engineering Physics.
Tonight, Dr. Strzelec will discuss energy terms that the public needs to understand in order to make wise decisions about energy use and public policy.
So Dr. Strzelec was actually born in Milwaukee, Wisconsin.
She is local.
She went to high school in Waukesha Catholic Memorial and she is a UW-Madison triple threat.
She got her BS in biochemistry, her master’s in mechanical engineering, and her PhD in combustion engineering.
So ladies and gentlemen, please join me in welcoming Andrea Strzelec.
[audience applauding] – Welcome.
Welcome to Energy 101.
As she said, I’m Andrea Strzelec.
And so if you’re wondering what do I mean by Energy 101, well, I really mean energy literacy, or as some of my students might call this, thermodynamics without the math.
So if I look familiar, you may remember me.
In January of 2021, I came to you live from my basement.
So it’s really a nice pleasure and a treat this time to be here in person and seeing live faces.
Now, the reason I’m here tonight is because we had such awesome feedback from the last talk, and I got lots and lots of great questions on energy.
And so the idea was born to create a talk on some energy literacy.
So when I talk about energy literacy, it empowers people to make informed energy decisions and understand the economic, ethical, societal, and international factors that guide energy choices and decision-making.
These factors are intertwined, and as I talked about in my previous lecture, and why life cycle analysis is so important to make sure we see the whole picture.
So that’s a bit of a spoiler alert.
So energy literacy requires that we understand energy systems, physical energy processes, biological energy processes, and energy transfer.
So here’s my outline for tonight.
We’re going to talk about what is energy.
We’re gonna talk about the difference between energy and power.
Those are terms that people use interchangeably, and it’s kinda like nails on a chalkboard to me as a thermodynamicist.
So we’re gonna talk about energy conservation ’cause that’s an interesting topic, and the problem with entropy.
And then we’ll talk about how we properly evaluate energy use and compare apples to apples.
So at the end, we’ll revisit my previous talk topic, and we’ll see if you can spot, you know, I have a test for you when we get to the end.
So when I say the word energy, this is probably what you think of: electricity and its uses, and that’s definitely true.
That’s only part of the overall energy picture.
So energy is one of these words.
It’s ubiquitous, right?
We use it all the time.
But I think that this is a great quote.
“The more basic a physical notion, the more difficult it is to define in words.”
And that’s certainly true for, well, like I said, for a word that we use all the time, it’s amazingly difficult to define.
And if we look at the physics textbook, it says “the capacity to do work.”
Well, that’s not particularly clear either.
The U.S. Department of Energy says that “energy is a physical quantity “that follows precise natural laws, and that often, “energy can be indirectly observed by observing processes.”
Super clear, right?
Like a plate of spaghetti.
So let’s try on our own.
If we think about energy, energy has no physical essence.
It’s not a thing, right?
We can’t grab a handful of energy.
There’s no such thing as pure energy, despite what Depeche Mode would, you know, have you believe.
It’s always carried by something, like me, right?
I am carrying energy.
Energy can exist and be stored in various forms, and we’ll talk about some of those forms.
It can be converted from one form to another.
I look forward to converting a pizza form of energy into my physical energy later.
And it can be transferred between systems by processes.
So if I had to come up with a definition of energy, I would say that energy is the ability to create change.
Sometimes it’s easier to think about energy in terms of its sources.
So sorry if that’s a little bit small, but on the left, we have coal and nuclear.
We have oil, we have natural gas.
Then we have some more renewable sources like solid municipal, solid waste, and hydrogen.
And then we have the green power, like wind and solar and biomass and geothermal and biogas and biofuels.
And then as I mentioned, food is also an energy source, right?
And the interesting thing about all of these is if we think about what’s the one energy source that all these things came from, it’s the Sun, right?
The Sun grew the biomass that the animals eat that we then eat as food.
The Sun grew the biomass that the dinosaurs ate, and the dinosaurs then died and became the oil in the ground and the natural gas.
Everything tracks back to one major energy input source to our planet, coming from the Sun.
Now, energy uses are equally varied.
There’s my favorite, the nice Ford Power Stroke diesel there.
It’s a 6.7 liter, in case you were wondering.
