[Tom Zinnen, Outreach Specialist, Biotechnology Center, University of Wisconsin-Madison]
Welcome, everyone, to Wednesday Nite @ the Lab. Im Tom Zinnen, I work here at the U.W.-Madison Biotechnology Center. I also work for U.W.-Extension Cooperative Extension, and on behalf of those folks and our other co-organizers, Wisconsin Public Television, the Wisconsin Alumni Association, and the U.W.-Madison Science Alliance, thanks again for coming to Wednesday Nite @ the Lab. We do this every Wednesday night, 50 times a year. Tonight, it’s my pleasure to introduce to you Raluca Scarlat.
Shes a professor in engineering physics here in the College of Engineering. She grew up in the Bay Area and went to Irvington High School there. Then she went to Cornell University for an undergraduate degree in chemical engineering. Then she went to the University of California-Berkeley back home and got her PhD in nuclear engineering. And then she came here in 2014 to take her faculty position. Tonight, shes going to be talking about the next generation of nuclear reactors.
This is a topic more near and dear to my heart than I realized because my brother worked at a nuclear power plant, the one in Cordova, Illinois, for about 30 years. He retired about three years ago.
You get a – a feeling for ups and downs of a technology when your brother reports to you every Christmas and Easter and all that good stuff. So, Im looking forward to hearing what the future is of this. Please join me in welcoming Raluca Scarlat to Wednesday Nite @ the Lab.
[applause]
[Raluca Scarlat, Assistant Professor, Department of Engineering Physics, University of Wisconsin-Madison]
Thank you very much for the invitation and the introduction. So, I find that when I meet up with friends or go to parties and people ask me, So what do you do? And I say, I’m a nuclear engineer. Then the conversation ends.
[laughter]
And nobody knows what to say. They look a little embarrassed and then they walk away.
[laughter]
So – so, I – I hope that – that – that – I hope that we can change that. I hope that nuclear engineering will become less of a polarizing topic.
And so, thats what Ill hope to do through my talk today is to give a little bit of nuance to nuclear engineering, so that the discourse can be a little bit more than about, Should we have nuclear? or Should we not have nuclear?, but a little bit more about how and why.
And this – this parallels also a little with my career path in that I didnt start as a nuclear engineer. I started as a chemical engineer. And I really went to grad – I – I worked in the petrochemicals industry and I – I was really interested in energy and sustainable energy and clean energy. And I went to graduate school in nuclear engineering because I couldnt quite convince myself if nuclear energy was something to be explored or not. I just couldn’t – I couldn’t find enough information that was convincing either way.
And – and after graduate school, I stayed in this field for the same reason because I felt like there are really some unanswered questions about how nuclear could be explored responsibly.
And so, thats – thats my field of research and teaching today at the University of Wisconsin-Madison, is nuclear reactor safety and nuclear reactor design.
And Ill talk to you about the motivation for why we should even consider nuclear –
[slide titled, Story-board, with the outline of the talk, 1 – high energy density, no atmospheric waste, 2 – already a significant part of the global economy, 3 – Alchemy, 4 – Intermission – why cant nuclear grow, 5 – next generation of nuclear technology, 6 – molten salt reactor technology, and 7 – research at the University of Wisconsin-Madison]
– the current role of nuclear energy in global energy production. Then well talk about alchemy. And then therell be an intermission to talk about why cant nuclear grow? Ill talk about the next generation of nuclear technology, what the future might have in store for us. Molten salt technology is one of the future nuclear energy technologies thats being studied, that I study in my group. And then Ill talk about the work that I do in my research lab.
[new slide titled, Energy Density Part I – The Fuel, showing a thermal image of the Deep Water Horizon B.P. oil spill in April of 2020 from space, noting 18.7 billion dollars in fines, 4.9 million barrels of oil spilled equal to one day of U.S. energy use and that per capita thats $60 and half a gallon]
So, part one, energy density.
In 2010, there was a oil spill in the Gulf of Mexico, the Deepwater Horizon oil spill, that led to about $18 billion in fines. It spilled about five million barrels of oil. And it cost, per capita, about $60, and it spilled about one half of gallon of oil. That’s equivalent to one day of U.S. energy usage in one spill. And that – I like to start with this image because it reminds us of the scale of energy consumption and the scale of the fuel required for the energy that we use.
[slide animates on that this oil spill is equivalent to 6 barrels of uranium ore]
So, if we take the energy of – that is used in the U.S. per day and we look at how much nuclear fuel might be necessary, we end up with six barrels of equivalent uranium ore. So, were comparing six barrels of crude uranium ore to five million barrels of crude oil.
So, this difference in energy density of the fuel is the first reason that we should look towards nuclear as a possible source of energy because its so much more compact. We dont use the energy of the electrons that circle around the atom. We use the energy of the nucleus. The nucleus is much more compact than the electrons that circle it. And the energy thats stored in the nucleus is much more than the energy thats stored in the electrons that surround it.
