Hello, and thank you to Wisconsin PBS for the opportunity to speak today.
My name is Minhal Gardezi, and I’m a graduate student at the University of Wisconsin-Madison in the physics department.
I took my first class in physics ten years ago as a sophomore in high school, and from then on, I knew I wanted to study it for the rest of my life.
I ended up taking two and a half years of physics classes in total in high school, and then went on to major in physics and computer science in college.
I then did a research fellowship in physics for a year before beginning my physics PhD here in Madison.
And even though it sounds like I’ve been pretty single-mindedly glued to physics for my whole life, I’ve actually always been interested in other fields.
Even though I took and enjoyed every STEM class in my high school, my favorite classes were actually in the humanities.
And for that reason, I chose to go to a liberal arts college, where even though I double majored in STEM, I was also guaranteed the opportunity to study things like English, poetry, history, religion, all fields that are typically divorced from STEM.
But I’ve never really liked that seeming division in the first place, this idea that you’re either very scientific and mathematical and robotic or you’re very artistic and dreamy and human.
Surely you’re allowed to be both at the same time, and surely incorporating both into what you do is beneficial to both realms of study.
Coming into graduate school, I knew it was going to be the time to hyperfocus down into physics, but I still wanted to do research in a way that incorporated all the things I learned from my non-STEM education, things like artistic creativity and effective communication and cultural understanding.
At Madison, I received the opportunity to do interdisciplinary physics research.
And from a typical physicist perspective, interdisciplinary usually means crossover with other STEM fields like chemistry or biology, material science or engineering.
But the research I’ve gotten to do is interdisciplinary in a way I never really encountered before, as it has crossover with the humanities.
I conduct cultural heritage studies using X-ray spectroscopy.
Cultural heritage refers to the remnants of humanity that build up our cultural identity.
It includes tangible things like artifacts and buildings as well as intangible things like language and folklore, and even natural things like landscapes or biodiversity.
Cultural heritage studies allow us to understand how our past led to our present.
It can help us connect to our roots and even reveal roots that we didn’t know existed, as well as ties between different cultures and centers of knowledge.
Cultural heritage studies are typically conducted by historians and anthropologists, and in general, people who are scholarly experts in specific cultural histories.
Cultural heritage artifacts are of particular interest.
These are things like artwork or documents or fossils, things that you might typically find in a museum.
These artifacts tend to be highly protected, as they’re often the only tangible connection we have to our past.
However, while historical and cultural studies are hugely important to understanding these objects and understanding our past, there’s only so much of that type of information available.
Eventually, scholars will hit a block where there is no longer any additional hard information to be extracted by human means alone.
And that is where physics comes in.
Spectroscopy is a study of how light interacts with matter.
And when light is in the form of X-rays, we refer to it as X-ray spectroscopy.
In my research, I take those cultural heritage artifacts and I study them using X-ray spectroscopy.
We can think of these artifacts as chemical time capsules that can be opened up using X-rays.
X-rays allow us to see beyond the limits of our eyes, and they generate hard scientific data that can help aid cultural studies to uncover new information about our past.
Simply put, I blast artifacts with X-rays and learn new things about the old things.
And today, I’d like to walk you through what it is I actually study, and in doing so, illustrate just how meaningful this type of interdisciplinary work can be.
Beginning on the cultural heritage side of things, my research aims to uncover more information on early print.
And here, print specifically referring to the process of creating documents through mechanical means.
And when I say early print, I’m referring to the first instances of these printing machines, which were printing presses.
These presses used what is called movable type to mass-produce documents.
And the way they work is you cast individual letters or entire words in metal, and then you arrange them to form a page.
And then you ink that page and press a paper down onto it, effectively printing a single document.
And you can print that document over and over and over again to produce multiple copies, and then rearrange all of your types to produce another page until you’ve effectively mass-produced an entire book.
Now, when I was told I would be studying early print, this was about the extent of my knowledge.
I knew how the printing presses worked, but I didn’t know much in the way of cultural heritage.
So to broaden my knowledge, I turned to the place where all great research endeavors begin, which is, of course, Wikipedia.
[audience chuckling] On the page for printing press, one thing immediately jumped out: the name Johannes Gutenberg.
It’s a name familiar to me, and maybe you recognize it too.
I remember learning it in school that Gutenberg was the father of the printing press or the inventor of the printing press, and he printed these famous Gutenberg Bibles.
But once I actually started reading the page, something else jumped out at me: this sentence right here at the end of the first paragraph, which reads, “Typically used for text, “the invention and global spread of the printing press “was one of the most influential events in the second millennium.”
That’s a pretty bold statement to make, and it surprised me.
Not because I didn’t think it was true, but because I’d never really considered just how important the printing press was.
In fact, if you google “most important inventions,” you’ll find the printing press always makes the list.
And again, there is no mention of the printing press without Gutenberg’s name attached to it.
At this point in my research, I had two main questions.
Why was the printing press so important, and what exactly was Gutenberg’s role?
To answer those questions, I had to dive deep into the history of print.
And I’ll lay out what I learned here.
