[xylophone music under the video of a globe spinning with the title, The Wonders of Physics with Professor Clint Sprott, and the subtitle, 2017 Physics of Space Travel]
[narrator, Peter Weix, dressed as a Klingon]
Star Date 02.19. 2017. Captain Sprott has heard about a planet orbiting the temperate zone around Proxima Centauri which is the nearest star to our solar system, and he has come up with this crazy idea about mounting an expedition and seeking out new life and new civilizations and boldly going where no one has gone before. And so here for the 34th year that indefatigable schemer, that intrepid space explorer, the wonder of physics Professor Clint Sprott.
[upbeat music]
[Clint Sprott, Professor Emeritus, Department of Physics, University of Wisconsin-Madison, dressed as a Star Fleet Officer from Star Trek – The Next Generation appearing from behind a curtain]
Welcome to The Wonders of Physics. Now when I was a student one of the things that inspired me to go into physics was when John Kennedy have that speech and he said, We choose to go to the moon in this decade and do the other things not because they are easy but because they are hard. Now that was over 50 years ago, and I think we need a new challenge. Going to Mars is too easy. I think we should go all the way to that new planet just discovered around our nearest star.
[Peter Weix, dressed as Klingon]
But Captain Sprott we don’t have any rockets powerful enough to get us there.
[Clint Sprott]
Nonsense, in fact I have our Chief Engineer Mike Randall working on that problem right now. And he’s here to give us his report. Lieutenant Zhulu beam him in.
[Female assistant, Dani Zhu, dressed in a red Star Trek – The Next Generation uniform]
Aye Captain.
[house goes dark]
[computerized beeping and red and green dotted lights appear]
[applause]
[bagpipe music]
[Mike Randall, Professor, Department of Physics, University of Wisconsin-Madison, dressed in a Star Trek – The Next Generation top and a kilt]
Aye, Captain, call me Scotty. You cannot change the laws of physics. Long may your lum reek. You’re a wee scunner. My hovercraft’s full of eels.
[Clint Sprott]
Scotty? Mr. Randall, I didn’t know you were Scottish.
[Mike Randall]
Actually, I’m not I lost a bet with my wife.
[laughter]
[woman shouting from the audience]
[Mike Randall]
Thanks, honey.
Anyway, let’s talk about what it will take to get to Proxima Centauri.
Space travel is governed by Newton’s three laws of motion. Newton’s first law says that an object at rest stays at rest and an object in motion stays in motion. Or put in simple terms stuff –
[picks up a basketball]
– or matter as scientists like to call it – stuff wants to keep doing what it’s already doing.
If something is sitting still, it wants to keep sitting still. If something is moving it wants to move in a perfectly straight line at exactly the same speed forever.
Or until some outside of it pushes or pulls on it.
Now Newton’s first law is good news for space travel. That’s because space is mostly space. There’s really not much for our spacecraft to run into to slow it down. So, once we get it up to speed it will pretty much keep going on its own.
Now for Newton’s second law I’m going to need help from a cadet in the audience.
[pointing at the audience]
How about you sir? Yup yup, you right there, yes, yes yes yes.
[young boy comes down from the audience to the front of the lecture hall]
[applause]
[Mike Randall]
Alright and what is your name?
[Young boy]
Tristen.
[Mike Randall]
Tristen here you go.
[Hands Tristen the basketball]
[Tristen]
Alright.
[Mike Randall]
Just stand right here.
Okay Tristen [claps for the ball]
[Tristen throws ball to Professor Randall]
[Mike Randall]
Oh, that was a great pass.
Hey Tristen – [hands ball back to Tristen]
– how did you get the ball to me?
[Tristen]
I threw it to you.
[Mike Randall]
Yeah, you threw it to me. What – what did you do to [makes pushing motion] the ball when you threw it to me?
[Tristen]
I pushed it.
[Mike Randall]
Yeah, you pushed it. Now scientists would call a push or a pull a force. So, Tristen you applied a force to that basketball. Now the ball is made out of stuff.
Everyone what do scientists call stuff?
[Audience]
Matter.
[Mike Randall]
Matter, matter. The amount of stuff in that ball, the amount of matter in the ball is called its mass. Okay? So, you applied a force to a mass. You with me?