[new slide titled, Energy Density Part II – the Waste, with three points, 1 – human metabolism – how much kg of CO2 do you expire per day, 2 – CO2 from U.S. energy use per capita, and 3 – how much uranium ore per year per capita]
So, the other part of energy density has to do with the emissions, the waste that is produced.
So, how many kilograms of CO2 do we expire per day?
[Raluca Scarlat]
Does anybody know? Yes?
[first male audience member]
Four or five kilograms.
[Raluca Scarlat]
Four or five kilograms. Does anybody want to take another guess?
[second male audience member]
10.
[Raluca Scarlat]
10 kilograms.
So, the – the CO2 that we expire comes from the food that we combust. As we eat the food, we burn it, and we release CO2 from the carbon matter that we eat. We inhale oxygen and we release CO2. And we use that energy in our body. Its not unlike combustion or burning in – in a fire except that its slower and more controlled. So, most of the food that we consume throughout a day – throughout the day comes out as the mass of CO2 that we expire. So, if we eat on the order of two kilograms of food per day, we expire on the order of two kilograms of CO2 per day. So, this should give us a scale for what do we mean by CO2 emissions.
Now, how much CO2 do you think that we release per person in the U.S. per day from all of the energy usages that we have in the U.S.? So, that includes transportation. It includes electricity. It includes the heat for industrial applications. So, making furniture, making processed food, all of that takes energy, sometimes in the form of heat, sometimes in the form of electricity. So, all of that takes energy, and the energy – some of that energy comes from natural gas and coal. Those are hydrocarbon sources that emit – emit CO2. So, how much CO2 do you think is emitted per person from all of the energy production –
[return to the Energy Density Part II – the Waste slide]
– that is done in – in the U.S.? Does anybody want to take a guess?
[third male audience member]
20?
[Raluca Scarlat, off camera]
20? So, on the order of 20, thats – thats right. We – its 18 times – its about 18 times what we – what we exhale per day. So, you would require 18 people for – in order to sustain our lifestyles, our energy consumption. Now, there is a difference between the CO2 that we exhale and the CO2 thats –
[Raluca Scarlat, on camera]
– emitted into the atmosphere from fossil fuel and that difference is its source. The CO2 that we exhale comes from the food that we grow. And the food that we grow gets its carbon from the air. The plants absorb CO2 and make that into plant matter. And so, the food that we eat, we then exhale the CO2 out, and that gets reabsorbed into the plants and we – we eat it. Its a continuous cycle. Were not adding, when we exhale, were not adding to the CO – the overall CO2 in the atmosphere. Were just part of a cycle.
Whereas, with fossil fuel, that cycle has been broken because a lot of plant matter was buried underground and became petroleum and natural gas. And now what were doing by combusting that plant matter, that organic matter, is were releasing it from underground into the atmosphere. So, thats no longer a cycle. Were adding to the CO2 that is in the atmosphere. Were using the atmosphere as our landfill, except in this case, its our airfill.
So – so then, lets contrast that with the amount of ore that is necessary for nuclear energy production. One of the fuels for nuclear energy is uranium; not the only one, but one of them. And we can think of nuclear fuel, we extract it from the – from the ground as solid fuel. We combust it in a nuclear reactor. And the combustion products are solid. So, it stays as a solid throughout its lifetime. So, as a proxy for nuclear waste, we can think of the total amount of fuel that we use thats also the total amount of waste that we produce.
Now, there are methods to recycle it and sort of reduce that volume by reusing it over and over, but as a proxy order of magnitude, what might be the amount of – of nuclear fuel that we use? So, what mass do you think one would need? Yes?
[fourth male audience member]
A gram.
[Raluca Scarlat]
A gram? Yes, another guess?
Its a little bit more than a gram. Its 56 grams.
[return to the Energy Density Part II – the Waste slide now with values for all the questions of CO2, 1 – kg of CO2 per day for one person – 1Kg per day or .4 tons CO2 per year, 2- per capital CO2 consumption – 6.6 tons CO2 per year per person (18kg exhaled), 3 – uranium ore per year per capita – 56g = the weight of an iPhone, 4 – lifetime emissions – 660 tons CO2 per capita, 6kg uranium]
So, its about half the weight of your iPhone. And so, now if we put these numbers in comparison on a per capita basis, so in our lifetimes, from fossil fuel, we would emit about 660 ton of CO2 per capita. And for – if we were to use nuclear energy instead, we would generate six kilograms of nuclear waste per capita.
[new slide titled, Waste from Your Lifetime Energy Needs, featuring the cover of a National Geographic magazine with the main headline of What Dogs Tell us with a picture of a poodle on it and next to the magazine photo the statistics Fossil Fuel 10,000 times you for atmospheric waste versus 1 times a four-month-old poodle of solid waste for Nuclear Fuel]
I tried to find a picture that would be representative of six kilograms. And it turns out that a four-month-old poodle weighs about six kilograms.