So here’s a basic timeline, and for reference, here are the centuries encompassing the European Renaissance.
Johannes Gutenberg created his famous press near the beginning of the Renaissance.
He printed his first 42-line Gutenberg Bible right around 1450 in Mainz, Germany.
As soon as those Bibles entered Europe, use of the printing press spread like wildfire.
Craftsmen around Europe recreated and modified Gutenberg’s press, developing their own printing centers.
And in just 50 years, an estimated 20 million volumes were printed.
By the end of the Renaissance, it had increased to 200 million.
The press outproduced hand-copying by so much, it wasn’t even a contest.
And this led to mass communication, and as a result, a boom in literacy.
So it’s not just that Gutenberg invented his press near the beginning of the Renaissance; rather, it’s more like Gutenberg’s press is one of the causes of the Renaissance.
Mass-produced print made education more accessible to lower class communities.
It allowed for the spread of news, leading to social organization and even revolution.
The average person now had more knowledge and therefore more power.
The printing press revolutionized the dissemination of information, and in that way, it’s very comparable to the modern invention of the internet in terms of its impact, especially in how immediate and widespread that impact was.
Following the Renaissance, the press made its way to colonial America, and then together, the Western powers entered what is known as the Industrial Revolution.
During this time, there was a huge transition away from hand-powered printing presses towards steam-powered rotary presses, which were much more powerful.
But even though the presses were evolving a lot, they still employed Gutenberg’s fundamental printing principles.
Gutenberg’s direct influence remained all the way up until the late ’80s, when we saw a transition towards digital print, which is what we use today.
And the field of print continues to advance every single day, even into the third dimension with 3D printing.
But even though the nature of printing is evolving far beyond Gutenberg’s press, he was emblematic of the spirit of human ingenuity and the development of new technology for greater accessibility of knowledge.
All together, Gutenberg’s impact was profound, and there is no question that his press completely changed the world.
However, Gutenberg’s printing press is only a piece in the larger history of print.
He was a key figure, no question, but to call him the inventor of print is a little misleading.
The first books he printed were the Gutenberg Bibles.
And although many of them remain preserved to this day, the oldest extant document printed on a movable metal type press that we have preserved is not one of those Bibles.
It was not printed on a Gutenberg press and it wasn’t even printed in Europe.
It was printed on the other side of the world.
It is a Buddhist text called Jikji printed in Cheongju, Korea in 1377, nearly 80 years prior to Gutenberg’s Bibles.
And in fact, that 80-year gap is just the tip of the iceberg.
There is a rich history of East Asian printing that stretched hundreds of years before even the timeline shown on the screen.
And it should be noted that while this pre-Gutenberg printing existed throughout East Asia, and particularly in China, I’m gonna focus specifically on Korean printing, like the printing of the Jikji.
Before the advent of the metal type presses that printed the Jikji, much of the early East Asian printing utilized woodblocks.
There were these big slabs of wood into which entire pages were carved at once.
And sets of these woodblocks have been very well-preserved to this day.
Like the Jikji, many woodblock printed documents were Buddhist texts, but there were also woodblocks used for the daily history keeping and record of everyday life.
And it’s in one of those preserved woodblocks that we actually see the first mention of movable metal type presses being used in Korea back in 1234.
So that is nearly 200 years before Gutenberg’s press was made.
Any documents potentially printed in Korea between 1234 and 1377 have not yet been discovered, if they exist.
And the Jikji remains the oldest extant document printed on a metal movable type press.
But it wasn’t until the end of the 19th century when a French diplomat acquired Jikji and brought it to Paris that the Western world at large was even made aware of East Asian printing.
It was donated to the Bibliotheque nationale de France, and finally in 2001, it was declared a UNESCO Memory of the World.
Jikji has remained in the BNF to this day.
It’s actually sitting there right at this moment.
And in fact, just two weeks ago, the Jikji was brought out for display for the first time in 50 years.
It’s a very highly protected document.
Just looking at the red and blue here, we can summarize the history of print with two big facts.
One, the Gutenberg press had a much larger impact on the world than the Korean press.
But two, the Korean press undeniably came first.
Now, if you didn’t know about this Korean printing history, you’re not alone.
Not many people outside of East Asia have any knowledge that printing presses existed before Gutenberg, much less that there is such a rich printing tradition throughout East Asia.
This trend of knowledge indicates that the Gutenberg press had almost a global impact, whereas the Korean press had much more of a local impact.
There are several reasons why this could be.
The first is the context of the invention.
Before Gutenberg, Europeans predominantly relied on handwriting to create documents.
The invention of movable type print specifically for producing documents was revolutionary.
But in Korea, investigations into different means of printing and even papermaking and inkmaking had been done extensively for centuries.
Movable metal type was an invention that pushed the boundaries, creating a new frontier in a field that was already extensively explored and adopted in everyday life.
In Europe, there was almost an immediate widespread adoption of movable metal type printing, while in Korea, both metal type and the preexisting woodblock method were used.
Another major factor is the difference in language.