Wayne Gretzky playing hockey, another fan favorite of mine.
My beautiful computer and phone, the electricity that I need to plug those in.
Those are uses of fans and hot water heating and, you know, all the kinds of uses you use around the house.
And then that last square is energy and the body.
The food energy gets transformed into ATP, adenosine triphosphate.
See, she said I had a biochemistry degree, which is the energy carrier that you use in your cells in the body.
So energy has lots of forms.
It has lots of uses, and, you know, for example, what you may feel like– You can use it up too, right?
With few exceptions, very few.
The use of, like, solar, we’re not depleting that, but the use of energy depletes the source.
So for example, when you feel like your energy is used up because, you know, you’ve been playing a fantastic game of hockey and you’re exerting yourself, it’s because your body is using the energy that you have carried in your blood faster than it can be replenished by your body transforming the food into ATP.
So this is another plot I’m sure you’ve possibly seen before.
I think that I’ve shown it before in my talk or a couple weeks ago, I know there was a talk that showed it.
This is, on the left, energy sources, and on the right, energy uses in the United States.
And so what you see is we have petroleum, natural gas, renewable energy, coal, nuclear.
A lot of it goes to electricity.
Other goes directly to transportation or industrial processes or residential power or commercial power.
So when we think of energy, right, we tend to maybe focus a little bit on electricity, but it’s much, much broader than that.
And we can think about it in terms of, you know, what its source is, what its use is, and the transformations we need in order to do that.
So some of the forms that energy takes, it can be thermal.
It can be kinetic; that’s the energy associated with motion.
It can be potential.
So related to the gravitational field, right?
If I dropped a ball and it hit the ground, that had potential energy.
Oh, I apparently like thermal so much I listed it twice.
[audience chuckling] Radiant, so solar energy.
It can be sound.
Sound can be energy waves.
And then we have electrical, electromagnetic, mechanical, chemical, and nuclear.
And those forms on the right-hand side mostly involve motion, right?
Especially we’ll talk about electrical energy, right, as it flows through wires, we’re talking about the motion of electrical energy.
So energy can undergo transformations, as I mentioned.
So you can go from one form to another.
We can turn electrical energy into the sound and light of our TV.
We can turn light into the chemical energy stored in plants, right?
We can turn chemical energy and batteries into light.
So we can do light into chemical and chemical back into light.
We can, you know, do chemical, our food, into mechanical energy that we create.
Electrical energy can become thermal energy in your toaster.
My favorite, chemical energy can become mechanical energy in your vehicle, and that’s what engineering is all about.
Transferring energy from one form to another to do something useful.
So we also need to define energy transfers.
So there’s two kinds of energy transfer.
There’s thermal energy transfer, which we call heat.
So it is incorrect to say heat transfer.
We do thermal energy transfer, and that process is called heat.
And then work, which is pretty much all the other kinds of energy transfer.
So you can have moving boundary work, you can have paddle wheel work, you can have shaft work, you can have spring work, lots of forms of work.
And what I always tell my students in thermodynamics is heat’s really easy to recognize.
You’ve got conduction, convection, and radiation, and if it’s not one of those, it’s work.
But these energy transfers require a gradient.
They require some sort of difference, right?
So for example, in order for thermal energy to transfer, you need a hotter body and a colder body.
If they’re the same temperature, there’s no thermal energy transfer.
So there’s always some kind of gradient, some sort of potential difference that’s required in order to transfer energy, whether it’s thermal or mechanical.
Now, the larger the gradient, the faster the energy will flow, and that means more power.
And we’ll see that in a minute.
At a higher voltage, more electrical energy flows.
Okay, what about power?
Well, talking about transfers also brings in time dependence.
We talked about the rate at which work happens, and that’s really important.
Energy is not the same as power.
People use them interchangeably, but they’re not the same.
Power is the flow rate of energy.
It’s the rate at which energy is transferred or used.
So you get an electrical bill.
But what that actually is is an aggregate amount of the total energy you used, even though you used it at some rate, you used power.
So for example, running more apps on your phone requires a faster power draw, right, from your phone, and it’ll make your battery die faster because it’s more quickly depleting the energy that’s stored in the battery.
Now, so the rate; by the way, we talked about work transfer.
