[Alexis Hernandez Abrego, Outreach Specialist, Biotechnology Center, University of Wisconsin-Madison]
This week I would like to introduce Professor Jack Williams, who was born in Chicago, Illinois, and attended University High School in Sacramento, California, where he went – later went to pursue his bachelor’s in geology at Oberlin College, to finally obtain his PhD in geosciences at Brown University. He came to Madison in 2004 and is here today to talk about the ecological responses to climate change since the last Ice Age.
Please join me in welcoming Professor Williams to Wednesday Nite @ the Lab.
[applause]
[Jack Williams, Professor, Department of Geography, University of Wisconsin-Madison]
Great. Thank you all. It’s really a pleasure to be here.
I do have degrees in geology and geosciences, and if you’re paying attention, I’m – I’m now a professor in geography, which has confused – confused my family to no end.
[laughter]
They can never quite remember which geo am I on any given day. But the reason for that is, as you’ll see, that I really do a fairly interdisciplinary kind of work. I study species responses to climate change. So, ecology, climatology, using the geological record and using the sediments we can find in the many lakes and mires around us, as archives of past ecological and climatic history.
So, that opening slide there gives you a bit of a sort of taste of the scales that we’re going to be working on. Looking from the last Ice Age –
[slide titled, Ecological Responses to Climate Changes Since the Last Ice Age, featuring Professor Williams bona fides and four illustrations of ice coverage of North America at various points since the last Ice Age – 21,000, 15,000,13,000 and 1,000 years ago]
– 21,000 years ago, the – the last glacial maximum we call it, where we had ice sheets sitting over North America. Right here where we stand was under ice. And then those ice sheets retreat, and, as temperatures rise, as CO2 levels, carbon dioxide levels in the atmosphere rise, various species move north. And so, one of the things that we use as time period quite a bit for is studying ecological dynamics in a warming world, which has obvious comparisons to what’s happening today.
[Jack Williams]
So, sort of a four-part, four-chapter structure here. Give a bit of an intro first here –
[slide titled, Chapters, with an outline of the talk – Part one – Introduction, Part two – From Mud to Models – Using the Past to prepare for the Future, Part three – What have we learned? Six Lessons from the Past and Part four – What should we do?}
– then move into understanding a bit more about how we use the past to gain insight into ecological dynamics and make preparations for what’s happening now and is expected to happen in the future, give an overview of some of the key lessons we’ve learned along the way, and then finish a bit on – on this crossover from paleoecology, which is the field of study that I’m representing here, to conservation biology, how do we make informed management decisions given this kind of information from the geological record?
[new slide titled, Ecological Risks Posed by Climate Change featuring photos of a polar bear and an American pika along with the following bulleted list. Loss of existing habitats – top of the mountain problem, Fast rates of change – how closely can species track rapid climate change?, Tipping points and tipping elements – where are the non-linear responses and Novelty – bottom of the mountain problem – how well can we predict species changes to novel environments]
So, to start with and to give an overview of if – if you think, again, sort of this context of a changing world, rising temperatures, rising carbon dioxide concentrations. Carbon dioxide now at 400 parts per million, which we haven’t seen since the Pliocene –
[Jack Williams]
– and it’s still going up. So, if we then think about the fact that we expect temperatures to rise and other kinds of climatic changes to go with that, we then start to ask how we best conserve species of concern, biodiversity, ecosystem services, all kinds of things that you might want to adapt and manage under these changing climates.
And, in ecology, we can think of some general areas of risk that might come with those changing climates.
[return to the Ecological Risks slide]
So, the first one is habitat loss, that if there are species that are endemic to, dependent upon, the best competitors in, highest rates in population and transit growth, other certain climate regimes and those climates disappear, then we’d expect those species to maybe shrink in population size, perhaps even be at risk of extinction. Sometimes this is called “the top of the mountain” problem or “the polar bear problem” because polar bears are very charismatic species that are dependent upon arctic sea ice as a key habitat, and as that –
[Jack Williams]
– arctic sea ice melts back, there is concern about polar bears having their habitat diminished. No one actually thinks, as far as I can tell, that polar bears will go extinct, but we do think that they’ll have reduced population sizes and so forth.
Then we have fast rates of change. How, if climates change quickly, if there are rapid rates –
[return to the Ecological Risks slide with the second point – Fast Rates of Change highlighted and the photos of the polar bear and American pika animating to a graph of various plants and animals on Earth plotted by the maximum speed at which they can move in kilometer per decade]
– of temperature rise over the coming decades, and the scenarios being, say, two to four degrees Celsius temperature rise over this century, that’s pretty fast, the question becomes, how quickly can species keep up? And so there, at the far right, as – as you can see here, is some different estimates of rates of, let’s see, if I look at my cursor there, so we have these different bars here representing the rate of dispersal ability – how – or mobility of different species. How far can they move in sort of typical, sort of, populations, kilometers per decade? Some species can move quite far. The large animals have high mobility. Some species, the trees, can’t move at all. They have to throw propagules out and reseed and move that way. So, they don’t move as fast. And then the – the various lines there represent spatial rates of climate change. Converting these estimates of temperature change in any given area to how far will thermal zones move laterally. If you’re a tree, if you plant trees, you may look at Arbor Day and their maps of plant hardiness maps –
[Jack Williams]
– those plant hardiness zones are expected to shift as temperatures rise and species will need to move to keep up with those shifts.
There’s also the question of rapid and abrupt changes. So, the first point to there was fast climate drivers and can species keep up? There’s also the question –
[return to the Ecological Risks slide with the third point – Tipping Points and tipping elements – highlighted and the graph animating to two illustrations, one with a map of the Earth with population densities of the continents noted and one of a series of balls rolling on green hilly plains with the hilliness of each plain varying by the amounts of perturbations of the environment]
– of fast ecological responses. Will species respond non-linearly? Where are the tipping points? And so, you know, this idea that there might be multiple stable states represented conceptually by those balls there. And that you might have one stable state, like a grassland, and then you might push a system hard enough that it flips another state, like a forest. So, there can be, sort of, back and forth between these states. Down in the lower right there, we have different parts of the world. That – that figure looks a little washed out from here, but that’s a map of the world. And those colored areas are different regions of the world where we have observed system dynamics in the past of these sort of abrupt shifts. The Saharan Desert in Africa, North Africa, shifting pretty quickly back and forth, doing a grassland state and a desert state. The North Atlantic circulation flipping on and flipping off, speeding up and slowing down. There are some parts of the Earth’s system that seem to show this kind of bistability, these quick flips in state.
[the Ecological Risk slide animates to the last point – Novelty – being highlighted and the previous world map and balls and plains animating to illustrations, one of a pair of colored pyramids representing thermal zones flipping as the bottom of the mountain changes and one with a map of the world with novel climates highlighted in red, orange and yellow]
And then the part that I have actually worked the most on, and I’ll develop – someday I’ll develop all these a bit more as we go forward, but the part that I’ve worked on the most, and will develop the most here, is this novelty challenge, sometimes called “the bottom of the mountain problem”, where, at the top of the mountain, if you sort of look at that cartoon there, that cartoon is supposed to represent, sort of, thermal zones, cold blue, so cold at the top, warm is at the bottom, and in a warming world those thermal zones move up-slope, that those coldest environments at the top move off the top of the mountain and disappear, which is bad news for those species. But the bottom of the mountain has warmer than present conditions than has ever been seen at that mountain before. So, now there’s new climates that have not been seen at that location before, and the question becomes, how will species behave or respond to those warmer than anything seen at present conditions? Or – I’m focusing on temperature here – but there might be other dimensions of climate. More extreme precipitation regime events and so forth.