Gutenberg was using the 26-letter Roman alphabet, and the cost and labor of casting hundreds of copies of each character was well worth the benefit of the mass production of print.
But early Koreans, on the other hand, were using a Chinese-based script, which contains tens of thousands of characters.
It required significantly more metal to make a full typeface and it may not have been so justifiable to divert so much metal to the cause at the time, especially considering the need for metal weapons during the wars of the Goryeo dynasty.
The much simplified 24-character Hangul alphabet, which is used today, was developed back in 1443 in an effort to increase literacy.
However, it would not be widely adopted for another 500 years.
And for this reason, although metal movable type was an ingenious invention in Korea, there was no direct urgency behind a widespread transition to that movable type as there was in Europe.
Overall, each printing press was developed under completely different conditions and went on to have completely different impacts.
Nonetheless, each one is an example of human ingenuity, and it’s really something to marvel at, that two completely different peoples on completely different sides of the world arrived at the same type of technology.
However exciting these inventions may be, much remains unknown about them.
In terms of overall knowledge, very little is actually known about the Gutenberg press.
There’s no official documentation of Gutenberg’s design process, no record of his notes, and no record of even what the press itself looked like.
There are some artistic depictions that were made many, many, many years later, but no original hard documentation of his work.
On the other hand, there is extensive documentation on the Korean printing tradition, dating back hundreds of years before even the life of Gutenberg.
This is documentation on how these presses were set up, how the pages were made, how the paper was made, how the ink was made.
There’s a lot of knowledge.
When it comes to preserved artifacts, there are no remaining original presses from either printing center.
There are also no preserved types from the Gutenberg press, meaning we actually have no idea what metals Gutenberg used to cast those types, or how he arrived at their production.
There are, however, many early Korean types preserved today, although it is unclear if the earliest ones, like the ones used to print the Jikji, have been discovered.
These early types are understandably very important and protected objects, and are therefore difficult for us to gain access to, at least at this stage in our research.
Many of these types are preserved in Korea, particularly in Cheongju at the Cheongju Printing Museum.
But there are some that exist in other parts of the world.
And for example, the Library of Congress actually has a pretty substantial collection of these early Korean types.
When it comes to prints though, those are much more abundant.
Many of the original Gutenberg Bibles are printed to this day, and are sort of scattered all over the world in different libraries.
And similarly, many early Korean prints of all kinds have been preserved, including the famous Jikji, which is in the BNF.
It is through studying these prints using X-ray spectroscopy that we hope to uncover more information on early print and potentially fill in some of those other gaps in our knowledge.
If it is possible to learn about the presses themselves from these prints, we can gain a lot more insight into the inventive pathways going on back then.
And it may be even possible to tackle even bigger unknowns, particularly in the question of connection.
Was there any type of knowledge transfer between East Asia and Europe that led to Gutenberg’s invention, or were the two inventions truly independent?
This is all…
These are all questions that my research seeks to answer, contained in the main question, can X-rays trace the origins of printing?
To answer this question, my PI and I are part of a larger UNESCO-funded collaboration called “From Jikji to Gutenberg.”
It brings together print scholars from across Europe, Korea, and America.
On the team are paper experts, ink experts, type experts, conservationists, librarians, private collectors, over 50 people, including two physicists, my PI and myself.
Through the collaboration, we have gained access to a wealth of historical documents, namely individual Western and Korean leaves, and most notably, leaves of an original Gutenberg Bible printed in the 1450s, and an entire bound Confucius text printed in Korea about a decade earlier.
It should be noted that despite the collaboration’s name, “From Jikji to Gutenberg,” we don’t actually have access to the Jikji itself, as it is a hugely important artifact held under lock and key.
Nonetheless, these documents that we did scan are priceless cultural heritage artifacts well worth examining for the advancement of our overall cultural heritage understanding of early print.
So, and with that, we should be caught up on the cultural heritage side of my research, which means we can move into the science part.
As I mentioned before, I use a technique called X-ray spectroscopy.
And I would like to introduce you all to X-rays and the specific technique of X-ray fluorescence.
X-rays are one of many types of electromagnetic waves included on the electromagnetic spectrum.
Electromagnetic wave is just a fancy way of saying light.
And it comes in many different forms, depending on its energy and wavelength.
Wavelength is a measure of the physical distance between the peaks of a wave.
The shorter wavelength electromagnetic waves have higher energy, and the longer ones have lower energy.
Each type of wave carries a certain type of information and can be picked up using a certain type of detector.
For example, starting on the left here at the longest wavelength and lowest energy, we have radio waves.
These waves carry sound information and are detected by things like radios and televisions.
They have wavelengths on the order of miles, and have such low energy that they don’t really affect any organic life they may come into contact with.
Beyond that are microwaves, which can carry signals detected by telephones, but are most commonly used in microwave ovens.
These waves have just enough energy to jostle the electrons in your food so that it heats up.
The energy is still relatively low though, which is why it’s recommended to use a rotating plate and to mix your food in between microwaving so the waves can thoroughly reach all parts of your food and heat it up.