Work, the amount of energy and work at a particular time rate is power.
When we talk about the rate of thermal energy, we just say that heat transfer rate.
So a little bit more straightforward there.
Talking about energy versus power, your electric bill tells you how much energy you used in kilowatt hours over a total period of time.
So you get a bill at the end of the month, and it takes that power draw that you did and integrates it and gives you a total amount of energy used.
A battery, for example, contains energy.
But when you draw that energy outta the battery, you’re using it as power.
So they’re related concepts, but they’re not the same, and it’s not words that can be used interchangeably.
So another one, and this is the one that I always use to help me remember, you transfer chemical energy as fuel into your car, but your car is propelled by the power train, right?
You’re drawing it out as power.
It’s that time rate of energy that you’re actually using there.
Okay, so now we’ve talked about, like, kind of what energy is.
It’s the power to create change.
We’ve talked about forms that it can handle.
We’ve talked about ways to move it around.
Now we need to talk about some sort of overarching way of looking at total energy, and that’s where energy conservation comes in.
So the first law of thermodynamics.
It was defined in the 19th century by James Prescott Joule, and he was awarded the unit name for doing so.
So we often talk about the SI unit name for energy is joule.
A joule per second is a watt, not like the Watt brothers that famously all played football here, but the power one.
Energy, the first law of thermodynamics says that energy is not created or destroyed.
That’s the one you’re probably most familiar with, right?
People think energy’s neither created nor destroyed.
Energy is conserved.
That’s the first law of thermodynamics.
And it turns out that in the math version of this, which by the way is Mechanical Engineering 361 or EPD 361.
That is, the mathematical version of this shows that it’s a mathematical model for energy conservation.
So we can use this idea to mathematically quantitate transfers and forms of energy.
I promised you no math tonight.
I’ll keep my word.
So in order to look at this, in order to, like, define these things, the very first thing that we have to do, and if you saw my other talk, you saw a version of this slide, too, is define the system.
So the system is whatever we wanna study, right?
It can be my cell phone, it can be my computer, it can be an apple, it can be a can of Diet Coke; doesn’t matter.
Whatever we want to investigate, that’s our system.
The surroundings is everything else.
So anything not in the system is in the surroundings.
And the defining line between the system and the surroundings is what we call the boundary.
Transfers of energy are defined at the boundary.
So when it crosses from the system into the surroundings, that’s a transfer.
When it crosses from the surroundings into the system, that’s a transfer.
Within the system, there are no transfers.
Within the system, you have forms.
So the forms exist within the system, and those amounts change due to the transfers.
So the first law of energy conservation says in all that the sum of energy transfers into and out of the system are equal to the energy changes held within the system in its various forms.
So this would’ve been math, but I changed it into words for you.
Now, this isn’t a closed system.
So if we’re looking at something where there’s no mass going in and out, ’cause mass also carries energy, we can also look at it for processes which occur at a rate.
And then all you have is the time rate of change of the energy is equal to the sum of the transfers in and out and changes of energy held within the system.
So that’s gonna be important.
We’re gonna talk about this because what we need to take away from this is that transfers of energy in and out, it’s kinda like your bank account, right?
You get deposits in, you take withdrawals out, and what that changes is the balance of money that’s in your bank account.
Same thing works for energy.
My dad’s an accountant, so he probably really likes that analogy.
Okay, the other thing we have to define just briefly is what is a cycle?
A cycle is any process that begins and ends in the same state.
So if I were to walk all around this room and end up right back exactly where I am now, that’s a cycle.
If I take two steps there and two steps back, it’s the exact same space.
That’s a cycle.
If I take two steps that way and then four steps over there and then six steps back and then come back to where I am, still a cycle.
A cycle has to be at least two processes.
Can be many, many more.
But it’s a sequence of processes that are connected and eventually return to the initial condition.
And that’s important because that’s how we make power.
We generate power, energy delivered on a time rate by doing power cycles.
So what we have is we have some sort of hot body.
That hot body can be burning fossil fuels, it can be coal or natural gas, it could be burning biomass, it could be burning gasoline in an engine.
It could be a nuclear reaction; it can be solar energy.
And then there’s some sort of system it’s transferred into, process takes place, power is generated.