This is called novelty, and there’s a map at right there, sort of mapping out globally, areas of high climate novelty. This is a paper we did about 10 years ago now. And we did a sort of analysis of 21st century climates by the end of this century versus –
[Jack Williams]
– climates at the beginning of the century and identified places where the climates of the future had no close counterpart in the climates of today and they tend to occur in the tropics and subtropics. The warmer world and those parts being at the leading edge of a warming world becomes a question of how well can we predict how species respond in these – anything that’s – to climates that are warmer than anything we see at present?
So that’s the set up. And these are all kinds of questions that are – that are ecological questions today and, not coincidentally, they are ones that can be addressed by looking at the past. And that’s a big part of what I do in my work is trying to work with geological data and the geological record of past climatic and ecological events and draw parallels and insights into what’s happening today.
So, in this picture here –
[slide titled, Model Systems for a No-Analog Future, featuring a graph with years of the Holocene plotted on the x axis with years before the present demarcated with a minus sigh and years in the future demarcated with a plus sign and temperature change in Celsius plotted on the y axis. The challenge is how to predict species responses to rates and states of the global climate-carbon system with no recent analog]
– what we’re seeing now is changes in temperature, on – on the vertical axis, and this is expressed as relative to the late 20th century, like 1960s, 1990s or so. And positive values will be warmer than that reference point. Negative values will be cooler than that reference point. And the time scale is a little tricky here. It actually changes its scaling, and this line here, if I get my cursor back, represents today. This is looking out 100 years in the future. This is looking back over the last 150 years. So, this would be back to about 1860 or so. This is basically the domain for which we have weather station data, instrumental data, direct observations of the climate system and its changes through time.
And what we see is that there’s been a rising temp change – trend in temperatures with some little stair steps along the way, and we expect these trends to continue. And this is being driven by the rising greenhouse gases from our fossil fuel and land use and land cover change through the emissions of greenhouse gases, carbon dioxide, methane, and nitrous oxide, primarily.
We go further back in time, we can see there’s been some variations back here, but you can see that over this time scale we’ve had a range of variation of, you know, plus or minus a degree or so but nothing like what we expect to see over the next century. And this is now going back to about 10,000 years ago. So, this is now back to 2,000. This scale is changing here and back to about 10,000 years ago.
[slide continuing the theme of Model Systems for a No-Analog Future now with the four ways of tracking change numbered with a corresponding photo or illustration. First is Field and Lab Experiments featuring a phot of a field experiment in a forest. Second is Earth System Models featuring an illustration of a grid system of measuring Earth temperature. Third is Modern Analogs featuring a photo seismic activity in the ocean. And finally, fourth are Geological Analogs featuring an illustration of ice coverage on the Earth in the last Ice Age]
So, this is a – a common challenge we face. And we – and I – I’m focusing on ecology, but you could sort of extend this challenge to any area of the world. How do economic systems behave? How will flood regimes in the greater Milwaukee area behave? This general challenge, we’re moving the system to a state that’s not like anything we’ve seen in recorded human history and how do we best adapt and plan?
So, in this challenge, we have sort of, if you’re a scientist trying to tackle this, you would – I think it would be fair to say you have four general classes of tools. You can do experimental manipulations, and this – these are from the Department of Energy’s Free Air Carbon Dioxide Enrichment experiments, the F.A.C.E. experiments, where they built towers around forest plots and agricultural plots and grassland plots, pumped CO2 in those areas, and watched how the forest or other ecosystems behaved under an enriched, ambient CO2 levels. There’s other kinds, you can do warming experiments and so forth.
We have numerical models and computational models that model the atmosphere and the oceans and the other parts – the cryosphere and the ice sheets, how they behave under rising temperatures or other changes of rising greenhouse gases and forcing the climate system. You can go to modern analog systems, such as deep-sea vents there. People are using deep sea vents to study ocean acidification because there’s a lot of those, that’s very acidic water in the vicinity of those deep-sea vents. So, if you want to know how deep ocean communities will behave under rising CO2 levels and the rising CO2 concentrations that will permeate into the oceans, that becomes a system you can study.
And then the part that, again, that I’ll develop further here is going to the past and looking for geological time periods that have some counterpart to today.
[Jack Williams]
So, to take that same diagram now, and that’s the same one I showed two slides ago, and now expand it out. So, now we saw –
[return to the previous slide with the graph of temperature change over years now expanded to cover more time – adding the Pliocene and Pleistocene geographical ages to the Holocene and Future]
– the last 10,000 years, which is here, and now I’ve extended out to the last – to the last five million years. And so, this is going back to 500,000 years ago and back to five million years ago. And now you can see when you sort of zoom out a bit further, there’s all kinds of climatic events happening that have some interesting parallels and are – are good model systems for what’s happening now.
And some of the key features you can see is that you can see there’s a slow drift of the Earth’s system towards a cooler state over this sort of five million time period. And then you can see that over the last 500,000 years there’s been a very pronounced glacial/interglacial cycle, and I’ll turn back to this in a little bit, but we have these, you know, warm periods, cold periods, warm periods, cold periods, and these are pretty abrupt transitions. And I’ll zoom in to show you that some of these transitions are similar in rate and magnitude to what’s expected over here.
And so, obviously this is a cooler world than we’re expecting to have in the future, but the rate of change and the amount of change is comparable from those time – from those time periods to what’s expected this century.
[new side titled, Periodic Glacial-Interglacial Cycles, Paced by Variations in the Earth’s Orbit, featuring a graph of temperature change during the glacial-interglacial time period as well as a diagram of the Earth’s orbit around the Sun and two maps of the Earth – one of ice coverage during the last Ice Age and one of Earth Modern Day]
So, now to go into that just a bit more here. So, now to kind of go from that framing to actually talking a bit about these geological time periods. This is another version of this data here.
[return to the previous slide graphing temperature change by geological age and back to the above slide]
This is what’s confusing and annoying about my field –
[return to the temperature change by geographical age slide]
– is that we don’t – we aren’t consistent about which way time goes. Time is running from left to right in this plot.
[return to the glacial-interglacial slide]
Now it’s running from right to left. So that’s older over there, and it’s younger over here. So, that’s 1.8 million years ago, at far right, to today. We also, being geologists, we count back from the present, so that’s zero years ago at left and 1.8 million years ago at far right. So, what you see when you look at this is that there is this very regular climate variation. This is one of the really cool things that got me excited about paleoclimatology when I was a grad student and starting to learn about it, is that there’s this very regular oscillation in the Earth’s system between what we call interglacials, the warmer periods with smaller ice sheets, and the glacials, the colder periods with larger ice sheets, and you can see it far lower right there. The ice sheets covering North America during a glacial period in parts of Eurasia as well, and then the interglacial, like the one we’re in right now, with ice sheets restricted to Greenland and – and Antarctica.