In the center of the spectrum here, we have visible light.
As the name implies, these are light waves that humans can see.
The waves carry information on the color and appearance of matter in the world around us, and our eyes serve as the detectors.
The lowest energy visible light is red, and just below that is infrared light.
Infrared waves are emitted by sources of heat like the Sun or fires, and they carry information about how hot that heat source is.
Some animals actually have eyes that can detect infrared light.
That is, they can see heat with their eyes.
But humans cannot do that.
But there are ways to shift up that infrared light into the visible spectrum, allowing us to see heat signatures.
The highest energy visible light is violet, and just beyond violet is ultraviolet.
Ultraviolet or UV light is emitted by high-energy sources like stars.
And although we can’t see it with our eyes, we are exposed to UV light every day from the Sun.
UV light is the beginning of the relatively high energy side of the electromagnetic spectrum.
And while a single UV wave does not have enough energy to do that much impact on organic matter, prolonged exposure to UV light can do damage, which is why it’s very important to wear sunscreen and sunglasses, both of which protect from UV exposure.
At the far end of the spectrum are gamma rays, which are incredibly dangerous and are only used in extreme circumstances, as they can be highly damaging to organic matter.
But in the sweet spot between UV and gamma rays are X-rays.
X-rays are high enough energy to penetrate matter.
For example, X-rays are commonly used by doctors to see inside you.
They have enough energy to penetrate through the squishy things like your organs and your blood vessels, but are not energetic enough to penetrate through dense things like bone or metal.
Doctors will expose you to controlled, low energy X-rays very briefly to generate images of your skeleton and also any metal that might be in your body.
Areas of the body not meant to be scanned are usually covered in lead-lined vests.
Like when you go to the dentist, they put a big vest on your chest because that lead vest will block any potential stray X-rays.
And a similar and more rudimentary setup is used at airport security checkpoints to search for any metal in your clothes or belongings.
However, X-rays that are used on humans are what we call soft, as they use the lowest possible energy to get the job done while minimizing any risk to a person.
X-rays in general can have much higher energies, high enough that they can interact with the subatomic particles that make up matter.
These high energy X-rays, called hard X-rays, have accordingly shorter wavelengths, actually down to the scale of individual atoms.
This means that because of their…
Sorry.
Together, this means that X-rays, when shown on matter, can reveal very high-resolution information on the atomic scale.
And it is those hard, high energy X-rays that I work with as an X-ray spectroscopist.
To generate those high-quality hard X-rays for use in experimental setup, we have to go to a facility called a synchrotron.
And here’s a schematic of a typical synchrotron facility.
A synchrotron is a system of circular particle accelerators that form a radiation light source.
And this image here is actually the logo for a synchrotron called SSRL, or Stanford Synchrotron Radiation Light Source.
Now, those are all some pretty cool-sounding words I just said, but in English, a synchrotron generates very high energy light like X-rays that can be used in a controlled matter for experiments.
The way it works is first, there’s a smaller booster ring that takes electrons from some material and accelerates them around in a circle.
Using electric and magnetic fields that are timed precisely, the electrons are brought up to a fraction of the speed of light, which is very, very fast.
Those sped-up electrons are then fed into the much larger storage ring.
Again, electric and magnetic fields are applied, but this time, they’re synced up to the electrons in a way that keep them moving at the same speed, this very, very high speed.
When very low mass charged particles are moving very fast, like these electrons, they must send off energy in order to change direction.
You can think of this as kind of a conservation of energy, momentum situation.
In order to turn one way, the electrons need to push the other way, and their push is in the form of released light, specifically X-rays that are released radially outward.
These radially projected X-rays are caught at several points along the ring and funneled into separate, high-quality X-ray beams, which are here represented by all those arrows.
Scientific workstations called beam lines are constructed all around the storage ring to utilize those X-ray beams for experiments.
At a typical synchrotron, each beam line is optimized for a different type of spectroscopic phenomenon.
When it comes to X-rays hitting matter, several different physical phenomena occur.
But my research involves a particular one called X-ray fluorescence or XRF.
To explain XRF, I’m going to use this rudimentary model of an atom where the nucleus of the atom is shown in red and the electrons orbit around it in shells.
Now in reality, electron behavior is a little more complicated and abstract than this, but I’m going to use this very simple model to explain.
The closer an electron is to the nucleus, the more energy it takes to pull that electron away.
And that energy is actually on the same scale as X-ray energies.
In X-ray fluorescence spectroscopy, first you expose an X-ray to the sample.
It hits that atom and it transfers its energy to the atom.
And the X-ray’s energy is powerful enough to actually eject a core electron from that atom.
It knocks it out, leaving behind a hole in that inner shell.
By the rules of electron arrangement, that hole must be filled, so a nearby electron drops down from its shell to take the ejected electron’s place and fill that hole.
This backfilling electron is moving from a higher energy state to a lower energy state.
So in order to make that backfilling action, it must get rid of some energy.
It does this by releasing an energy packet called a photon.
A photon is the particle version of light.
And the release of photons is called fluorescence.