[laughter]
So – so, a four-month-old poodle –
[Raluca Scarlat]
– would be your lifetime solid waste generation for all of your energy needs to sustain our lifestyle, as compared to fossil fuel where 660 tons is the weight of about 10,000 people. So, that’s the scale comparison.
Another comparison I like to point out here is that in the case of fossil fuel, the landfill is really in our atmosphere. So, its atmospheric waste that we dont manage or control, whereas, in the case of nuclear fuel, it is solid waste that we manage – that – that we manage safely.
And so, this is – this is the motivation for learning more about nuclear power, from learning more about whether we can explore it responsibly and safely and in a manner that the – in which the risks of this technology are far smaller than the benefits of the technology.
So, is nuclear energy already used today? It is.
[slide titled, Global Nuclear Energy Production, featuring two graphs – one of worldwide distribution of civil nuclear energy generation capacity showing over 80 percent of capacity in countries that produce more than 18 Gigawatts and a second graph showing the top planned nuclear reactors by country with China, Russia, and India in the top three spots. Additionally, the following statistics about nuclear energy – worldwide = 447 power reactors in 31 countries, 390 GWe installed capacity = 7% of global capacity, 60 reactors under construction = 16% of existing capacity, and in addition, 250 research reactors in 55 countries]
In – there are about 450 reactors around the world in 31 countries. The United States, France, Japan, Russia, Korea, India, the U.K., Canada are some of the countries that have the largest number of reactors. And then there’s a large number of country – of countries that have one or two reactors in their country. And what is also very important to know is that there are a large number of reactors planned and under construction around the world in countries that already have nuclear power and also in countries that do not have nuclear power yet and are learning to develop this technology. So, China, Russia, India being the top countries where – where reactors are being constructed and being planned.
[new slide with a graph titled, U.S. Electricity Net Generation by Fuel Source 1949-2013, showing year on the x-axis and millions of kilowatt hours on the y-axis, showing coal and natural gas as the largest energy providers with nuclear energy coming in at third]
In the U.S., about 20% of electricity comes from nuclear power. We have about 100 nuclear reactors. And in the state of Wisconsin, about 15% of the power comes from the one nuclear power plant that – that we have in the state of Wisconsin.
[new slide with four maps of the U.S. noting the number of commercial nuclear reactors in the years, 1966, 1973, 1985, and 2014 showing an increase in the number of reactors built with the largest concentration of new nuclear plants having been built on the East Coast and the Midwest]
And whats interesting to note is when these reactors were built. This is a time flash of – you start, if you start in the night in the top left corner, 1966, reactors – commercial reactors started being built; 1973, there were more in the Midwest and on the East Coast; 1985, there were a few more on the West Coast and quite a lot more in the Midwest and on the East Coast. 2014 and 1985, begins to be a challenge of Wheres Waldo? to figure out if there were any new plants being built. The – the – the map doesnt look very different between 2014 and 1985. There was a long gap in which nuclear was not very much of interest.
And the main reason that nuclear was not of interest is that climate change was not a concern in 1985, so – and the cost of nuclear was not clearly competitive with fossil fuel. So, natural gas was cheap and abundant, so was coal. Climate change was not a concern, so there really wasnt a strong impetus to work on – on nuclear energy.
[new slide with a map titled, U.S. National Parks and Nuclear Power Plants, with blue dots around each of the nuclear plants representing a 50-mile radius of each nuclear plant]
And Ill – this is a print version of the map that I have on the screen. Ill pass this around. Each of the circles that you see is a 50-mile radius around each of our nuclear power plants around the United States. Point Beach is the – the plant in Wisconsin. Why is 50-mile relevant? Nuclear power plants operate in a way that does not release radioactivity in the environment. And they – they not only put a lot of thought into how to ensure that radioactivity is not released but they also put a lot of thought into how to show that radioactivity is not released. So, how to verify this assumption that power plants do not contaminate the land, and 50 miles is the radius on which there is food sampling done and soil sampling and water sampling.
And then, what I also added to this map is – is the national – the – the national parks. So, its interesting to see that some of the nuclear power plants are quite close to national parks. If youd like to learn more about this map, nuclearenergy.edublogs(.org) is the site that I use for the courses that I teach, and youll find more information about this map there.
[return to the Story-board slide with the outline of the talk]
So, the next topic –
[Raluca Scarlat]
– that Ill talk about is alchemy. And why talk about alchemy? Where alchemy is the change of any material into gold.
And many chemists a long, long time ago worked on, Can we make gold using chemistry? And the answer was, No, we cannot. But we can make gold using nuclear science.