[Tristen]
Yeah.
[Mike Randall]
When you did that, when you pushed on the basketball the ball which hadn’t been moving suddenly did what?
[Tristen]
It moved.
[Mike Randall]
It moved but it moved in a very special way. Scientists and astronauts have a word for this. Long word starts with A, shout it out.
[Audience members]
Acceleration.
[Mike Randall]
Acceleration, very good.
Acceleration means a change in speed or direction. You made the ball speed up, so you made it accelerate.
[Tristan]
Yup.
[Mike Randall holding up two fingers]
Newton’s second law puts all three of those together. Force, mass, acceleration all go together. In fact, you may even know it as a math equation force equals mass times acceleration. The important thing to remember is that force, mass, and acceleration go together.
Okay, Tristen we’re going to do this again. [takes basketball from Tristen]
[Tristen]
Alright.
[Mike Randall]
We’re just going to do it with a different ball. [puts down basketball and picks up medicine ball]
Here you go. [hands medicine ball to Tristen]
[Tristen]
Whoa, okay. [tosses medicine ball back to Professor Randall]
[Mike Randall]
[grunts] Medicine ball. [laughs] A lot more mass.
So, [hands medicine ball back to Tristen] Tristen did you push on that ball the same as before?
[Tristen]
No.
[Mike Randall]
No, did you push less, or did you push more?
[Tristen]
I had to push – pushed much more.
[Mike Randall]
Pushed much more. Thats because – and you knew to do that, I didn’t tell you to do that. You knew because you understand Newtons second law. You knew that with more mass you had to apply a bigger force to get the ball accelerating fast enough to make it over to me.
Excellent job sir.
Let’s give Tristen a huge round of applause.
[applause]
Thank you.
[sets medicine ball down]
Now how does Newton’s second law fit into space travel? Well, Proxima Centauri’s still a long way off so we want our spacecraft to accelerate to the highest speed possible.
Newton’s second law says there’s two ways to do this. We could either – we could apply a really large force, and we can also reduce the mass of our spacecraft, make it lighter.
For example, world famous physicist Stephen Hawking last year along with his fellow scientists and investors announced a really exciting new project called Breakthrough Starshot where they’re planning on building miniature robotic spacecraft. And they’re going to send them to nearby stars like Proxima Centauri. These space – these nanobots [picks up ping-pong ball] have about the same as this ping-pong ball. Now it turns out I have an identical ping pong ball in this tube. Let’s see what happens when I apply a really large force to that small mass.
So, what I’m going to do is I’m going to pump all the air out of this tube.
[turns valve on tube to let the air out – at the end of the long tube are two aluminum cans]
In a minute I’m going to pop the tape on this end. The air’s going to come rushing back in. It’s going to apply a force of about 25 pounds to that ping-pong ball, we’ll see what happens to it.
Now this is loud folks. You’re going to want to cover your ears. I’m going to put my hearing protection on. [puts on earmuffs]
And in five, four, three, two, one.
[removes tape from non-can end of tube]
[loud bang]
[ping-pong ball travels through the tube rapidly and goes through both aluminum cans and ends up on the next table over from the table with the tube]
[organ chord plays Ta-Da]
[Mike Randall]
He-he. [closes valve, puts down earmuffs, and walks to can end of tube]
Did we lose it?
Oh, it’s over here.
[holds up aluminum can now with a large ping-pong shaped hole through it]
Went right through that one. And it’s embedded in this one.
Anyway, that went really fast right? That ping pong ball experienced almost 2000 Gs of acceleration. And ended up at a top speed of almost the speed of sound. Just about 760 miles per hour.
Now the nanobots I was talking about earlier; they’re not going to be powered by air pressure. Once they’re in outer space they’re going to unfurl giant reflective sails about the size of this portion of the wall. Huge ground-based lasers will shine on those sails and accelerate the nanobots up to 20% the speed of light. That’s 134 million miles per hour. And they’ll do it in about 10 minutes.
Now once they’re up to speed it will still take about 20 years for them to get to Proxima Centauri. Once they’re there they’ll start taking pictures and sensor data and they’ll radio that data back to Earth. Now radio waves travel at the speed of light, but that data will still take another four years to get back here to Earth.