So, we have this regular oscillation, and if you actually were to do a – a periodicity analysis, you would find out that there’s a 40,000-year beat back before a million years ago, and it shifts to about 100,000-year beat. Those big cycles are happening 100,000 years ago or every 100,000 years. And then they shift to a higher frequency back before. Why it goes from 40,000 years ago to 100,000 years ago –
[Jack Williams]
– is an area that people are still working on, but we know, in general, that all that periodicity is being controlled by variations in the Earth’s orbit. That the orbit varies at these timescales. It changes from being more circular to more elliptical. That’s what that diagram there shows. The tilt of the Earth changes from being more closer to perpendicular to further away from perpendicular. We wobble like a top wobbles. And those three components of motion, the eccentricity, the tilt, and the wobble, which we call procession, affect the amount of sunlight we get at different latitudes. That changing the amount of sunlight we get at different latitudes and seasons directly controls how – the growth and melting of those ice sheets and other parts of the climate system as well. So, we have a very good understanding of this orbital, what we call the orbital variations –
[return to the slide featuring the glacial-interglacial graph and illustration of the Earth’s orbit]
– the orbital forcing of these ice ages and their waxing and waning.
[new slide titled, The Last Deglaciation – Key Features, featuring a map of the largest extent of the ice in the last Ice Age in North America as well as a graph of temperature change from present by geological age – Pleistocene/Holocene. Additionally, there is a bullet pointed list noting that during this time the global temperature rose by 4 to 5 degrees Celsius, regionally there were abrupt temperature rises of 9 to 14 degrees Celsius in 1 to 40 years, the ice sheets melted, the sea level rose by 120 meters and novel climates emerged]
Okay, but this isn’t a paleoclimatology talk. This is an ecology and biogeography talk. So, this is really the structure that –
[return to the glacial-interglacial temperature change slide]
– as an ecologist, we’re working in. What we’re interested in less is what’s causing these climate variations, but how do species and ecosystems respond to them and survive them because if you’re a species that’s been around for any more any more than a few hundred thousand years, you’ve had to live through multiple glacial and interglacial cycles and you’ve had to be able to adapt to and accommodate –
[Jack Williams]
– this kind of climate change in the past.
So, now what I’m going to do is zoom in into just one of these events. That’s the best study of them all because have lots of of lakes and mires and radiocarbon data of sediments that cover it. And so, this is the last deglaciation. This is the last transition from the last glacial maximum to the current interglacial, what we call the Holocene.
[return to The Last Deglaciation slide]
So. now we’re looking, time is now going that way again. Oldest, 22,000 years ago, to present at far right. Timescale or vertical scale again is temperature as difference from present. So, this is a record from Greenland. So, this is telling us that Greenland was about 10 degrees colder than present about 20,000 years ago. And that about 10,000 years ago, it reached more or less modern day or pre-Industrial Revolution temperatures, and it’s been more or less constant there ever since.
And what you can see is, of course, you can notice very quickly that it’s not just a little linear ramp up. There’s some very abrupt changes along the way. There’s some distinct events, and we have given them names. We call the – that first abrupt warming the Bolling warming as part of a broader event called the Bolling-Allered. And then there’s that Younger Dryas, which is that return to sort of near glacial conditions. And then the abrupt end of the Younger Dryas, which is the start of the Holocene, the start of the current interglacial.
So, lots of things are happening climatically. Globally, temperatures rise by about four to five degrees Celsius. Some of those changes aren’t what we would, you know – sometimes you hear geologists say, “Oh, things happened really fast in the past.” And people say, “Oh, yeah, you’re a geologist, you’re talking about millions of years, that’s not meaningful.” These are really abrupt, even on human timescales. People think basically it’s Greenland and other high-resolution records that some of these abrupt shifts in the climate of these areas could have been nine to 14 degrees Celsius in one to 40 years. In our own lifetimes would have been sort of the comparable kind of rate of changes. So, these are big past climatic events. These aren’t global; these are North Atlantic, western Europe. But in these areas, at least some regions, we’ve had very rapid changes.
Ice sheets melt back over this time period. That plotted upper right is showing this, I think, this really beautiful diagram of the Laurentide Ice Sheet and the Cordilleran Ice Sheets. The dark blue is its furthest extent, and then it’s melting back over this sort of from about 19,000 years ago to about 6,000 years ago. The ice sheets are wasting away as temperatures rise. Sea level rises because that water has got to go somewhere, and it winds up in the world’s oceans. So, that’s about 400 feet or so of sea level rise from the last glacial max to the present. And then –
[The lower half of The Last Deglaciation slide animates off the temperature to geological age graph and the previous illustration of the Earth’s rotation on its axis over time and a new graph plotting summer temperature vs. winter temperature in North America for three different time periods, 15,000 years ago, the 20th century and a future date of 2050, animate on]
– novel climates emerge. And what I mean here by novel climates is that if you actually look at the ranges of climates that occurred at some points in the past to the ranges of climates that happened today, there are areas – areas of non-overlap. And the way to, kind of, show that visually is at that far right plot there, where what we’re looking at here is just two simple temperature axes – summer temperatures and winter temperatures. And we’re looking at the- the – the – the – each location that represents some location in North America for three different time periods. Red, being a projected foward set of climate model simulations for what we think North American climates might look like maybe 30 or 40 years from now. Black would be the late 20th century, the recent past. And then blue is 15,000 years ago.
And what you can see is that there’s areas of non-overlap. I don’t know how well you can see it from – from your angle, but there’s an area over here, for example –
[uses mouse pointer to point out an area of red on the summer/winter plot that does not overlap the other two colors – showing an increase in temperature]
– whereas we move into a warming world, portions of climate space open up in North America. These novel climates that aren’t available to species in North America today, presumably mostly in the southern parts of North America, whereas, 15,000 years ago –
[uses mouse pointer to point out a non-overlapping blue area on the plot where temperatures where cooler]
– there’s a whole other portion of climate space that opens up back then. This portion of climate space is – is more seasonal. We have, kind of, warmer than present or similar to present summer temperatures, and colder than present winters. And the reason for why we have this sort of portion of climate space open in the past that’s not open today is because of these changes in the Earth’s orbit and the way that affects temperature seasonality. It actually has different effects on summertime temperatures and insulation, incoming solar radiation, and wintertime values. So, that opens up portions of climate space that species had available to them 15,000 years ago –
[Jack Williams]
– that they don’t have today.
Okay.
So, that was all, sort of, setting the stage and setting the context here. Now to go into, okay, now we have, kind of, some understanding of these times and places, let’s go in and how do we, sort of, study these time periods.
So, this is, kind of, a starting point for the – the – that data we gather. We go out in the field, and we get muddy.
[slide titled, Methods – From Mud to Maps, featuring two photos on of Jack in the field in muddy clothes and one of a picture of a mire wherein the studying is taking place]
We – we go out into middles of mires, we go to – we set up floating platforms on lakes, and we take long tubes of mud from those sediments.
[new slide titled, Coring Horseshoe Lake, IL, featuring a photo of students and researches using long tubes to collect mud from the sediments in Illinois]
Here’s a – a cleaner looking team actually out on a lake here. And this is from Horseshoe Lake just outside of St. Louis by Cahokia, if you’ve ever been down to Cahokia, we’re working right outside of Cahokia there and getting a record of environmental and vegetation history from Cahokia. And so, you can see that ba – that maybe there’s a couple steel tubes there, but it’s about a meter long steel collection barrel that we drive down, a drive at a time, bring back a meter of mud at a time. And as long as that lake has been around, it’s been collecting mud, and so we can take those – those –
[new slide tilted, Extruding Core, with a photo of students and researchers on a floating platform on a lake pulling the mud and sediment out of the long tubes]
– those – sample – those cores of mud back to the lab and analyze them. So, now we’re extruding that – that core of – of – that – that long tube of mud. We’re going to wrap it in those – we’re going to first wrap it in – in cling wrap, and we’re going to wrap it in those PVC. It’s got, kind of, a consistency of, kind of, –
[Jack Williams]
– a banana at the top, down to a pencil eraser at the bottom as the bottom sediments get more and more compressed and dewatered by the weight of the above sediments. So, it has some cohesion and – and stability to it, but we wrap it up and protect it and take it back to the lab.