In this case, the photon will be high enough energy that it is also an X-ray, hence the name X-ray fluorescence.
All together, we shine X-rays on atoms, which causes them to fluoresce X-ray photons back out.
But why is that useful?
Well, if you actually look at the photon, it’s not just that it was released because the backfilling electron had to get rid of some energy to drop down.
That photon is in fact actually the exact energy difference between the original shell of the electron and the backfilling shell, the shell that it moved to.
So if we measure the energy of that photon, we are going to actually measure the exact energy difference between those two shells.
And that is significant because if we look at the periodic table, the factor that distinguishes an atom of one element from another is its atomic number, which is the number in the top left.
And that is actually the number of protons that are in the nucleus.
So the more protons that are in a nucleus, the more positive that nucleus is and the larger that nucleus is, meaning the more pull it will have on those electrons.
And from element to element, that nucleus will grow larger as we move down the periodic table, but the electrons stay the same.
What this means is that the difference in energy of those first two shells of an atom is going to be different depending on the element we’re looking at.
It’s going to be a unique value for every element.
In other words, that photon is going to have an element-specific energy.
So if we measure the photon that is released after exposing an atom to X-rays, the photon can actually tell us exactly what type of element that atom belonged to.
The entire XRF pathway allows us to determine the elemental makeup of a sample.
We hit it with X-rays, measure the fluorescence, and determine exactly which elements were in the spot that we just hit.
Once we’ve collected the fluorescence, we create what is called an XRF spectrum.
On the x-axis is the fluorescence energy, and on the y-axis is the intensity, which is a measure of the number of photons that we recorded.
A peak on the energy axis indicates the presence of a specific element that are labeled above those peaks, whereas the height of the peak on the intensity axis tells us relatively how much of that element was present in the sample where we hit it with an X-ray.
In the sample that created this spectrum, for example, there was a pretty large amount of calcium, iron, and copper, but lower amounts of other elements like manganese.
These spectra are the type of data that I most commonly work with.
And while these spectra hold very valuable information, it can take some time to develop the intuition to interpret these peaks.
So if this image is a little confusing to you at this point, don’t worry; it’s understandable.
However, there is another exciting application of this spectroscopy method that can yield much more intuitive and beautiful data, and that is elemental mapping.
To illustrate elemental mapping, let’s use this example of a piece of parchment.
This is the sample that we’re gonna be studying with X-rays.
And that image there is a camera image.
It’s what you actually can see if you were holding this parchment in your hand.
We want to determine which elements are in the parchment and if there’s any significance to those elements.
So we will use XRF spectroscopy.
We aim an X-ray beam at a point on the paper and measure the fluorescence that comes off, and produce an XRF spectrum that tells us exactly which elements are sitting at that point.
However, this single spectrum does not encompass the data for the entire sample, nor does it give us any sense of the spatial distribution of these elements.
Instead of taking a single point of XRF data, we can instead raster scan the sample behind the X-ray beam, like this animation shown here.
Here the yellow beam is the X-ray beam, which hits the sample and produces the fluorescence shown in red and green.
And the detector on the left continuously collects that fluorescence signal.
A computer program then connects the collected signal to the location at which the scanning beam produced that signal.
It allows us to effectively take an X-ray image of the sample.
For each pixel on the sample, there’s an entire XRF spectrum like the one showed here.
We can then take the grid of pixels and filter each pixel to only display the relative amount of one element, generating an elemental map.
In other words, a spectrum shows the relative amount of all the elements at a single pixel, but a map shows the relative amounts of a single element at all pixels.
Here, for example, is an iron map for the parchment, where higher signals of iron are shown in white and lower signals are darker.
Now, this map may look like a picture, but it is formed from pure X-ray data.
And it represents something far beyond the capabilities of a camera.
In fact, if you look closely here at the top of the map, you can actually see that it reveals some text going along and across the page.
This parchment is actually a piece of a larger text called the Archimedes Palimpsest.
A palimpsest is something that once contained writing or artwork, but was later effaced or covered up to display something new.
Here, the parchment was effaced to display religious texts and images, which is what we see on the left there and what we see if we were to look at the document with our eyes.
But a few hundred years before that was done, the parchment actually contained a copy of Archimedes’ original notes.
Using XRF elemental mapping, those notes were uncovered, restoring several of Archimedes’ works thought to be lost.
This research was actually conducted almost 20 years ago by my PI, and it was very influential in cementing XRF as a valuable cultural heritage tool.
XRF spectroscopy and elemental mapping have similarly been used for many different cultural heritage studies.
For example, my PI also studied the Galen palimpsest in the middle here.
Galen was a 2nd-century Greek physician whose work hugely influenced Western medicinal practices for many centuries.
This is a palimpsested copy of his notes written in Syriac, once again revealed using X-rays.
My PI also studied an intact skeleton of an archaeopteryx, which is the dinosaur predecessor of all birds.
He used X-rays to learn more about what this animal looked like when it was alive, including the color pattern on its feathers.
There are countless other examples of cultural heritage studies done using X-ray spectroscopy.