Now I’ve saved the best of Newton’s laws for last. Newton’s third Law says that for every action [points left finger to the right] there is an equal and opposite – [points right finger to the left]
[audience members]
Reaction.
[Mike Randall]
– reaction. Or put in simple terms, if you push against [makes pushing motion] something it pushes back [makes pushing motion in opposite direction].
Something I didn’t tell you at the beginning of the show, I – I used to be a rocket scientist. I’m not kidding about that. I used to work on the Space Shuttle main engines and other rocket engines. So, I know that Newton’s Third Law is often referred to as the Law of Rockets, here’s why.
[picks up leaf blower]
In a rocket engine you got a lot of gas going this way –
[moves arm away from blowing end of blower indicating direction of flow]
– that’s the action. And the rocket goes this way –
[moves arm away from engine end of leaf blower indicating opposite direction of flow]
– that’s the reaction. Now I can also tell you as a rocket scientist that this is a leaf blower.
[laughter]
Oh, but the idea is still the same.
[turns on leaf blower as he stands on circular platform]
[leaf blower blowing air causing Mr. Randall to spin on platform]
[organ music playing]
[Mike Randall sickly moaning – eventually stepping from circular platform and walking dizzily]
[laughter]
[Mike Randall leaning over pretending to vomit]
Blargh!
[laughter]
Oh, this happens every time.
[Mike Randall picks up leaf blower, turns it on and aims it at children in front row of auditorium]
[leaf blower blowing]
[children squeal]
[Mike Randall turns off leaf blower]
I’m sorry.
[laughter]
Anyway. Now that we understand how Newton’s Third Law works, I have a rocket here with me, you want to see it?
[audience]
Yeah!
[Mike Randall rolling a rocket out on a cart]
This rocket is powered by ethanol.
[lifts up 5-gallon water cooler bottle filled with a small amount of ethanol]
There’s a little bit of ethanol in here.
[shakes and swirls ethanol in the water cooler bottle]
I’m going to shake it up a little bit.
[sets bottle down on end of 45-degree ramp that is on the top of the cart; the opening of the bottle is towards the bottom of the ramp]
Okay, set it up.
[Peter Weix, dressed as Klingon]
Are you sure this is safe?
[Mike Randall]
It’s safe for me.
[Narrator dressed as Klingon]
I’m out of here, you’re on your own. [exits behind curtain]
[Mike Randall]
We chased off the Klingon. [laughs]
Okay, again this is going to be loud. You’re going to want to cover your ears.
[places earmuffs on]
All right, give me a big countdown.
[lights butane lighter and moves it towards end of the bottle]
[Mike Randall and audience]
And in five, four, three, two, one.
[Mike Randall lights ethanol on the end of the bottle, flames shoot out of the end of the bottle and sends the bottle off the ramp and to the far end of the room]
[water bottle bounces off the floor]
[Mike Randall lifting his arms in exaltation]
Yeah!!
[organ chords play Ta Da]
[applause]
[Mike Randall, moving the cart towards the curtain while the Klingon narrator returns]
[to Klingon narrator]
It’s safe.
[Klingon narrator]
Oh, thanks for warming my seat up for me.
[Mike Randall]
You’re welcome.
[audience full of excited chatter]
[Mike Randall]
Pretty impressive, all that hot gas went this way [indicates to his right], and the rocket went that way. [indicates to his left]
[alarm blares]
[sound of chirping Tribbles]
[Mike Randall]
Oh no, we’ve got Tribbles in the replicators again.
[young audience member]
Nooo!
[Mike Randall]
Hey, I bet if I – I bet if I beamed the Tribbles over to Mr. Weix’s house, if I did that then they’d be no Tribble at all.
[audience groans at the pun]
Okay see you later. [waves and exits stage right]
[organ plays Ta Da!]
[applause]
[Klingon narrator, Peter Weix]
Well, he had some very moving ideas. But, you know, Captain Sprott in space it’s very, very cold. How are we going to stay warm?
[Clint Sprott]
Well, heat is the easiest form of energy to generate. There are many ways we can do that. And Dr. I. Kra’Nee will explain. Lieutenant Zhulu, beam him in.