So, commercial nuclear reactors –
[slide titled, Water-cooled nuclear power plants, with an illustration of all the elements and workings of a nuclear power plant with an inset photo of a steam powered locomotive train]
– make gold every day. And this is how they do it. They start with uranium, which is a rock, youll find uranium in granite. Or thorium, thats another fuel that can be used. Thorium is co-mined with rare earths. Rare earths are used in electronics, so, iPhones, computers, they all use rare earths. And thorium is a byproduct of min-mining rare earths, which is stored as a waste product at the moment, but it could also be used as nuclear fuel. So, we start with a rock. We purify it. And then we put it in what we call a reactor, which is essentially a pressure cooker. It is actually literally a pressure cooker because it has water in it, and its pressurized. So, you pressure cook some rock, and you get gold and a lot of energy.
And how that works is that the thorium and uranium are very heavy nuclei and we split them, and in splitting them, we produce gold and lead and pretty much the entire periodic table. We also produce a lot of energy from that splitting. And that energy we harvest as heat that goes into the steam that we produced in our pressure cookers. And that steam is then used to run steam turbines. And steam turbines are not very much different from the way that trains used to run a long, long time ago. So, one could say that this is extraordinarily old technology coupled with extraordinarily new technology. So, why this coupling and why haven’t we evolved a little bit from pressure cookers and steam power?
The answer has to do partly with the map that I showed you before, that in 1985, we stopped developing nuclear power. So –
[Raluca Scarlat]
– there wasn’t a strong commercial incentive to develop and commercialize new technology.
So – Ill – so, Ill tell you, now today, there is a motivation to develop nuclear technology, and that is climate change. And also, energy security and geopolitics could be motivations for developing new technology. And it is possible to move away from steam turbines to – to what we call air turbines, a more modern technology that would then become more economically competitive with fossil fuel. But before we fast forward to the future and the present development, I want to tell you a little bit more about alchemy.
[slide titled, Alchemy – nuclear energys biggest challenge – fission and neutron absorption, featuring a diagram of the periodic table with red arrows pointing at colored elements that are labeled as fission products and a grouping of heavy elements highlighted in blue]
And so here is a periodic table. At the very bottom of the periodic table are the heavy elements. Theyre heavy because they contain a lot of neutrons and protons. And here at the bottom we have uranium, neptunium, plutonium, thorium. These elements split to produce the elements that you see in color here. So, they produce a large fraction of the periodic table, and these elements that are produced are towards the top of the periodic table, so theyre lighter. Weve split a heavy atom into two lighter atoms.
And these lighter elements that are produced, some of them are in their natural, non-radioactive state. Some of them are in a state that is not quite yet stable, so they emit radioactivity, they emit energy, until they reach a stable state.
So, that means that if we run a reactor that splits uranium, once we stop our reactor and we stop splitting uranium, we still have those radioactive elements that weve produced that will continue to be radioactive until theyve reached a stable state. And that does not happen instantaneously.
[Raluca Scarlat]
We cant stop those elements from being radioactive until they naturally reach their stable state.
And that is one of the challenges with nuclear technology, that we dont have a stop button that is quite a stop button. We have an almost stop button.
[laughter]
But its not a complete stop button. So that means that we continue to produce heat. So, if we have our pressure cooker and we continue to produce heat and we dont take out that heat, then our pressure cooker, at some point, will pop. And thats part, in a way, what happened at the Fukushima plant in Japan.
We – the – the – the reactors shut down because there was an earthquake. Following the earthquake, there was a tsunami wave that flooded the plant, and as it flooded the plant, it flooded the – the electrical switchyards and the emergency generators, diesel – diesel generators. So, the plant was without power, without means of any pumps. And if there were no more pumps at the site, that means that there was no way to pump water, and if there was no way to pump water, there was no way to cool the plant. So, that energy that was continuing to come from the decay of radioactive elements in the core was building up in the reactor itself and overheating the system.
And if we overheat the system, that means that we break our pressure cooker, we break our vessel, and whatever is inside begins to leak. And what’s inside? Its – its those radioactive elements that weve produced from splitting uranium, and such as iodine and cesium. And if iodine is released into the environment, it is then absorbed into our bodies. And a radioactive element absorbed into our bodies will damage our D.N.A. And there are natural mechanisms of repairing our D.N.A., but if the rate of damage is higher than what our bodies can keep up with, then it can lead to disease.
So, in order to prevent release of these radioactive elements into the environment, we have to ensure a means of cooling the reactor, even after weve shut it down, so that we can remove that heat thats generated by the radioactive elements and safely contain them.
Now, another challenge, and – and so to give you a sense of how leaky of a stop – stop button the – the shutting down of the – of the uranium reaction really is, this is a plot of the power level in percentage –
[slide titled, Heat produced by Fission Products and Management of Used Waste – after reactor shutdown at time = 0, showing a graph with time on the x-axis and the fraction of full power on the y-axis showing a long gradual release of heat after the reactor shuts down]
– after the reactor has been shut down. So, this is one second. And 6% of the power is being produced one second after weve shut down the reactor. And then if we go down to two hours, were at 2%. If we go down to a day, were at about 1%, and 1% from one gig – from a one-gigawatt plant is still a lot of power that one needs to remove.