So, there we can – here’s another one where, before we wrapped it, just getting a quick photo of it.
[slide titled, Mud!, featuring a photo of a researching holding a core of mud that has been extracted from a tube]
And you can just see that some of the sedimentological variations. This is a lake that has dried out, obviously, at some point. So, you can see how the uppermost sediments at left have become oxidized, they’ve been exposed to oxygen. They’re getting kind of rusty and iron rich. Whereas the blueish sediments at right, that’s reduced iron that hasn’t been exposed to oxygen yet. So, just as a first pass, visually we can start to get some in-insights into the environmental history of that lake.
[new slide titled, Lakes and fossil pollen used to study past vegetation, featuring a small photo of students and researchers coring a lake at Silver Pond, Ohio, a diagram of how pollen is transferred from trees and vegetation into the sediment of the lake, four photos of four different sample pollen grains, chestnut, chenopod, oak and pine, and a photo of a one meter sediment core section from Anderson Pond in Tennessee]
And then the, kind of, what our field works with are what we call proxies. What we want to know about our past temperatures, where do species live, past water pH, but we don’t have thermometers. We don’t have species traps or – or photo cameras. What we have are proxies for these things. So, we use pollen as a proxy for the past plants. We use various stable isotopes, like oxygen, as a proxy for water balance or temperature and so forth.
And different labs work in different proxies. Ancient D.N.A. increasingly use a proxy as well. And so, my lab does pollen analysis. We’re actually celebrating the 100th anniversary of pollen – this is a birthday centennial year. Pollen analysis was invented, or didn’t invent it, but initiated –
[Jack Williams]
– by Von Post, a Swede, in 1917. So, this has been around for a while. But it – because it’s been around for a while, it’s a mature proxy, and it’s one that we’ve got a lot of data on over the last century. So, we can now work with large proxy networks of different records from different places where people have been doing this kind of work for a long time.
So, the way it works is that plants produce pollen. Some plants –
[return to the Lakes and fossil pollen slide]
– are relying upon wind dispersal, so they throw that pollen up in the atmosphere. And they hope that by chance some of that pollen they throw up there will land on a female flower and perpetuate the species. It’s a very inefficient way of doing that, but pollen is cheap and so they – it’s that – it’s that – they do this sort of broadcast approach. Some of that pollen settles on the lake, settles down through the water column, is captured at the mud at that bottom, and then, as that mud accumulates up, it – the older sediments are at the bottom, they have pollen from when that lake formed 15,000 years ago or whenever, and the uppermost sediments are the most recent sediments deposited in the last few years.
We core it, we bring the mud back, and then, under light microscopes and sometimes S.E.M.s, scanning electron microscopes, we identify the pollen to genus, sometimes to species, sometimes to family. And here’s just some different photos for different – different pollen grains as you’d see them –
[Jack Williams]
– under a light microscope.
So, kind of just one quick example of this kind of work. So, here’s one site in northwestern Indiana where we have a beach.
[slide titled, Example – Spicer Lake and American Beech, featuring a map of the Midwest with sample sites demarcated as red dots with an arrow pointing at a red dot of the sample site and two photos of the types of vegetation in one particular area in Indiana]
[new slide titled, 15,000 years of climate-driven vegetation change in Northern Indiana, featuring a graph with the various vegetation of Indiana plotted on the x-axis and the frequencies of the pollen spores for this vegetation plotted on the y-axis showing change over time of the frequency of each type of vegetation]
And here’s one lake sediment record. And what we produced is called a pollen diagram recording, from those lake sediments, all the different pollen that we find from the different zones. It tells us something about forest history and change in this site.
Not only do our – does my field go left to right versus right to left, we also go up and down. So, now time is on a vertical axis here. Oldest at the bottom, youngest at the top. And that actually makes some sense because that’s the way the sediments are deposited. The oldest sediments are at the bottom, the youngest sediments are at the top. So, this is kind of how a geologist often sees these things.
So, each curve, then, represents the relative abundance, usually what we do for any given horizon we might count 300 or 400 pollen grains, identify them to these different tree taxa, and then we say, “What proportion of those we counted were fir or spruce?” And so, what we can see is each curve here represents a different taxa. We can see that in northern Indiana, 15 to maybe 12,000 years ago, there’s a lot of spruce around there and a smaller amount of birch, I’m sorry, fir. And a little bit later pine comes in. So, we can see boreal forests. The kind of forests that we find in southern – in northern – more northern Wisconsin, southern Canada. Those forests were occupying northern Indiana 15 to 12,000 years ago. They disappear, pine comes in, we get sort of a pine zone for about 1,000 years. Pine disappears, you get oak, that big, green curve at near far right. Oak comes in, we get these oak dominated forests. You can see that beech curve; it’s flagged with beech up there. Beech comes in around 7,000 years ago. So, we can see these, sort of, long-term changes in forest history that are being very directly driven by climate change, that as –
[Jack Williams]
– temperatures rise, the boreal forests die out. They – those species effectively migrate north, and then other species, like oak, migrate in and become more abundant. And then, a little bit later, sometimes species take a little while to get there, beech comes in and is establishing by what we call the mid-Holocene about 7,000 years ago.
So, that’s just one site. And, of course, one site gives you a lot of information about temporal history at one location. It’s also a lot of work. You know, someone’s got to sit there at the scope and count these pollen grains. And so, what our community has moved to is creating community, what we call community curated data repositories. And the one that – Oops.
Hold on.
Mozy’s nagging me. Sorry.
Okay.
[slide titled, Many sites – Maps of continental-scale climate driven range shifts, featuring an illustrated map of North America with the types of geological data represented by various colored dots on the map]
So, what we’ve built are databases to house our data so we can move from, sort of, single point temporal analyses to multi-point spatiotemporal analyses to look at range shifts and range migrations. This is a resource at Neotoma Paleontology Database. The U.R.L. is there. It’s one that I’m one of the P.I.s involved in building this and – and helping build both the infrastructure and then the contributing of data to it.
And so, what this lets us do then –
[the lower half of the Many Sites slide animates off the dot map of North America and replaces it with a map of the distribution of spruce trees 20,000 years ago showing the last ice sheet and most spruce trees in the Ohio valley at that time period and then animating to more recent geological time periods showing the receding of the ice sheet and the migration of spruce trees to a more northerly range of today]
– are things like this, where now what we’re looking at here is an animated map of North America. This is an earlier visualization that goes back to a paper published in 2004. We’re working on creating some updated versions of this, but it’s still a great visualization so I still use it a lot for – for – for talks. And what we’re looking at here is every frame is a thousand years. We’re stepping forward a thousand years in time. And so, the first thing to pay attention to is you can watch the ice sheets retreat. You can watch the ice-free corridor open up between the Cordilleran – the Cordilleran and Laurentide Ice Sheets. You can watch Florida shrink because as sea level rises, areas with low relief, like the eastern seaboard, lose the most. So, Florida is quite large and loses a lot of land area between the last glacial maximum and present. The west coast not so much happens.