That can be electricity through a generator, it can be shaft power to move a vehicle, it could be jet engine thrust.
And then, whatever energy didn’t get turned into power gets rejected to generally the atmosphere.
So that’s either through a cooling tower if you’re at a power plant, or through your exhaust pipe if you’re looking at an engine, or maybe into water, a lake or river, an ocean; often, power plants, right, send their effluent water out into the lake.
So you’re taking energy in, you’re converting some of it to power, and the rest is going out to some lower temperature source.
This sets up what I really wanna talk about, which is the idea of efficiency.
When we define efficiency of any energetic process, what we’re interested in is what we want, so usually power, over what we had to do to get it.
And going back for a second, that was that hot body work, right?
That’s what we had to do to generate the power.
We had to use a nuclear reaction, we had to burn something, but it’s the power coming out that we want over what we had to put in to get it.
So this idea of thermal efficiency is based on the conservation of energy.
Assuming that work and thermal energy transfer or heat are interchangeable, and they are.
Transfers can become transfers.
Transfers can become forms.
Forms can become transfers.
And the theoretical limit based on the conservation of energy is 100%.
But we know that’s not true.
We know that we’re not going to get 100% out, and that’s the beginning of the limitations of the first law.
So the first law does not tell the whole story when it comes to energy.
All energy is not equal.
There is something that we call the quality of energy.
So you can do more with high temperature heat than you can do with low temperature heat, right?
If you have a thermal source that’s at very high temperature and you have one that’s at, like, low temperature, you can do a lot more with this one, right?
We know these things instinctively even if we don’t know them formally or mathematically.
And so energy, all energy is not equal.
Energy has different quality.
The first law is also missing the ability to tell us which way a process will go.
Now again, you know this, but the first law doesn’t tell me if I have a hot cup of coffee out on this table, will it get hotter, that’s possible by the first law, or will it not?
Will it get colder?
First law doesn’t tell us that.
And the first law also doesn’t describe the lost work potential of energy.
So when you have energy and you don’t turn it into work, that’s a real bummer, right?
That’s lost work potential.
And that is something we have to find another way to get to.
So when we talk about the quality of energy, as I mentioned, all energy sources are not created equal.
Work and heat are not equal.
And here’s the way I like to think about it.
You can turn money into food, but you can’t always turn food into money.
If you’re a really good chef, you probably can.
You probably turn the food into more money.
But you can always turn money into food.
It doesn’t always work in the reverse.
And that’s because energy has two parts.
It has a good part, something we can do something with.
And that’s what I’m referring to as work potential, and it has a bad part that we can’t.
So we also talked about the directionality, and I will tell you about that in just a minute.
The directionality of a spontaneous process, well, you know, water runs downhill.
A hot cup of coffee on the counter gets colder, but a cold glass of water on the counter warms up.
So wait a minute, we just said the hot cup of coffee gets colder, but the cold thing warms up.
That seems counterintuitive, but you know, ’cause you’ve seen it, that that’s exactly what happens.
In each case, the process also will not spontaneously reverse itself.
My hot coffee that has gotten cold requires the microwave to get it hot again, right?
To restore that initial condition takes effort.
You know this, you see this all the time.
Even if you couldn’t mathematically put numbers on it or put a name to the formalism, you know this from your lived experience.
You really know more thermodynamics than you think.
So work potential, let’s talk about that for a minute.
When a system is not in balance, which our fancy word for is equilibrium, with the surroundings, a gradient exists.
And remember, it takes a gradient to do transfers.
So, and work is a transfer.
So if a gradient exists, we have an ability to do work.
The potential to do work is called availability, or exergy is the other name for that.
And that’s the good part of energy.
So here I have a balloon.
The balloon is expanded.
That balloon has the potential to do work.
We could untie the balloon and blow that air at something and do some work, probably some moving boundary work.
But while that balloon exists, while that air is in the balloon, while that air is pushing against that boundary, that balloon has the potential to do work.
Now, availability and energy are related, but they are distinct.
When you fill a vehicle’s tank with fuel, it’s the availability of the chemical energy in the fuel that you want and what you pay for.
So let’s choose a room-temperature cup of gasoline in an air atmosphere as our system of interest.