[Return to the slide with the periodic table]
Now, another challenge related to alchemy is these other elements at the bottom of the periodic table that are produced in the reactor. So, if we start with uranium, as uranium splits and produces these other elements, it also produces neutrons. And those neutrons can be absorbed in other uranium elements and – that do not split quite yet. And the absorption of neutrons into the uranium element allows us to hop over to the next element. So, we can hop up to neptunium, plutonium, americium. And plutonium is a material thats also been used for nuclear weapons production. So, one of the challenges of – of handling the – the used fuel from a nuclear reactor and recycling the used fuel from nuclear reactor is demonstrating that this plutonium that has been produced cannot be extracted by – by somebody with intentions that are not in the interest of the state to produce nuclear weapons.
And I – I mentioned earlier that there are a large number of countries –
[Raluca Scarlat]
– that are looking to build nuclear reactors that do not already have nuclear technology. And so, the challenge for them in the international politics context is to demonstrate that they are going to use nuclear technology for peaceful uses, not for the development of nuclear weapons.
And if one is building nuclear reactors and running nuclear reactors, then one is producing plutonium. And if one wants to recycle this fuel that weve used, then one could maybe extract the plutonium for non-peaceful purposes. But one could also not do that. And so, the challenge of exporting nuclear technology is having in place the institutions, both international – both international and national, to ensure that the application of nuclear technology is for peaceful purposes, not for weapons.
So, this – these are the two – the two aspects of alchemy in nuclear reactors.
[slide titled, Actinides – Nuclear Non-proliferation, Waste and Fuel, featuring three pie charts for the amount of naturally occurring uranium, low enriched uranium and highly enriched uranium along with a smaller version of the periodic table from the previous slide]
We produce radioactive elements that we need to manage, both in terms of the fact that they produce heat and in terms of the fact that they produce radioactivity that can damage our bodies. And then theres this dimension of peaceful uses of nuclear technology which we need to ensure and demonstrate.
And the International Atomic Agency is an international agency whose role is to ensure that nuclear energy is used for peaceful purposes, and to ensure that countries that do not already have nuclear weapons will not develop nuclear weapons, and to also help countries that do not have nuclear technology develop that technology, so that the world has access –
[Raluca Scarlat]
– to this technology, so, both the developed and the developing countries have access to this technology if they so choose to.
And the I.A.E.A. has two roles. One role is to help in the training and the – the training of new countries that wish to learn how to use this technology and the deployment of this new technology to new countries. And then the other role is verification that the technology is being used for peaceful purposes.
What is not within the I.A.E.A.’s role is ensuring safety. And thats an important – thats an important dimension when we think about developing of nuc – develop – development and export of nuclear technology, because every country is sovereign, so every country is responsible for ensuring the safety of their technology.
But as an exporter of nuclear technology, we are the most knowledgeable of the technology that were exporting. And so, we have a certain amount of responsibility to ensure that the country that were exporting to has really developed their technical know-how to responsibly ensure the safety of – of their reactors. And at the same time, that country is sovereign, so they – they make their own decisions as to what they consider acceptable risk and unacceptable risk. And maybe their decisions are different from ours. Maybe their decisions in their particular context are more risk-taking than ours or less risk-taking than ours. So, there it becomes an interesting fine balance between how much it is the responsibility of the exporting countries to ensure and verify the safety of the technology that theyve exported.
And an interesting fun fact – the reactors at Fukushima were exported by the U.S. by G.E., in collaboration with – with Japan. And, of course, the nuclear – the – the international nuclear community aided Japan in the response or offered to aid Japan in the response to the accident, but ultimately, it was Japans responsibility to manage the economic and the environmental and the human impacts of that accident. And so, one might ask whether theres also a moral responsibility that the U.S. might have carried over decades in exporting that technology to Japan. And its a difficult question to ask because were talking about spanning decades, spanning generations. So now were talking about responsibilities that span from one generation to another. And that is a difficult conversation to have but not an impossible conversation to have.
So, with that, intermission.
Why cant nuclear grow – both in the U.S. and internationally?
Any thoughts or guesses?
Yes.
[female audience member]
Nobody wants a plant in his backyard.
[Raluca Scarlat]
Nobody wants a plant in their backyard. Why not?
[same female audience member]
Theres danger there. It’s a risky business.
[Raluca Scarlat]
Because theres – because theres a perception of danger. What do we mean by danger?
[same female audience member]
Radioactivity.
[Raluca Scarlat]
Okay, so theres – theres a perception of danger because there might be radioactivity from the plant that reaches the houses surrounding.
[return to the Actinides slide]
[Raluca Scarlat, off-camera]
Yes?
Because the timeline – because of the time – timeline of commercialization and construction is very long, so it relies on government involve – involvement –
[Raluca Scarlat, on-camera]
– for development of technology, and thats challenging, and maybe there isnt a policy to support that quite directly.
Yes?