It is clearly an effective method to access information inaccessible through other means.
What’s extremely important to note, however, is that this research isn’t conducted by physicists alone.
Experimental decisions and data analysis are all informed by the knowledge of scholars who are experts in the preexisting cultural heritage of these objects.
This collaboration is essential to generating new knowledge.
And with that, we are all set on the cultural heritage and the physics side of my research, and we are ready to discuss the actual experiment conducted on early print.
So as an overview, kind of taking everything I had just discussed and putting it all together for my experiment, we begin by acquiring several early print artifacts.
Then we bring them to a synchrotron, specifically the Stanford Synchrotron Radiation Light Source I mentioned earlier, which is in Menlo Park, California.
Then we conduct XRF spectroscopy and elemental mapping on the prints.
Then we work with scholars through our collaboration to interpret that data and hopefully generate new knowledge.
We began our experiment last July, in July of 2022, with a week-long experiment at SSRL, studying several of these documents.
We have since had a second experiment in December of 2022, and have had several meetings with our collaborators to discuss our results.
Here’s what SSRL looks like from above.
It’s actually pretty spot-on to that logo.
And our work was done in beamline 7-2, which is actually right here in that little circle.
Here’s an image inside the beamline.
This giant blue door is the gateway into the hutch where the X-ray beam and experimental setup are.
The door and the glass window pane on the door are all lead-lined to prevent any stray X-rays from passing through.
But in general, there are a lot of safety protocols going on here and the entire setup is overall very safe.
Inside the hutch is our XRF scanning setup.
In this image, there’s a document mounted in that metal plate back there.
All of our samples were actually specially mounted to fit that metal plate by Stanford Library conservators.
The mounting process was an aspect of this research I didn’t even think about going into it, and I’m very grateful to the librarians who did all the heavy lifting for us.
But when it came to the actual bound texts like the Gutenberg leaves and the Confucius texts, we had to get a little more creative in the mounting process.
Here, my PI and I are testing out ways to safely mount the Gutenberg Bible leaves.
And we then checked our method with one of the conservators in the beamline hutch.
These documents that we were working with are over 600 years old, so the careful handling of those documents was definitely a priority when conducting these experiments.
Here’s a closeup on the sample mount in the hutch, and that’s actually the Confucius text I mentioned earlier mounted in there very carefully.
Coming in from the right is the X-ray beam, coming out from the wall from the synchrotron and funneling through that sort of pie-shaped appendage.
It hits the sample, the sample fluoresces, and the detector on the left measures the fluorescence.
Neither the beam nor the detector move during an experiment.
They stay fixed in place and the sample on its mount moves behind them.
Here is an example of one of the elemental maps for this scanned document.
Here, red indicates a high signal of the element while blue is low, the meaning that this particular element is very strongly present in the printed regions of this document.
Now, you may look at this image and think, “Wow, that’s a really pretty picture.”
And that’s certainly what I was thinking last July back at the beamline when we were scanning for the first time and this image slowly appeared on our computer screen.
It wasn’t until I turned around and saw all of the beamline scientists standing there with their jaws on the floor that I actually really understood the importance of this image.
Think back to the Archimedes palimpsest.
In this instance, the goal here was to determine if there was underlying text beneath this top image and if X-rays could reveal that text.
The underlying text was believed to be written in iron gall ink, so when they flipped to the iron channel, the text appeared.
But in our experiment, we were not certain X-rays could pick up any trace of the materials used to make the document at all.
This is because we’ve been informed by our Korean scholars in particular that the inks were largely carbon-based and presumably only contained elements that were lighter than phosphorus.
This is important because our specific beamline setup is only suited to detect elements heavier than phosphorus.
So if there are elements lighter than phosphorus, we won’t really see a clear signal for them.
What’s more, we were not examining something like a palimpsest, looking for underlying texts.
We instead wanted to gain as much information as possible on the prints’ chemical makeup in order to determine if there was anything hidden in that data that could give us a clue about the presses themselves.
In essence, we weren’t really sure what we would find, but there was always a possibility that it would be very little.
Instead, we saw beautiful, clear images like this one, showing a strong spatial correlation of elements to specific regions of the document, like in the printed ink versus the paper.
You can even see a little smudge on this map in the bottom left corner corresponding to the spot where people pick up and turn the pages of the book.
So hundreds of years of page turning left a trace that our X-ray could pick up.
To illustrate the sensitivity of our instrument, I wanna show a couple different maps.
And these are of a Korean document printed much later in the 18th century.
This right here is the camera image.
Again, what you would see with your eyes.
There is writing printed in black and then red emphasis circles painted on in red ink.
Here is a map of an element that seems to have been present in both inks used.
And as a reminder, this is pure X-ray data, showing that for this element, it really showed up in these printed regions.
And conversely, here’s a map of an element that was only used in the red ink, and there’s very little signal of it anywhere else on the page.
If we only ever saw this map and not the actual document itself, we would have no way of knowing if any text was actually printed or what was really going on on this because no data showed up for it in the elemental channel.
So I really just wanna highlight that all these images are constructed from pure X-ray data.