So I have a cup of gasoline.
We’re imagining this ’cause obviously it’s not a great idea to have a cup of gasoline in this room.
We have a cup of gasoline in a acrylic box filled with air, and that’s our system of interest.
Right now, that gasoline has value, right?
We know it’s probably $3 and, you know, 59 cents or whatever a gallon, right?
We know it has value, but then suppose we burn that fuel.
So now we’re left with a slightly warm mixture of air and combustion products, and it’s only slightly warm because there’s a lot of air compared to a little bit of gasoline, right?
And so the temperature of the air now is slightly warmer than it was before.
The energy in the system will have remained the same because that box is constant and none of it has been transferred in or out, none of it left.
Energy is conserved.
However, we’ve lost the work potential of the fuel.
It’s been spent.
It turned into thermal energy.
So that’s the difference between energy still the same and availability or work potential that has been depleted.
So we need a way to quantify that lost work potential.
If we don’t exploit the process to develop work, that is lost irrevocably.
So I just burned that imaginary gasoline and air, and I made warm air and it’s gone, and we’re never getting that back.
So for example, if I take my balloon and I pop him, no more work potential, and we can’t get it back, right?
So if we think about it, the initial fuel air combination has a lot greater potential for use than warm air does.
The energy in the system will have remained constant.
So the energy is conserved.
However, we lost that work potential of the fuel.
We can’t use it in a car instead.
And the fuel also had economic value, whereas warm air does not, right?
Or we’d be really cleaning up with what’s outside right now.
Availability, work potential, has the economic value.
That’s what we care about.
So when I say energy has two parts, has the work potential, that’s the part we care about, and then the bad stuff.
And we’re gonna get to that in just a second.
The work potential of energy is what we pay for.
That’s why we pay for electricity.
That’s what we care about, right?
So, and well, I’ll save my question for just a minute.
So the limitations of the first law, it doesn’t tell the whole story.
All energy is not equal, and the first law treats it as such.
It’s missing the ability to tell us which way a process will go, and it does not account for that lost work potential of the energy.
So that’s why we have the second law.
The second law of thermodynamics is what tells us the direction that a process will take.
It’ll establish the conditions for equilibrium so that we know if work potential exists and when it’s used up.
It’ll help us to determine the best theoretical performance of cycles.
And that comes in handy later, you’ll see.
And it also gives us a qualitative way for evaluating the factors that keep us from attaining the best theoretical performance.
And that is through defining a property that you’ve probably heard of called entropy.
What you probably already know about entropy is that the entropy of the universe is always increasing.
A physics book will tell you that entropy is the measure of a system’s thermal energy per unit temperature that is unavailable for doing work.
Well, if energy is the capacity to do work and entropy is the part of the energy that’s not available to do work, we’re starting to tease this out here.
But entropy is that bad part of energy that I mentioned.
It’s the part that we can’t do anything with.
When a system is not in balance with the surroundings, a gradient exists and there’s a potential to do work.
And when that work is done, we’ve either gotten some work out of it, and what was left over is the entropy.
So how energy is conserved, but we still need to turn the lights off is because energy’s two parts.
The good part, availability, that’s what we pay the power company for.
And the bad part, that’s entropy.
And the fact is availability or work potential is always decreasing.
Every real process we do degrades that work potential.
So when we transfer energy from the power plant to your home, we’ve degraded it.
It’s not all still available for you to do work.
What MG&E generates is not 100% used by all of us out here.
A lot of it gets lost to entropy generation that happens as it gets transmitted over the lines.
When you use it to charge your phone, more of it gets degraded.
Availability or the potential to do work is always decreasing, and that work potential is lost to generation of entropy.
So you’re right, the entropy of the universe is always increasing, and that’s because the work potential of the universe is always decreasing.
So we talk hear people talk about happy thoughts like the eventual heat death of the universe.
That’s where it comes from.
Eventually, we just use up all the work potential and all we have is the chaos and the bad part.
Energy stays constant because the availability decreases and the entropy increases, keeping the total energy constant.
So you know, when you’re a kid and you hear, like, “Turn off the lights, save energy,” right?
Or “We’re having a brownout to save energy.”