[male audience member]
The cost of decommissioning one more nuclear plant, it seems the costs were never really anticipated, and they keep rising; thats what I read in the papers, especially in Germany, they have that problem.
[Raluca Scarlat]
So, the cost of decommissioning a plant that was built 40 years prior and the uncertainty around it and the duration of – of that decommissioning process is a question mark. And when one deploys a new technology, one wants to know that its reversible. If we dont like it, we want it to go away quickly, and so we want to have that option with nuclear as well.
Yeah, thats a good point.
Okay, take one more.
[second female audience member]
No long-term storage facilities for radioactive waste.
[Raluca Scarlat]
No long-term storage facilities for radioactive waste. That is, to some degree – from a – from a policy point of view, that is correct. From a technology point of view, that is not correct. So, its –
I mentioned that the fuel can be recycled. If we choose to recycle spent fuel, it is not a good option to put our current used fuel in deep geologic repositories from which retrievability is expensive or impossible. If we choose to not recycle it, then disposing it in a deep geologic repository, either a large repository or a deep bore hole system, is a choice that needs to be made.
We do have waste, nuclear waste disposal sites around the country. We do not have disposal sites for used fuel. And it is – it is a policy decision that the government needs to take. It is not a lack of a technological solution. And to give you a little bit more background into why this policy decision is challenging, the government took the responsibility to manage the spent fuel from the industry. So, when the industry was developing, the government said, We will help you in your development. So, we will take the responsibility of managing your used fuel if you pay us a tax. So, a tax on the electricity that they sold – that utilities sold was collected by the government. And they did not meet their – the government did not meet its contract with industry to take the fuel and manage it in a centralized fashion. And now the tax is no longer levied. There is still a large fund of over $10 billion that the government has for the – for the management of the used fuel. And now its a manner – its a matter of setting up the institutions that can manage that fund and making policy decisions that enable the usage of that fund.
And theres an interesting report called the Blue-Ribbon Commission on the Future of Nuclear Fuel in the United States, if youre interested in reading more about the policy decisions behind managing spent fuel.
There are also interim – what we call interim storage facilities that are in the process of being licensed in the United States. And this is a relatively new – new development, and what we mean by an interim storage facility is a centralized facility that collects the used fuel from all of the sites throughout the United States, and then from there, the fuel can be taken for a longer-term usage, or for recycling. And I – I do need to explain what I mean – what is meant by longer-term usage.
The radioactive – Im going to go back to the alchemy slide. The radioactive elements that are produced –
[return to the Alchemy slide featuring the periodic table]
– they will reach a stable state, within – some of them within a couple of seconds, some of them within a few minutes, some of them within a few days, and most of them, among the lighter elements, within tens of years to hundreds of years. Designing a repository for hundreds of years is not unlike other technologies that weve – that – that – that we manage. There are buildings that have existed for hundreds of years. We – we can handle that.
As a – as a society and as a culture, hundreds of years does not seem daunting. However, the elements at the bottom that are being produced by absorption of neutrons, so the heavier the elements – the elements that are heavier than uranium, those are not very highly radioactive. They dont produce a lot of heat. But because theyre not very highly radioactive, they take a long time to decay away to something stable. So, theyre not highly radioactive. They decay slowly. They take a long time to reach a stable state. And a long time means millions of years. So, maintaining the integrity of anything for millions of years is daunting for our – for human civilization.
Now, the majority of the waste that is produced by nuclear power is here, is not the bottom. So, if we were to recycle the fuel, one, we could use these heavy elements for power production because we can split the atom. And two, even if we didnt use it for power production, if we just separated out the heavy elements, then we would condense the waste quite a bit, so it makes the management cheaper and easier. So, those are the options for recycling.
Yes?
[male audience member]
Do I remember you said one of recycling problems is the danger of proliferation of – of weapons problems?
[Raluca Scarlat]
Correct, because if we recycle, were separating out elements from our waste fuel, and one of the elements we could separate is plutonium, which is a proliferation risk that plutonium would then be used for weapons. So, it’s a complex – it – there are many dimensions to managing this technology. Its not good nor bad. Its – there are many layers to it.
There are also many technological fixes. So, the question is, Can we find the combination of institutions and technology to ensure that – that nuclear power –
[Raluca Scarlat, on-camera]
– is responsibly explored because it doesnt just take the technology, it also takes the institutions that oversee that the right technology is being used in the right way. And thats, in a way, unique to nuclear technology, that we cant just think of technological fixes. We have to think of technology in the context of institutions.
[return to the Alchemy slide featuring the periodic table]
So, if we look to recycle nuclear fuel, we would want to extract only the heavy elements for energy production, and the lighter elements could be extracted for precious metals or for medical applications. Molybdenum, for example, can be used for medical applications. There are – theres actually, in the state of Wisconsin, a nuclear reactor thats being built specifically for the nuclear application of producing moly-99. So, is there a commercial case for recycling? And the short answer is not yet, because uranium fuel is relatively cheap, so recycling is not necessarily economically profitable.