So, you watch sea level rise and ice sheets melt, but then the main thing that – that this is supposed to show here is the forest dynamics and showing how spruce migrates north. It used to be, spruce used to be growing south of us 20,000 years ago, and its northern range was right around here. And then as temperatures rise, the southern spruce populations die out, the northern spruce populations expand into new areas, and the whole population moves northwards.
This is some of our best evidence. And when we talk about –
[Jack Williams]
– species migrating and moving in response to climate change, it’s this kind of data that that concept is founded upon. Now, of course, we’re starting to see species shift their ranges in response to current climate change. That’s happening around us now. But the best documented data we have for how species respond to these sorts of long and large climate changes come from this kind of data.
So, okay, everything I’ve been showing you has been very data centric. Out in the field working with the mud, in the lab counting the pollen, making maps. Straight up from the data but, of course, in the sciences you really work from two directions. You have the empirical lines of evidence and then modeling your theoretical lines of evidence, and you’ve got sort of the intersection between the two, which is where the interesting science happens.
And on the modeling side –
[slide titled, We can use paleodata to test, improve ecological forecasting models, featuring three illustrations of the way species distribution models work, with data collection of species field observations and environment a.i. G.I.S. maps, statistical and dynamic modeling using ecological niche modelling and spatial predictions using predicted species distributions]
– a very common tool, of course there’s all kinds of different ecological models out there, a very common one that gets used all the time is what’s called species distribution models, S.D.M.s. They’re called environmental niche models. And what you basically do is you take some set of occurrences where a species is found out in – out in, you know, out in the environment, you then overlay that set of occurrences with the environmental characteristics, the topography, the temperature, precipitation and other variables, and you build a predictive model that predicts the species occurrence or abundance as a function of environment, what we call a response curve, the response of a species to that environment, and then you say, “Well, what if the environments were to change?” What if – what if we have a global warming or some other degree of climate change using a climate model to give us that scenario? And then you say, “Well, this is where we think a species would be in the future.” So, we use this sort of approach all the time to predict how species will respond. Where should we conserve habitat? What’s the risk of extinction using these kinds of modeling approaches?
[new slide titled, S.D.M.s (and other approaches) used to predict biodiversity responses to climate change, featuring a graph of pre-industrial temperature rise plotted against percent of predicted extinction base on current rates versus targeted rates]
And just as one example, this is just one of many papers, this is just one synthesis paper coming with estimates of how many species do we think will go extinct over the coming century in response to the different climate scenarios. So, the X axis is how much temperature rise will there be relative to, sort of, 1800s, pre-industrial baseline. And then what percent of species will be predicted to go extinct. And this is a meta-analysis of many different studies, and they all predict some degree of extinction, some just a few percent, some quite alarmingly, you know, 20% to 40%, but there’s a fair cloud there. And then some, the – the red line being some fitted curve to that. And a general sense that the more we have temperature change, the more we stress species, the more we change environments around them, the higher the rates of extinction. And those S.D.M.s I showed you are part of the backbone that predict these kinds of responses.
[new slide titled, Hindcast experiments with S.D.M.s, featuring three illustrated maps of the extent of oak distribution from 15,000 years ago to the current observed distribution of oak to the modeled future distribution of oak]
And so, one thing – of course, the challenge with this is that –
[return to the previous S.D.M. graph on extinction]
– we can’t test these predictions, right? We – we – or we have to wait 90 years to see how many species have gone extinct by the end of the century, you know, taking no action in the meantime. That’s not a very satisfactory approach to doing things.
[return to the Hindcast slide]
So, what we can do instead of a model testing ground is what we call hindcasting. We can take these models that are usually run from the present into the future and instead run them from the present back to the past and see how well they do there.
So, this is one set of analyses where we actually – we actually ran it forward, but we ran it forward starting from 15,000 years ago. We said, “Okay, let’s take this model, start it from 15,000 years ago, before the last deglaciation, before the climate changes happen, and then say, okay, how well will that model predict where that species would be today?” And so, here’s the distribution of oak at lower left. The colors are kind of ugly, but the greens are areas of high oak abundances. Oak was generally further south in Florida and so forth, southeastern U.S. And then, as temperatures rise, it expands northward.
The lower right – sorry, yeah, the lower right is what’s been modeled for its expansion. The upper right is what we observe from the – the – the – the modern botanical and – and pollen data. And we get a pretty good fit between the two. So, this actually a case where the hindcasting works, where this species distribution model is able to predict a northward range expansion of this set of taxa of of this genus in response to climate change.
[new slide where the three maps of oak distribution animate off and are replaced by a graph titled, S.D.M. predictive skill for oak, that plots the time window used to calibrate S.D.M. on the x-axis and the correlation of simulated versus observed on the y-axis showing decreased predictability over time]
What we can also find out, though, is that, you know, if we actually do an analysis of how good was that fit, the correlation between what was modeled and what was observed, and we say how far back do we start the model and then predict it forward, we find that the correlation is pretty good if there’s not much time separation. If we if we take climates from 2,000 years ago and predict to present, we get a pretty good match. And if we go back to, say, climates from 14,000 years ago and then ask the model to predict to present, we’re not getting down to a correlation of 0. 5 or so. So, there is a sense that model performance degrades the further you go back in time, the more climates change between your starting point and your end point.
[return to the Chapters slide with Part 3 What have we learned? Six lessons from the Past highlighted]
Okay, so that’s thats, kind of, going now from the modeling side. And I’ll come back to that with a few slides at the end or near the end.
So, okay, from this kind of work, what have we learned?
[new slide titled, Six Lesson from the Past, showing these six observations species are sensitive to climate change with a mixture of fast and slow responses, when climates change, plants move, species respond individualistically, climate-driven extinctions are rare in the recent past except for large animals also affected by human pressures, novel environments produce novel ecosystems and ecological forecasting models have a tough time predicting into novel climates]
This is kind of – of, I’ll go through each of these in turn here. But talking about, sort of, we can see that species are clearly sensitive to climate change. We can see that species move in response to climate climate change. They do so individualistically. And we also see that species that we don’t have much evidence of climate driven extinctions except for the large animals, the mammoths and the – the other large animals that are probably being affected by a combination of climate and human action. We see this emergence of novel ecosystems in response to those novel climates and, as I’ll come back to, this challenge of ecological forecasting.
[Jack Williams]
Okay, so now I’m going to go through each of those in turn with a few slides.
So, this question about rates of response, but this really matters, you know. How – if if temperatures are going to rise by several degrees over this century, how quickly can species adapt? Should we be moving species and – through what’s managed called a system migration or managed relocation? Should we sit back and let them adapt on their own? Let nature takes its course or some set of other options?
And so, one thing we can see from the from the rec from the Paleo data
[slide titled, Species are sensitive to climate change, mixture of fast and slow responses, featuring a graph showing temperature change on the x-axis and ice core ages on the y-axis for various vegetations showing their various responses to climate change]
– is that when climates change, you very clearly get strong local responses and often very strong fast responses.
And this plot here, again time being vertical now, oldest at the bottom, this record here is a temperature record. So, this – this is going back. Remember that Greenland ice core and those fast changes we saw around the Bolling-Allerod and the Younger Dryas? This is one of those events. So, there’s a very fast warming here in Greenland about nine to 14 degrees Celsius in a few years. Probably not quite as big a warming as at this location. But what you can see is temperatures rise and almost instantly, from a geologist’s perspective, the communities turn over. They go from a sage, sedge plant community, kind of an open tundra, to a juniper woodland within the span of a few decades. So, almost within, you know – very quickly this plant community is changing and turning over response to that that temperature forcing that it receives.