And then you’re like, “Wait a minute, the first law says energy is always conserved.”
We don’t really mean save energy.
We mean save work potential, save availability.
So how does that work potential become the chaos that is entropy?
Well, it happens through real processes that we call irreversibilities.
That’s things like heat transfer, friction, chemical reaction, unrestrained expansion, electric current flow through a resistance like a wire.
All actual processes involve effects such as these listed, including naturally-occurring processes and ones involving devices we construct, from the simplest mechanisms to the largest industrial processes.
This is why we can’t ever reach 100% efficiency.
These real irreversibilities exist and this is why.
And it’s a bummer.
So going back to our fuel burning in a cup, during our fuel burning process, we converted the availability of fuel to chemical energy, and we turned that into the thermal energy which warmed the air.
That initial work potential of the fuel was destroyed by the irreversibilities of chemical reaction of combustion and the heat transfer from the combustion into the air.
Now, there are serious implications to this, right?
The implications are that real processes like power plants, or my beloved engines, operate at a lower efficiency than the maximum because of the irreversibilities that exist.
We want to try to minimize the existence of these irreversibilities and processes.
We reduce friction by using lubricating oils, for example.
But they will always be there.
We can never get rid of friction completely, right?
We need temperature gradient in order to do heat transfer.
We want to do heat transfer in order to make our power cycles go.
So we can’t avoid doing the things that are these irreversibilities, and we have to quantify them if we wanna avoid waste.
You can minimize these irreversibilities.
You can target them.
You can do things like add lubricating oils to reduce friction.
You can, you know, lower the temperature gradient in the case of a heat transfer to reduce that.
You can lower the resistance of a wire in order to help the electricity flow.
So it’s important that we have a way to quantify them.
The other implication is that we need a better standard than thermal efficiency to judge processes.
If work and heat are not equivalent, then how can we be judged on how much work we can provide from heat?
So we need to compare to an ideal case that still has real irreversibilities, but they’re minimized.
And you’ve seen that; you see that probably on some appliance you have at home.
That’s what these Energy Star ratings are.
They’re what’s called a second law efficiency.
So they’re comparing your real device to the best possible that that device could do, right?
In the Energy Star ratings, it’s compared against the most efficient water heater or whatever.
But second law efficiency says wait a minute, we know that energy is not equivalent.
We know that real things like friction exist, and therefore, the second law efficiency of any process is always gonna be higher than the first law efficiency of it because it’s sort of curving, right, based on reality, right?
We wouldn’t send a kindergartner in, you know, to take a college-level thermodynamics exam, right?
That doesn’t really make sense.
We would want to then curve the grades based on how the best kindergartner did, right?
We wanna compare apples to apples.
The other thing we need to do is complete a full assessment of processes in order to determine all of the lost work potential and the real efficiency.
And that means starting from the energy source to the end use, okay?
That’s life cycle assessment.
Life cycle assessment from an energy basis evaluates the total benefits and burdens over the entire life cycle for a product or process.
We often say from cradle to grave.
And what that means is that means from getting the energy resources, from mining the coal to processing it so it can be burned in the power plant to the use phase to transporting it, you know, that electricity to your house, to you using it in your coffee grinder, to the eventual disposal at any recycling that might happen.
And so those are more for manufacturing processes, right?
So you can think about if you’re gonna make a car, right?
You gotta go mine the ores for the, you know, metal body and then you have to process them and make it and then transport the car on one of those really scary car carrier trucks to your house.
And then at the end of the life of the car, after you’ve put your 250,000 miles on it, you know, how you recycle those parts.
So why is energy literacy so important?
Well, it’s so we can do that life cycle analysis, but the real reason is because this is the most important system.
We live in a system.
There’s energy coming into our system from the Sun, and everything we do is changing the work potential that exists within this system.
We have to understand energy forms and transfers and how to evaluate these things so that we use the resources, the finite resources within this system wisely and minimize the destruction of work potential and transformation into entropy.
You need to be able to evaluate information critically, right?
Okay, so now I have a test for you.
I saw this diagram on LinkedIn earlier this week, and what my question is for you is, is this a fair comparison?
So we’re going back to cars.
So here’s a diagram that I was shown on LinkedIn this week, and it says, “Electric vehicles are six times more energy efficient than gas vehicles.”