[Dani Zhu, pretending to work the teleporter]
Aye, Captain.
[computerized beeping]
[house goes dark]
[red and green dotted lights light up]
[Terry Craney, Professor, Department of Physics, University of Wisconsin-Madison as Dr. I. KraNee dressed in a blue Star Trek – The Next Generation uniform]
Thank you, Captain Sprott.
[applause]
So yes, let’s talk a little bit about heat and heat transfer and how we can transfer heat.
What I have here [indicates a large heating coil in front of a sliver parabola] is a heating coil. Uses electricity and is very similar to a coil that you have in your hair dryer at home. Except it’s just a little bit bigger. And this coil is going to produce a type of heat called infrared radiation. Now infrared radiation is very similar to visible light, the light that we see. It can be reflected; it can be focused.
And by the way do you know what happens to light when it turns bad?
It has to go into a prism.
[crickets chirping]
[laughter]
All right, we have infrared radiation being produced here [indicates heating coil], infrared light being produced here. That light is at the focal point of a parabolic mirror.
That infrared radiation is going to go into the mirror and be reflected across the room here –
[walks across the back of the lecture hall to another parabolic mirror on the far end of the room that has an unlit match at its focal point]
– the 25 feet or so over to another parabolic mirror over here. And at the focal point of this parabolic mirror, I have a match.
Now this may take a minute or two. But keep one eye on that match. We’re going to use infrared radiation to heat the inside of our spaceship. And it’s going to be the main way that we heat the inside of our spaceship. So again, watch that –
[match in the far parabolic mirror lights and begins to burn]
– while I set up –
[organ plays Ta Da!]
– there it goes.
[blows out match]
So, let’s talk about another way of producing heat and moving heat around and that is through the use of gases.
So, I’m going to light a candle.
[uses butane lighter to light a candle that sits in a holder on a table]
This is a normal candle here that you might have at home.
And I don’t know if you’ve ever thought about how a candle works, it’s really quite interesting, really quite simple.
We burn the – the wax and the heat [moves his hands upwards from the top of the candle] from the wax burning has a tendency to rise. The molecules vibrate [closes his hands and shakes them] faster, they take up [uses both hands to indicate a large circle] bigger spaces. And therefore, we say they are less dense. And what do we know about less dense materials? They have a tendency to –
[moves his hand in an upwards motion]
[audience]
Rise.
[Terry Craney]
-rise.
So, the air above the candle [moves his hand upwards] has a tendency to rise.
[moves hands in a sweeping motion towards the bottom of the candle indicating cold air]
Then cold air from the sides and the bottom come in and fuel the flame with oxygen molecules.
By the way don’t ever trust an atom or a molecule.
They make up everything.
[rimshot plays]
[audience groans]
You want another one?
[Audience]
Sure! Yeah!
[Terry Craney]
Two atoms are talking, and one says, “I lost an electron.” The other one says, “Are you sure?” And the first one says, “Yes, I’m positive.”
[rimshot plays]
[laughter and applause]
Anyway, back to my candle. This system of the rising air and the cool air coming in from the bottom will not work in our spaceship. And it won’t work because we have no gravity, and we have no density difference. So, the hot air won’t rise [moves hand upwards] and the cold air [makes sweeping movement to bottom of candle] won’t come in to take its place. And I’ll talk more about that in just a moment.
So, what I’m going to do now is take a glass tube. [holds up a glass tube]
Just a regular glass tube and it fits right over the candle. And I think this is pretty straight forward.
[places tube over top of candle on candleholder]
But watch what happens when I put it over there. It’ll take a second or two, but the candle does what?
[candle goes out]
Slowly goes out, okay.
And I think that’s pretty obvious. We don’t have the oxygen air [makes sweeping motions towards the bottom of the candle] the cold oxygen air coming in from the bottom to feed the candle.
[removes tube from candle]
So, one of the things about physics and about science is that any experiment is repeatable.
[relights candle with butane lighter]
So, I’m going to do it again. But this time I’m going to throw a little curve at you. I have a piece of metal here [holds up a T shaped piece of copper metal] a T type piece of metal. And I’m going to simply put that right down the middle of my tube.