But several centuries later, pine shows up. So, there’s both what we call the fast responses of sort of local community turnover, and then the slower responses, where pine probably was not anywhere near this site when these temperatures changed. It was probably hiding out in various refugia down in the Alps and had to expand northward to this location. And trees don’t get up and move, so it took a few centuries too for – for pine to get there. So, we can see that there – this is an example of what we call a delayed response or a lagged response, where it takes a few centuries for the for the full, sort of, ecological effect of that rising temperature to be felt at this site.
[Jack Williams]
We’ve already walked through this point, the idea that that was at one site and then we can, sort of, step back and look at the continent and see that species accommodate climate change by moving. They they try to stay within their thermal zones of their climatic optima or ranges of tolerance, and one way they do so is by moving spatially
[slide titled, When climates change, species move, featuring the earlier map of North America showing the movement of spruce north as the last ice sheet recedes]
– as the environments change around them.
[new slide titled, Species respond individualistically, showing eight maps of the distribution of spruce (top 4 maps) and ash (bottom 4maps) in North America from 21,000, 15,000, 13,000 and 1,000 years ago showing that spruce moves northward as the ice recedes but ash stays put]
Moreover, I’ve only been showing you spruce, mostly because it’s its a nice kind of iconic one, but if you actually were to look at other taxa and start to look at how they respond, what you start to see is that different species respond to different rates and in different directions. And so, we can see that that spruce again, northwards shift. We look at ash. Ash doesn’t really move much of anywhere. It expands a little bit north. There’s a bit of a northern range shift to it. But mostly what ash does, it goes from being not very abundant to being really abundant, between 15,000 and 13,000 years ago, and then not being very abundant again. And so, it’s a completely different trajectory from what we see from that, sort of, simpler story of, sort of, northward expansion with rising temperatures.
And notice that today we think of spruce being up in Canada and northern Wisconsin and ash being parts of southern Canada and and the eastern deciduous forests. So, we think of these as largely disjunct species from different biomes or or ecosystems. But if you’ve been an ecologist walking the landscapes 15,000 to 13,000 years ago, you would have thought that spruce and ash were just that was a normal association. And so, the mixtures of species change. What we start to mean by novel communities or reshuffling of species in novel mixtures. That as – as climates change, each species follows its own path. And as a result, you get this mixing of and breaking down of exiting community around you and new ones emerging.
We should expect the same sort of things happen in the future. We should expect that because of this, some of these co-evolutionary relationships should be pretty weak. If your neighbors are changing all the time, maybe you’re not tightly co-evolved with the other species around you
[Jack Williams]
– and so, we’re starting to think about some of those implications for co-evolutionary relationships. And, again, this, sort of, prediction question.
Another point that’s, I think, of real interest is that if you actually look at the last, you know, 1.8 million years, even going further back, one thing you don’t see a lot of are species extinctions. Species extinctions are there. They’re theyre not nothing, but they’re not very common either. And you can make a good case and theyre not much above, sort of, back to what a, sort of, typical background rate, species are kind of always coming and going and that that might be sort of typical. And when you do see extinctions happen, they often tend to be at the cold periods. That sometimes species die out either locally or regionally during the cold glacials
[slide titled, Climate-driven Extinctions Rare in Recent Past, featuring the Quaternary Conundrum why did so few species go extinct during past climate changes? The slide also shows a graph of the marine isotope record for the last 3 million years showing fewer extinctions during warmer periods than colder periods]
– but not as much of evidence of extinction during the warm glacials. So, there’s some sense that there’s a bit of asymmetry here where the colder periods are more stressful for species and can drive extinctions more than the warmer periods.
This is sometimes called the Quaternary conundrum there because I just showed you a few slides ago these predictions of large rates of, you know, 15% extinction rates over this century in response to 21st century climate change, and yet, when we look at the geological record, we just don’t see that much of it happening.
[new slide titled, One Major Exception to Conundrum Death of the Megafauna, featuring an illustration of a wooly mammoth and the observations that worldwide at least 97 of 150 megafauna genera go extinct, the timing was 45,000 years ago to the late Holocene, in North America 34 genera go extinct and the classic question is what killed the megafauna?]
But the obvious counterexample to that, I’ve been focusing mostly on plants so far, but of course there was these very large animals roaming these landscapes, we used to have mastodons all over the place around here, and those mastodons aren’t here anymore. The mammoths aren’t here, the camels aren’t here, the horses, you know, all over North America. And, worldwide, we do have a major extinction event, but it’s very much concentrated on the big animals. We lose about two-thirds of all the big animals worldwide starting about 45,000 years ago up until the recent past. In North America, we lose lose lose about 34 genera and this long-running question about what does them in.
[new slide titled, Strong case for human role, featuring that same marine isotope graph from two slides previous along with a new set of graphs that plot the various sizes of species (x-axis small to large) versus number of species (y-axis) for North America, South America, Australia and Europe/Asia/Africa showing most extinct species being on the larger size for each continent. Additionally, it is noted that animals survived previous glacial-interglacial cycles, the extinctions were at different times on different continents and extinctions were restricted to large mammals]
And I’m of the personal mindset that it is some combination of climate change and human dispersal, right? Humans are moving out of Africa beginning around 100,000 years ago or earlier, they spread into Europe, into the southeast Asia, into Australia by about 40,000 to 50,000 years ago, into the Americas by about 15,000 years ago. And, interestingly, it’s not a perfect correspondence. It’s not its not as if, you know, the early hypotheses were this blitzkrieg hypothesis that when people showed up, very quickly they – they hunt down the animals.
[Jack Williams]
And now it looks like there’s often a period of of coexistence for several thousands of years, but then the animals do die out. And, for me, the the the telling point is that we don’t see waves of large animal extinctions during all these previous glacial and interglacial cycles going back the last million years.
[return to the Strong case for human role slide]
It’s only the most recent one where these extinctions happen. And, to me, that’s, sort of, one of the key signals that it’s this new factor of climate change plus this new predator, new large animal on the landscape that’s causing these extinctions to happen. It’s also telling that if you look at, you know, the – which animals go extinct, it’s the very large ones. And those bar charts over there are showing the blue, it’s its its rated rated by body size there, and then the the the vertical axis is the number of species that go extinct, or, sorry, the number the number the number of taxa in that body size. And so, what you can see is that the red ones are the ones that go extinct. The blue ones are the ones that survive. And so, the extinctions are very heavily concentrated on the big N, the ones the animals that will be the easiest to find, the most resources for for early hunters to get. So, and the timing and pattern is consistent as well.
[new slide titled, Novel environments produce novel ecosystems, featuring a return to the set of eight maps of North America from 21,000, 15,000, 13,000 and 1,000 for spruce top four maps – and ash bottom for maps showing their response to the receding of the ice. Highlighted are the four maps from 15,000 and 13,000 years ago that show large concentrations of both spruce and ash in the same general vicinity during these time periods]
Okay.
So, we see this idea of novel ecosystems that I talked about. Species, and that and I’ll show in a moment, a good case to be made that these novel communities are forming in part in response to the novel environments that they encounter that exist in the past that don’t exist today.