It’s completely different from something like Photoshop.
And finally, this is kind of a fun one.
This is a calcium map.
Calcium is present throughout the paper in that green signal, which is to be expected, as usually there’s a lot of calcium in things that have come into contact with water.
But interestingly, this map also reveals these little blemishes on the page.
And you can actually see that top blemish in the visible image if you look back.
I didn’t notice that with my eyes until I saw the X-ray image.
So it’s very interesting that our data can pull out all sorts of random details like that.
We even sometimes see fingerprints in the chlorine channel from the sweaty hands of readers 600 years ago.
All of this, again, pure, hard X-ray data.
The difficulty comes in interpreting why the elements we see are there in the first place.
For example, those red circles actually come from mercury.
That is a mercury map.
And consulting with scholars, that makes sense, as mercury was a known ingredient to turn inks red.
But if we hop back to the map I showed earlier of that Confucius text, as I mentioned, it’s a pretty big deal that we saw such a beautiful, clean image for an element, but it’s actually much more of a big deal than we could have imagined because this is in fact a map for copper.
The reason this is so surprising is because our Korean scholars informed us that they didn’t really believe copper would be a direct ingredient of the ink used to print this document.
Sometimes in some of the Western prints, there are metal ingredients that are used to act as a drying agent in the ink or a binding agent.
But based on the way that the East Asian inks were made, that was often not the case.
Which begs the question, where did this copper come from?
And in fact, in all of the other maps for our Korean-printed documents and most of the Western-printed documents, we continued to see this very strong metal signal, very often in copper for the Korean prints and lead for the European prints.
There is a possibility that the metal could be coming from the ink as it is some type of ingredient in the ink, whether added intentionally or not.
It could be something that is bound to the ink, organic ingredients in the ink.
Or it maybe could have come from the tools that were used to make the ink.
If the ink was, for example, boiled in a copper vessel or some sort of copper tool was used to spread the ink, perhaps that transferred the copper.
But the most exciting possibility for where this ink could have come from is from metal used in the types.
Now, if we could prove that the metal in these documents is from the types, that is a huge discovery.
It means that our question of, “Can X-rays trace the origin of printing?”
The answer to that question is yes.
And that by looking at these documents and looking at these strong metal signals in the documents, we can kind of fingerprint the types used to print them.
This would also allow us to try to reconstruct the specific metal alloys that were used to cast these types.
We can learn a lot about the resources the early printers had access to and potentially what informed their decisions in making these different types.
These are very specific alloys that would have to be used to make these prints, as if your alloy is too hard, you could pierce through the paper, but if it’s too soft, it could become degraded with very few prints.
The reason that determining the alloy is so important, not only can we fill in the gaps in our knowledge of printing presses, particularly when it comes to the Gutenberg press, where we know nothing about the alloys used to print it.
But fingerprinting these metal alloys used to print these documents could also give us a basis for comparison between the two printing domains.
If the types are very similar, for example, it could help back some theories that suggest that there was a crossover.
But if the types are very different, it would back the theory that these two inventions were created completely independent from each other.
But in order to jump to any conclusion like that, we need to do heavy analysis of all of the data that we have collected.
And particularly, we need to come up with a way to eliminate potential sources of this metal that are not from the types.
In order to do that, we have set up hand-printing experiments.
Now, these are a collaboration with several of the other people on our team that are experts in things like casting types and making paper and printing ink.
And actually, through these scholars, we were able to hand-cast the types shown here on the left, which are very specific and controlled metal alloys, in this case of tin, antimony, and lead.
And then another scholar handmade the paper according to European papermaking processes.
And a third scholar handmade the ink.
And he was very careful not to introduce any direct metal content into that ink.
And the same was done for the paper; no direct metal ingredients in the paper.
So all together, we generated these pressed documents’ printing using these types and these ink.
And by analyzing this data, we hoped to determine if the metal signal we see changes.
Or first of all, if the metal signal is present at all, because that would indicate really there’s only one source it could have come from.
But also if the metal signal changes depending on if the ink is exposed to the types or not.
That is, we can actually learn if metal is coming from the types, if it is just being brushed off, or if it’s actually being leached out from the ink.
All of these sort of experimental questions will help us determine whether or not it’s possible to fingerprint these types.
And sort of in conclusion, determine the answer to this question of, “Can X-rays trace the origins of printing?”
Now, in terms of the data analysis so far, that is ongoing, and it involves a lot of crossover with the other scholars on our team.
We are sort of checking all of our boxes and making sure the data that we see and the conclusions that we’re drawing are true before revealing the information.
Although I can show you some other maps to kind of give you an idea of what these documents are looking like.
For example, here is a 15th-century Korean document.
And in one channel, we see the clear copper signal, and then the other, this is a manganese channel, in which we don’t see anything.
And I put this print up to display kind of what the document looks like when we don’t see a signal.
Here’s an interesting one.
This is actually a printing of The Canterbury Tales, specifically “The Knight’s Tale,” done in 1476 in Europe.