But as the cost of energy fluctuates and the cost of uranium fuel fluctuates, we may get to a point where it is economically profitable to recycle some of the waste. And I – I mentioned the price of energy in the equation because it takes energy to produce fuel. So, the cost – a big part of the cost of nuclear fuel is energy. Its not just the uranium ore that costs.
[Power point presentation advances to a slide titled, Intermission – Why cant nuclear grow?, featuring the bullet points of motivated people, cost, slow innovation, supply chain, and cost]
So, but theres another dimension – theres another dimension to – to the cost of the recycling technology, and that has to do with the roadblocks to innovation in the nuclear field. Why arent we innovating faster? Why arent we cycling through technology faster? Because the plants that we have today, you might have noticed on the map that I passed around, their – most of their ages are 40 years old. And the plants that were building today are not that different from those plants that we built 40 years ago. So, our innovation cycle is very slow. Maybe in 30 years, one – one came up with – so that the – the plants that we build today, we called advanced water reactors, and the advanced water reactors took about 20 to 30 years to develop even though we already had water reactors. So, it was an incremental move, and it took decades to – to evolve.
And so, what are those roadblocks to innovation? And I would say that cost.
[Raluca Scarlat]
The economic competitive – competitiveness of nuclear is part of it. Nuclear is too expensive today, and there isnt enough optimism that we can innovate sufficiently to make nuclear cheaper, which brings us back to motivated people. If we had more optimism and more creative minds working in the nuclear field, then maybe we could work on making nuclear cheaper.
And then the other part of the equation is the supply chain because weve forgotten in the United States how to build reactors, and we do not have a supply chain that would support conventional reactors, let alone advanced reactors. We rely on an international supply chain which is important and valuable but sometimes has its challenges if we want to innovate fast.
And so, in an effort to address the challenge of motivated people, we have a nuclear engineering department here at University of Wisconsin-Madison, and we train new, talented students that we hope will – will find ways to innovate. Theres a nuclear innovation camp that happens every year at Berkeley. Its co-organized together with the University of Wisconsin. And there – theres a lot of research that we do at the University of Wisconsin-Madison on advanced nuclear energy technology, and on finding better ways to innovate faster in nuclear.
And so, one way to innovate in nuclear is to think of can we go away from the pressure cooker and the steam engine? So, that means going away from water as a coolant.
So, what can we go – go to? What can we move to from water? We can look at gas, helium as a coolant. We can look at salt, table salt.
[side with six illustrations of different types of reactors with illustrations for each including, molten salt, lead-cooled, very high temperature, sodium-cooled, supercritical water-cooled, and gas-cooled reactors]
Sodium chloride or fluoride salts, like the potassium fluoride that is – thats in your toothpaste. Those are salts that are solids at room temperature, but if you heat them up, they become liquid and they can be used as coolants. We can look at supercritical water, sodium, so liquid metal reactors. We can look at many, many coolants that could – that could, in essence, bring us to higher temperatures for – for nuclear reactor operations, because water-cooled reactors go up to about 300 degrees Celsius, which isnt very much higher than your oven at home. Its relatively cool for – for power production. And we cannot – we dont yet have the technology to – to operate water-cooled systems at high temperature. And high temperature brings us the ability to operate advanced turbines, like gas turbines that are used in gas combustion, which have higher efficiency, and the higher efficiency translates in a better economic case. And so, all of these – a lot of these technologies would move us towards higher temperatures and higher efficiencies and a better economic case.
[new slide titled, Some Molten Salt Start-up Companies, with a list of eleven companies including, Moltex (U.K.), Terrestrial (U.S. & Canada), Tansatomic (Boston), Thorcon (California), Kairos (California), Elysium (Boston), Terrapower (Bill Gates), TMSR (China), Seaborg (Europe), SAMOFAR (European consortium), and Copenhagen Atomics (Germany)]
So, Ill talk about one of these options for advanced reactors and thats molten salt technology. There is quite a number of startup companies that are looking to commercialize molten salt reactors, both in the U.S., in the U.K., in Canada, and across the world.
[return to the Story-board slide with the outline of the talk and Molten salt reactor technology being point six of seven in her speech]
The reason –
[new slide titled, Fluoride-Salt Cooled High-temperature Reactors, with three illustrations of the design and use of said reactors]
– that theyre of interest to the startup companies is that they can operate at high temperatures, they can operate gas turbines. Gas turbines can operate in baseline, but also, they can co-fire with natural gas for power peaking, which then makes the economic case much more competitive for nuclear and also opens a new market. It opens the power peaking market for nuclear. And so, theres quite a bit of effort to develop molten salt reactors.
Molten salt reactors, in the longer term, can also be used for online recycling of the fuel, so it minimizes the waste and potentially also improves the economics.