[new slide titled, Key no-analog plant taxa were poised to exploit these past no-analog climates, featuring an illustration of the current geographic and climatic range of ash trees in North America next to a graph that plot summer temperatures versus winter temperatures for ash as compared to the earlier graph of summer versus winter temperatures for the various and future time periods that was shown in a previous slide showing areas where there was no overlap]
And one way of looking at this, going back to this point about seasonality, remember what I showed you at far right there, that 15,000 years ago climates in the past were more seasonal than they are today. Now look to the far left and look at where ash is found today. And that middle plot here, I don’t know how well you can see it, but there is this black set of dots and, maybe a little harder to see, a set of gray dots here. This is the climate space of North America. And what this figure should be showing is that we have, the black dots is where ash is present in North America. The gray dots is where ash is not present but those climates exist. And then, for much of ash’s range, we have some constraints that ash cannot be found in these areas. We don’t find ash in these areas at least.
But over here ash is right up against the edge of the North American climate envelope. It’s its found right in the middle of North America, which is as seasonal as it gets in North America. So, there’s some sense that ash could expand into these climates, these more seasonal at present climates, if those were to exist. They don’t exist now, but these climate simulations suggest that they did exist – exist in the past. And so, some of these, sort of, spruce, ash assemblages, and some of these other no analog communities that formed we think are forming in response to this
[Jack Williams]
– heightened climate season – temperature seasonality of this, sort of, late Pleistocene time period.
So, one consequence then, as I showed you
Oops. I don’t have a
Oh, yeah, so I showed you a map a few moments ago about oak, I’m looking at how oak does. This is another map for
[slide titled, Ecological forecasting models have a tough time predicting into novel climates, featuring a set of four maps for the distribution of Fraxinus from 15,000 years ago compared to present day the upper two maps being the observed distribution and the lower maps showing the modeled/predicted distributions. The modeled present map shows that Fraxinus (ash) should not exist at all in North America but in reality, there are large swaths of North America where Fraxinus is abundant]
– Fraxinus. This isn’t quite as clean as the other one I showed you ago. But, again, here’s the modeled distribution of oak 15 – of – of ash 15,000 years ago. We take that model, predict it forward to present, and the model basically thinks that ash shouldn’t exist at present or should exist only at very low abundances. Where, in fact, we know that ash does exist at present. And so, this is a good – good – and the correlation between these two maps is terrible; it’s, you know, 0.09 correlation. And so, this is a good example where the model is not matching to the data. It’s having a hard time taking these novel climate spaces of the past and then predict – basing predictions on them into the climates of today and the species of today.
[new slide that replaces the four maps of predicted and actual ash distribution with a graph of the time window used to calibrate versus the correlation for ash where we find that predictions for more recent time periods are much more accurate than past predictions]
Just like that oak plot, we saw that – that, in general, time – predictions based on more recent time periods do better, predictions based on earlier time periods do worse, but now, if we look down here, the correlation, again, is very low. And so, basically, if you – what this is telling us is if you take a – the set of climates that we know for 14,000 years ago and the known distributions of – of ash from 14,000 years ago, build a predictive model based on this data and relationships, that model has little or no predictability to today. And so that is an example of how these novel climates can confound these models because, again, they’re statistical models. They’re not very good at extrapolation into the unknown. This is a case where they’re really breaking down in these sorts of situations.
[new slide titled, These taxa appear to be exploiting past novel clime space – shifting realized niches, with two graphs showing the distribution of ash seasonally (summer vs. winter temperatures) for the time periods of 15,000 years before present and 1,000 years before present showing a decrease in the abundance of ash over this time period]
And one more just, kind of, visualizations around this; this is showing the, sort of, total climate space of North America 15,000 years ago, the total climate space of North America 1,000 years ago, and then the black dots being the places of high ash abundances. And so, what you can see is this portion of climate space that opens up down here is also a place where we’re finding lots of ash. The ash is really preferentially occupying these highly seasonal climates, these climates that don’t exist today. And so, again, this is, sort of, these signals that as climates change and new portions of climate space open up, species are able to respond to and occupy these spaces and are – are sometimes our ecological forecasting models have a hard time predicting these novel climates and community responses to them.
[Jack Williams]
Okay, so those are some of the lessons, some of, kind of, the big, kind of, things we can take away from what we see from the geological record. And now just a – just a few slides at the end about what should we do. What kind of things can we – you know, we take this information and bring it to bear on – on current challenges?
In general, if you’re thinking about climate change and you’re sort of working at a high-level thinking about sort of policy options, there’s sort of two general options.
[new slide titled, Two main solutions, with the list of either mitigation – reduce our greenhouse gas emissions or adaptation – increase our resilience to climate change. Mitigate to avoid that which you cant adapt and adapt to what you cant avoid]
One is mitigation, which you try to mitigate or prevent climate change by reducing our greenhouse gas emissions. And the other one is adaptation, where you try to increase our resilience to climate change.
When I first came into – into the field, you know, 20 years ago, adaptation was viewed as, kind of, a taboo, that we shouldn’t be thinking about adaptation, we should be really focusing our efforts on mitigation. But increasingly, these are seen as not either/or, it’s and/both kind of situations because you want to avoid the really high-end climate scenarios that might cause a four-degree or five-degree warming this century that would cause all kinds of harm to low-lying areas, small islands, heat waves, you know, droughts, etc., and, you know, rates of adaptation –
[Jack Williams]
– for species and so forth, you want to avoid those outcomes, and mitigation does that for you. It moves you away from the high-end climate change scenarios down to the lower-end or middle-end climate change scenarios. Whereas, adaptation recognizes that climate change is already underway, you know, climate change is – is happening. It’s been underway for the last several decades. And now –
[return to the Two main solutions slide]
– it’s just a question of how much climate change we’ll have, and at what point we level out or maybe start to reverse things. And so, the Paris Accords said we need to stay at 1.5 degrees Celsius. So, that would put a limit around here or so. So, there’s different scenarios and pathways to get there, but no matter what there’s going to be some amount of climate change and some amount of adaptation need – needed to those. And so, adapt to what you can’t avoid.
[Jack Williams]
And so, if you think about, from an ecological perspective, one thing to recognize is that, again, species accommodate climate change by moving –
[slide titled, Accept and facilitate range shifts, featuring three maps of the distribution of spruce, oak and ash in North America that start with the last ice sheet and animate to present day distributions]
– but that’s actually been sort of a hard concept for us. You know, we have legal restrictions in place. We think about invasive species, there’s some species you can’t move across certain boundaries. And I’m certainly not advocating for wholesale movement of species across continents. We know about all the issues of moving exotic species into places they’d never been before. But within continents, within regions, we should recognize that species have moved in the past, and they will move in the future and doing things like adaptive planting, looking for seed sources from populations, maybe not in the current area that where you’re trying to reseed an area – a species, but to the south of it. And so, organizations like the U.S. Forest Service are starting to change guidelines so that simply, sort of, taking a seed from the current areas, they start to look for seed sources a bit further south and so forth. Some places, like in Alberta – Alberta – foresters in Alberta are actively planting species outside of their historic ranges –
[Jack Williams]
– in the expectation that by the times those trees come to maturity 60 or 80 years from now, the climates that they’ll grow up in will be the climates favorable to them and so they’re doing sort of proactive planting for future forestry and harvesting.