And they start with the electric vehicle and they talk about charging losses and power steering and cooling and electrical accessories and electric drive system losses.
And they say, “Well, that’s 87% to 91% efficient.”
And then they start with the gasoline vehicle, and they say, “Okay, same electrical accessories, “you have some idling losses, you have engine accessories, “you have your drive train friction, “and then your heat and other engine losses, a lot of it going out through the exhaust,” and they get to only, like, 16% to 25% efficient.
And by the way, I’m gonna show you in a minute, those numbers are terrible.
Those are way off.
But anyway, is this a fair comparison?
The answer is no.
One of these systems includes the energy source.
The car has gasoline in it.
Gasoline was an energy source that I showed you on that source slide.
The electric vehicle has a battery, it has a way to store energy, but that charge has to come from somewhere.
So you need the power plant or at least some electricity to be included in that as well.
So the conversion from the gasoline to the motive force, right?
That power, the mechanical work is taking place within the system.
The conversion of the energy source, which, by the way, in Wisconsin is still mostly coal, but we’re getting better on solar and other things.
Or from the nuclear power plant, whatever, is taking place outside the vehicle.
You are creating the energy outside the vehicle, right?
You are transferring that power through the electrical lines where you’re having an electrical energy transfer over a resistance.
So you’re losing some work potential there, right?
Then you are turning that electrical energy into chemical energy.
So you’re doing a transformation as you store that energy in the battery.
Anytime you transfer or transform, you’re destroying work potential.
Not always a lot, but you are.
And then when you pull that chemical energy back out of the stored battery, you are also using it.
So the engine serves the equivalent of the power plant, which is not included in this comparison.
Generation and transmission losses are also missing.
So here’s how I would say it ought to look.
We need to start with the wellhead for the car and go to the refinery, and then we have to transport that fuel to the gas pump where you’re going to put it into your car, and then you’re going to use it.
And then on the other side, you’re gonna go to the wellhead and get your natural gas, and you’re gonna go through power generation, and then you’re gonna go through transmission, and then you’re gonna go through your battery charger, and then you’re gonna use it in the car.
And the truth of the matter is the one pathway for the well to tank has about a 30%, 33% efficiency, and the other one has about an 83% efficiency.
And then in the use phase, the tank to wheel, then sure, the electric vehicle is about 80% efficient.
And then the combustion vehicle is only about, well, honestly, gasoline’s about 35% efficient.
These are a little bit old numbers.
But overall, ’cause efficiencies are multiplicative, that 30% times 80% gets you to 24%, and the 30% times 80% gets you to 24%, and they’re honestly not that far off when you compare the entire life cycle.
This is why we have to be careful and critical about what we read.
Here’s some more realistic engine numbers by the way.
So this is the plot from ExxonMobil.
I apologize for the colors at the top.
This was from 2005.
And if you don’t think engines have gotten more efficient in the past almost 20 years, you’re pretty dead wrong.
They have, but you can see at this time, the brake work was up around 35%, 37%.
That brake work is the dark gray box.
And you can see, it’s like around 35% there across the range of peak power.
But we get all the way back to 100% of the fuel energy because then we say okay, well, then mechanical losses, so that’s your friction that, you know, takes you up to about, you know, 38%.
Then you have your fluid flow losses from moving the, you know, air around or moving the gasoline around.
That takes us up to about 40% of the gasoline energy.
Then there’s a lot of waste in what we send out to the exhaust, to the ambient.
So that takes us up to about 65%.
Then we have the irreversibilities associated with the chemical reaction of combustion.
Now, if we account for that, we’re up to about 82% of the total fuel energy.
And then the last one in that green that’s a little illegible is cylinder heat losses.
And that’s how much the engine block is actually losing heat to the coolant.
Which you would think, “Oh, well, why do we need to throw away that energy?”
Mostly so your block doesn’t melt.
So you know, so what I often tell my students is what we need to do is we need to learn to identify where all the losses are.
We need to accept the things we cannot change.
We cannot change anything about the irreversibilities due to friction or chemical combustion.
And we probably really shouldn’t do anything about those cylinder losses ’cause we want the block to stay.