[new slide titled, An FHR Plant, with a cross-section illustration of a FHR reactor plant and how it works followed by another slide titled, Molten Salt research at the University of Wisconsin-Madison featuring five photos from studies being done at the University]
And then, in our research group, we work on understanding the behavior of molten salt, their behavior of coolants, and their behavior of solvents for the uranium fuel. So, these are some pictures from the research that we do. What you see here is liquid salt that weve melted on a Bunsen burner. And in it are pebbles of graphite that are floating. And this is a reactor concept. We could put small particles of uranium in a graphite sphere. These graphite spheres could float on molten salt. The molten salt behaves as a coolant, and it transports heat to a gas turbine, and the gas turbine would be an air-breathing turbine. So, an open cycle turbine that breathes in air and releases warm air and produces electricity.
And what is interesting about this reactor concept is that the salt does not boil. So, you cant have a pressure cooker. Its actually an open pot that you run.
So, that means that not having a high-pressure system takes away the risk of having a pressurized system that can disperse radioactivity into the environment. So, even if theres a break in one of the containment systems, there is no source of pressure that could disperse the radioactivity. The radioactivity can more easily be contained. And that has some advantages in terms of demonstrating safety. It also has some advantages in terms of economics because building an open pot is much cheaper than building a pressure vessel.
And so, we do a lot of research in my group on the behavior of graphite in molten salt and on the behaviors of molten salt. We try to understand what do we mean by pH in molten salt and how does that affect the degradation of materials that come in contact with it? We try to understand how viscous it is. What is the viscosity? What is the density? These are all unknowns because its a new fluid that were working with.
[new slide featuring the photos of all the people working on molten salt research at the University of Wisconsin-Madison]
And we – this is our group, our current group of students from U.W. working on molten salt research. And I want to point out that theyre students from many fields, chemical engineering, material science, physics, nuclear engineering, mechanical engineering, physics, chemistry. It takes quite a diversity of fields to tackle questions in nuclear engineering.
And this is a picture taken in our laboratory with what we call a glove box. Its a box with gloves. And the box is sealed, so theres no air inside. Its – its an inert atmosphere, and that allows us to maintain the purity of the salts and study them in an environment similar to what they would be like in a reactor.
[Raluca Scarlat]
And so, with that, Ill end with a question to the audience, which is, would you help nuclear grow, and how would you help nuclear grow? And then, with that, Ill take questions.
[applause]
Search University Place Episodes
Related Stories from PBS Wisconsin's Blog

Donate to sign up. Activate and sign in to Passport. It's that easy to help PBS Wisconsin serve your community through media that educates, inspires, and entertains.
Make your membership gift today
Only for new users: Activate Passport using your code or email address
Already a member?
Look up my account
Need some help? Go to FAQ or visit PBS Passport Help
Need help accessing PBS Wisconsin anywhere?
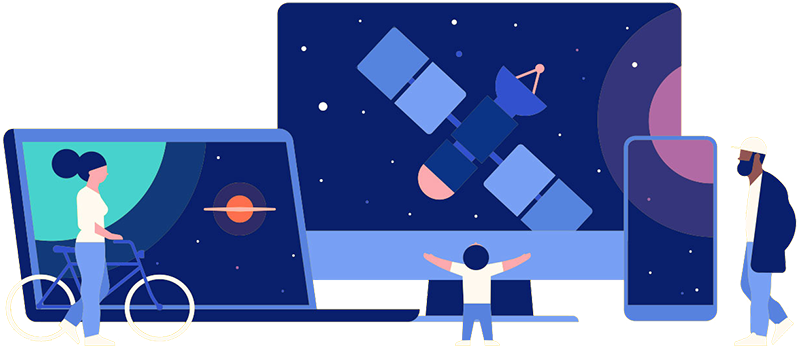
Online Access | Platform & Device Access | Cable or Satellite Access | Over-The-Air Access
Visit Access Guide
Need help accessing PBS Wisconsin anywhere?
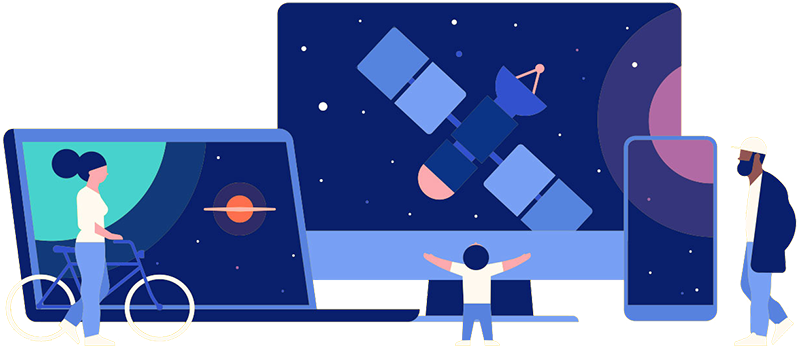
Visit Our
Live TV Access Guide
Online AccessPlatform & Device Access
Cable or Satellite Access
Over-The-Air Access
Visit Access Guide
Follow Us