This gets in this topic of managed relocation. And so sometimes if – if you’re worried that a species habitat will entirely disappear –
[slide titled, Managed Relocation, stating that when necessary, relocate vulnerable populations featuring a map of the world with six photos of species that may benefit from managed relocation – American pica, Iberian lynx, three butterfly species, and two plant species]
– or largely disappear as a result of climate change, maybe due to sea level rise or other factors, moving these vulnerable populations, picking them up from one place to north. This, again, can cause concern. I tend to be of the perspective that this is a natural process, it’s just one that we haven’t seen happen much because we haven’t seen that much climate change happen in our human lifetimes. And so, this is the kind of thing I tend to support as a – as a way that’s congruent with past climate change and past adaptations to it.
[Jack Williams]
And also recognize that what we think of as a normal or natural set of species around us may be a temporary phenomenon.
[slide titled, Prepare for and accept emergence of novel ecosystems, with a return to the set of eight maps showing the distribution of spruce and ash at various times since the last Ice Age]
Species move, associations come and go, and then as climates change, we should expect these shifting associations to happen in the future. Some species moving faster than others, some species being more temperature sensitive or rainfall sensitive or extreme sensitive, and so we’ll expect –
[new slide titled, There is a huge debate in restoration ecology about novel ecosystems, featuring two quotes. The concept of novel ecosystems, in its various formulations, is based on the fact of novel ecosystems – there is indisputable evidence that some ecosystems have departed entirely and irreversibly from their historical analogs and The novel ecosystem concept is based on the premise that we should be pragmatic and accept unavoidable, wide-spread, and irreversible changes in ecosystems caused by climate change, biological invasions and other altered global processes. This premise presents and inaccurate forecast for the planet.]
– to see this reshuffling of species. This has led to this concept of novel ecosystems, and there’s a bit of an argument about that right now. And there’s a couple of quotes about that argument. And so, Hobbes, for example, is arguing that novel ecosystems, the concept of novel ecosystems is based upon the fact of novel ecosystems. He’s saying already we can see that species – that some ecosystems have departed entirely from their historical analogues. Whereas other have argued, No, no, no, we can keep things in a current state. We – we – we – we shouldn’t sort of wave the white flag of surrender but accept these unavoidable and widespread irreversible changes. We – and so, there’s been an argument about this. What should we be conserving to? What should we be maintaining?
[Jack Williams]
I, again, tend to favor more of the former perspective. That these are dynamic systems that are systems in changing and we should look for ways to facilitate those changes, one that best promotes biodiversity and the – and the services that we depend upon.
And, again, we expect that climates are changing, we expect novel climates to emerge this century.
[slide titled, Novel climates expected to emerge this century, featuring a map of the world with the predicted distribution of novel climates by 2100 A.D.]
So, this is very much a real process for us all to be wrestling with. And that – we are wrestling with in the professional sector, and I think it’s bring – worth bringing out to the broader community as well.
[new slide titled, Novelty already increasing in all ecosystems – due to a variety of factors, featuring a set of three photos – Parthenium rangelands, Puerto Rican forests, Isle Royale and a map of the Coral Triangle]
And if we look around, I’ve been focusing here on climate change, but if you think about it, there’s, of course, all kinds of other factors transforming these ecosystems. There’s been invasive species. There has been land use and legacies of past land use. So, the Parthenian rangelands is an example of a toxic, you know, invasive species that we generally try to, you know, limit its spread. Puerto Rico is an example of an area that was widespread sugar plantations, is now reforesting. And as it’s reforesting, it’s becoming this interesting blend of native and exotic species, apparently with, you know, not major negative ecosystem or biodiversity consequence. If anything, biodiversity may have gone up in some of these areas with this mixture of natives and exotic.
Isle Royale is being a relatively pristine area but one that nonetheless is changing in many ways. And the Corral Triangles as an area that is changing and is – and is threatened by rising temperatures, ocean acidification, and so forth. And so, we can recognize that the world is changing, we can recognize that novel ecosystems are emerging. Some of these we might view as more positive than official, others we might view as more negative or ones to try to reverse, but, nonetheless, we should recognize that we are in this changing world and that we should seek to facilitate certain kinds of change and mitigate or reverse others.
[new slide titled, In a Nutshell, summarizing these basic facts – we live in a rapidly changing world, we can use the past to study how species respond to past climate changes, when climate changes, species move, novel ecosystems have emerged in response to past novel climates, we must mitigate climate change and adapt to it, we should accept and facilitate species range and shifts and we should accept and prepare for novel ecosystems – expect the unexpected]
Okay, so that’s – thats, I’ve kind of worked through these points here already. But, again, this perspective that comes out of paleoecology of the world has always been in flux. Now new changes are happening. We are accelerating the rate of change. And, in general, I would say that prudence would dictate that we try to slow down rates of climate change, we try to stabilize the climate system, but we should also accept that change is underway and that those rates of change lead to adaptation needs –
[Jack Williams]
– and those adaptation needs can be grounded in the lessons from the past.
Thank you.
Thanks a lot.
[applause]
Search University Place Episodes
Related Stories from PBS Wisconsin's Blog

Donate to sign up. Activate and sign in to Passport. It's that easy to help PBS Wisconsin serve your community through media that educates, inspires, and entertains.
Make your membership gift today
Only for new users: Activate Passport using your code or email address
Already a member?
Look up my account
Need some help? Go to FAQ or visit PBS Passport Help
Need help accessing PBS Wisconsin anywhere?
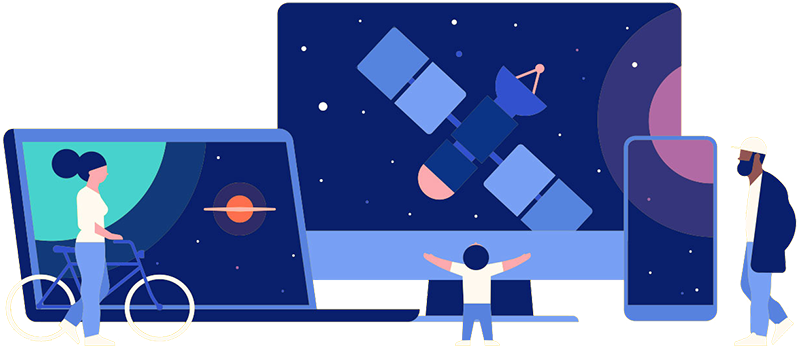
Online Access | Platform & Device Access | Cable or Satellite Access | Over-The-Air Access
Visit Access Guide
Need help accessing PBS Wisconsin anywhere?
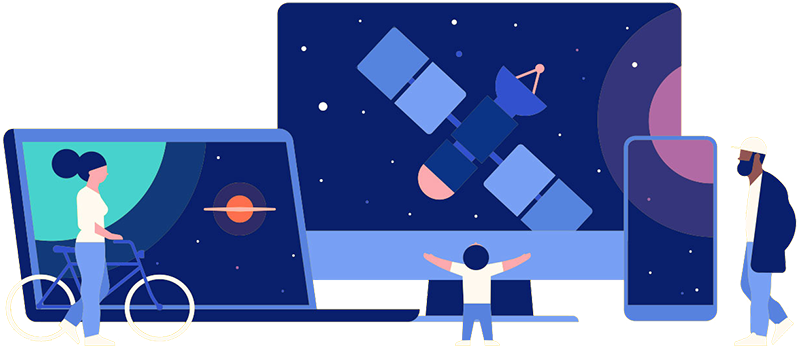
Visit Our
Live TV Access Guide
Online AccessPlatform & Device Access
Cable or Satellite Access
Over-The-Air Access
Visit Access Guide
Follow Us