On the left here is a map of zinc, whereas on the right is a map of copper, indicating too potentially some different type of metal alloy, if the metal is coming from the type.
Once again, here’s a 15th-century Korean document.
And what’s interesting about this is there’s actually significant water damage done to the document.
And that’s interesting because we can actually see how the metal interacts with water, how it spreads through the paper, and get a different chemical sense of the type of metal that’s in there.
And finally, this is an interesting one, Speculum historiale printed in 1474.
This is a document, like many of the documents, which contains what we call rubricking, or sort of hand embellishments done after printing.
So the signals that are appearing in red are actually done with red ink, embellished on the printed paper afterwards.
And we can see how the ink composition in the printed ink versus the embellished ink differs between the documents.
But overall, I would really like to emphasize that the work done in this project could not be done without all of our collaborators coming together.
And in fact, even though we as physicists were handed these historical documents and we were able to scan them, we could not interpret the meaning behind anything we saw without consulting the scholars.
And it’s actually consultation from the scholars that allows us to inform our next steps.
The only reason we decided to try to make our own hand printing is because we worked together with scholars to determine that is probably the best next step.
And similarly, moving forward as we analyze the data, we will develop more and more new experiments to try to test new hypotheses.
And it’s this interplay between the science side of things and the cultural historical humanities side of things that allows us to conduct this research.
I hope that overall, our project will really illuminate the importance of doing this type of interdisciplinary work and encourage other physicists and hard scientists to consider applying their very heavily, sorry, consider other physicists and other hard scientists to consider applying their techniques to fields that you wouldn’t typically associate with those sciences.
It is also my hope that our research here will shed more light and, I guess, awareness on the history of Korean print.
Printing overall was a hugely influential invention.
And both printing centers in Europe and in East Asia developed and arrived at this hugely important invention.
In 2027, we hope to produce scientific results that will give us some information that we didn’t know before about these presses.
And working together with all of the scholars on this collaboration, we’ll be holding several exhibitions around the world, hopefully, but mainly in Cheongju, Korea that put on display these documents and reveal to the public what we have learned.
That year, 2027, will actually be the 600th anniversary of the discovery of the Jikji.
So it’s all a very big, full-circle moment in the world of printing.
Our research is ongoing, but you can track the progress of it on this link here to the ” Jikji to Gutenberg” collaboration.
Thank you so much for your time, and I hope you learned a lot about printing today.
[audience applauding]
Search University Place Episodes
Related Stories from PBS Wisconsin's Blog

Donate to sign up. Activate and sign in to Passport. It's that easy to help PBS Wisconsin serve your community through media that educates, inspires, and entertains.
Make your membership gift today
Only for new users: Activate Passport using your code or email address
Already a member?
Look up my account
Need some help? Go to FAQ or visit PBS Passport Help
Need help accessing PBS Wisconsin anywhere?
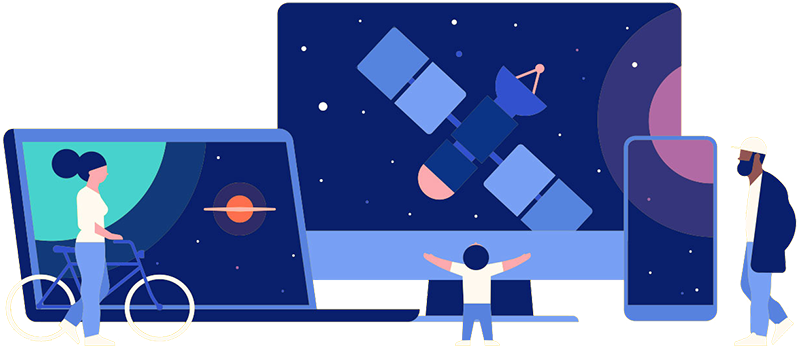
Online Access | Platform & Device Access | Cable or Satellite Access | Over-The-Air Access
Visit Access Guide
Need help accessing PBS Wisconsin anywhere?
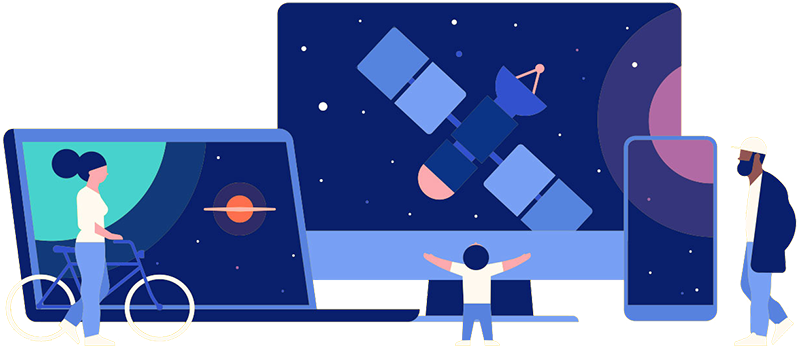
Visit Our
Live TV Access Guide
Online AccessPlatform & Device Access
Cable or Satellite Access
Over-The-Air Access
Visit Access Guide
Follow Us