[places T-shaped metal into the tube]
Now I’m going to put the tube over the top –
[places tube with metal over the candle in candleholder]
– like so and you’ll notice what happens.
[candle flickers but stays lit and doesnt go out]
It flickers, it’s not as strong a flame, but it stays lit.
Now why is that?
Well, what we have here is we have two different paths [moving his hands up and down alternately] for the air to flow. The cold air can come down one side [moves one hand down] with oxygen and feed the flame and the hot air [moves other hand up] can go up the other side to exit. And again, we have a density difference.
Now, how can we – how – how do we know which side is going to be which?
[uses butane lighter to light a smoke wand]
Well, it’s purely by random here but I’ll show you that if I use my smoke wand here –
[moves smoke wand to the top of the tube]
– one side –
[moves smoke wand to the one side of the tube and smoke rises]
the smoke will rise and the other side –
[moves smoke wand to opposite side and smoke goes into tube]
– it goes down. In the last show it was just the opposite.
Okay, to show that, I said earlier that this – this process is called convection currents. Convection currents cannot be setup in our spacecraft because there’s no density. So, how are we going to move air around [makes sweeping motions with his hands] in our spacecraft to heat the astronauts and keep our equipment warm?
Well, we’re actually going to have to use big blowers and fans and because of that it’s going to be very noisy inside of our spacecraft. Now to show that an experiment or a demonstration is repeatable –
[removes T-shaped metal from the tube]
– I’ll simply pull this out and what should happen?
[audience]
It goes out.
[candle slowly goes out]
[organ plays Ta Da!]
[Terry Craney]
It goes out.
[applause]
So, over here I have a balloon. It’s obviously less dense because it has a tendency to rise. Okay. What do you think the material in that balloon is?
[young audience member]
Helium.
[Terry Craney]
Could be helium, could be helium in there, yes absolutely. Because helium in most of the toy balloons that we have is – is helium inside of it.
What other material could be in there?
[another young audience member]
Plain air.
[Terry Craney]
No, if it’s plain air, would it rise? Because its not – its – it has to be less dense than air. Okay.
[older audience member]
Hydrogen.
[Terry Craney]
Could be hydrogen. Okay, hydrogen is a very explosive material. Helium is not.
So, how could we tell the difference between that balloon and whether it has hydrogen or helium in it?
[audience member]
Light it on fire.
[Terry Craney]
Light – now there’s an idea, Light it on fire, he says.
Heaven forbid, what could go wrong with that?
[laughter]
Maybe we should ask the designers of the Hindenburg blimp what could go wrong. Oh, what the heck, let’s do it.
So, I’m going to take a match here.
[holds up match]
[places match on the end of a long stick]
I’m going to put it on here.
[strikes match and holds stick with match up to the ballon]
[young audience member]
No.
[another young audience member]
Dont do it!
[balloon pops with no visible flame]
[Terry Craney]
What do you think?
[audience member]
Helium.
[Terry Craney]
Helium, I popped the balloon. It’s just like popping it with a pin, but no big explosion, right? So, obviously I had helium in there, okay.
Now over here on the other hand –
[indicates another balloon on opposite end of the stage]
[laughter]
[Klingon narrator, Peter Weix appears with a blast shield on over his head]
[audience member]
We need a volunteer.
[Terry Craney, indicating Mr. Weix]
Chicken!
Over here I have another balloon. Well let’s see what’s in this one all right.
[strikes a match and holds lighted match to the balloon]
You may want to cover your ears on this one.
[balloon explodes in large red flame]
[organ plays Ta Da!]
[applause]
[Terry Craney]
Obviously, I had –
[young audience member]
Hydrogen!
[Terry Craney]
– hydrogen in there.
Now we are going to use hydrogen as the main fuel in our rockets to get outside of the Earth’s atmosphere and to get outside of the Earth’s gravitational field. For two reasons, number one it’s very light and not very dense. And secondly, it’s very energetic. It has lots of fuel in it.
So, you see Mr. Weix, we have nothing to worry about here. We can use infrared radiation to heat the inside of our spacecraft. We can move air around inside our spacecraft. And we can use hydrogen to propel our rockets. So, we will have a very successful trip.
Thank you.
[organ plays Ta Da!]