And so we have to accept the ones we cannot change, and we have to have the engineering fortitude to change the things we can.
Now, in the center there in that orange, the exhaust to ambient, we can do things like waste heat recovery.
We can run an electrical generator off the thermal energy that’s still available in the exhaust gas.
We’ve done that.
DOE has done that.
They do that as part of the super truck program, and they’ve now hit, like, 55% brake thermal efficiency for heavy duty trucks.
It more than doubled their mile per gallon rating for doing that.
So the question is, if we can, why don’t we?
And the answer is because it’s expensive, right?
So again, I think it’s a matter of understanding what’s there, the energy sources, the energy uses, the energy transfers, the energy forms, understanding that while energy is conserved, that’s total energy.
And the good part of energy, the availability, the work potential, is always decreasing, and it’s decreasing because of those irreversibilities that generate entropy.
And we can do a lot to minimize them, but we can never get rid of them, and we should use that knowledge to do really good matching of energy source to end use.
We should use that knowledge to minimize the availability destruction that we have, that loss of work potential, and minimize the entropy generated.
And we should use that knowledge to critically evaluate graphics or news stories or things that we see where people aren’t showing us the full picture.
So thank you so much.
I really appreciate all the people in the room and online.
Thank you so much.
I look forward to discussion and answering any questions.
My email address is on the slide there.
If you are wondering about the other talk I mentioned, you can find it through University Place, and I’m sure they’ve put the website on here.
I encourage you to get in touch with comments and questions.
Thank you very much.
[audience applauding]
Search University Place Episodes
Related Stories from PBS Wisconsin's Blog

Donate to sign up. Activate and sign in to Passport. It's that easy to help PBS Wisconsin serve your community through media that educates, inspires, and entertains.
Make your membership gift today
Only for new users: Activate Passport using your code or email address
Already a member?
Look up my account
Need some help? Go to FAQ or visit PBS Passport Help
Need help accessing PBS Wisconsin anywhere?
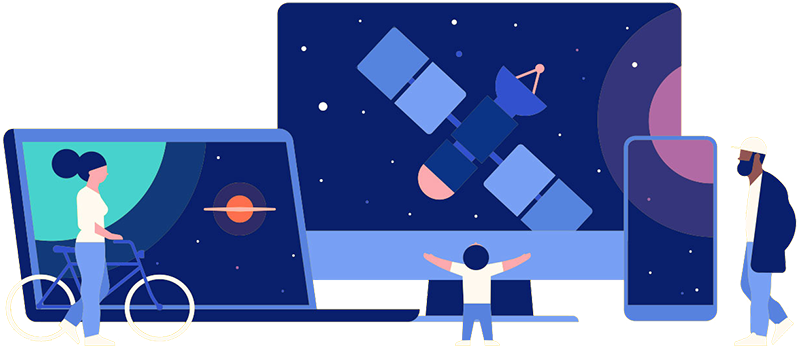
Online Access | Platform & Device Access | Cable or Satellite Access | Over-The-Air Access
Visit Access Guide
Need help accessing PBS Wisconsin anywhere?
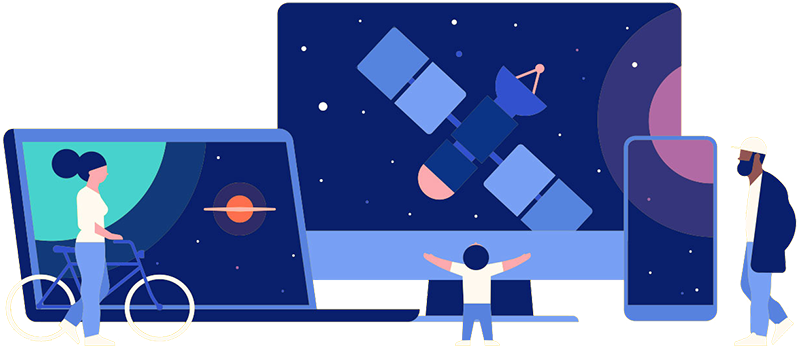
Visit Our
Live TV Access Guide
Online AccessPlatform & Device Access
Cable or Satellite Access
Over-The-Air Access
Visit Access Guide
Follow Us