[applause]
[Klingon narrator, Peter Weix]
Well, I’m starting to warm up to the idea of space travel now.
So, Captain Sprott how so sure are you that there is a planet out there?
[Clint Sprott]
Well, I’m glad you asked.
There are actually four ways we can detect exoplanets. One way is to try to image them directly. But you know our present telescopes are not nearly powerful enough to make that practical. Another way is to use an affect predicted by Albert Einstein almost 100 years ago. It’s called gravitational lensing.
Imagine if this light bulb [indicates a light bulb on the end of a long holder] is a distant star. And you’re seeing it across outer space. And suppose another star –
[indicates a large lens with a hole in the center to the right of the light bulb]
– comes between you and that star. Now I don’t have a star and I don’t have a gravitational lens, but I have an ordinary lens. And the phenomenon is the same.
When this star comes between you and the star behind it you see the intensity of the light increase. And if you look carefully, you may see that the image of this appears as a ring. And that’s called Einstein’s ring. And he predicted that long before it was ever observed in space. And several planets have been discovered this way. Very distant planets, but it requires this special circumstance of one star in front of another. And so, it’s not all that practical.
Another way one can do, imagine this is a planet [indicates a ball on the end of a stick] orbiting the star. As it comes around between you and the star [moves ball around the light bulb] you can see the intensity of the light decreases. And so, by looking at – at stars and monitoring the intensity of the starlight. If every now and then you see it dip, decrease, and very periodically, you can infer that there must be a planet in orbit around it.
Now some of you over there didn’t see that. And that’s because you’re not in the plane [indicates with his hand a plane in front of the light bulb] of the orbit. And that’s a problem detecting exoplanets as well. We can detect some that way, but certainly not all. And there’s actually a satellite devoted to finding planets by this very method. And hundreds of them have now been discovered using that process.
[turns off light bulb]
But that’s not the way the planet was discovered that’s around Proxima Centauri. It was discovered using the Doppler effect.
Have you heard of that?
Oh yeah, you stand beside the road and a car goes by sounding its horn.
Sounds something like – Neeeerrroooow! When it’s coming towards you it has a high pitch. When it’s going away from you it has a low pitch. Now I can illustrate that with something here that makes a sound.
[holds up a whistle in a whiffle ball on the end of a string]
It’s a little whistle and when it moves toward you the pitch of the sound goes up. When it moves away from you it goes down. Now you probably couldn’t here that very well so let me make it go a little faster.
[swings whiffle ball around his head]
[oscillating whistling]
Can you hear the pitch go up and down and up and down?
But a curious thing it sounds perfectly normal to me. Why would that be?
[audience member]
Youre standing still.
[Clint Sprott]
That’s right it’s not moving toward me or away from me.
[stops swinging whiffle ball and turns off whistle]
And you know the police use this to find out how fast your car is traveling. Not with sound, but with radar waves.
Now of course planets don’t make sounds and neither do stars and even if they did we couldn’t hear it across the vacuum of outer space. But stars do emit light, and light has particular wavelengths associated with it. And by looking at the shift in those wavelengths we can infer that the star is moving back and forth.
How would that tell you there’s a planet? Well, if there’s a massive planet orbiting a star [moves his hand in a wide circular motion] the star actually moves back and forth as the planet orbits. And that causes the starlight to shift in frequency ever so slightly. In the case of Proxima Centauri every 11 days the star moves toward you and then away from you at a very slow speed of about two meters per second. That’s about walking speed in fact. So, I can be quite confident that there is a planet there for us to visit Mr. Weix.
[Klingon narrator, Peter Weix]
Well, Captain Sprott, maybe that would work. But, you know, how do you plan on building a propulsion system for such a long journey into space?
[Clint Sprott]
Well, we could use electric propulsion. In fact, Lieutenant Seltzman has been working on that. Lieutenant Zhulu beam him in in.
[Dani Zhu, pretending to work the teleporter]
Aye, Captain.
[house goes dark]
[small round red and green lights light up]
[computerized beeping]
[Teaching assistant, Andrew Seltzman appears in a blue Star Trek uniform dressed as a Vulcan and giving the Vulcan V-shaped greeting]
[laughter]
[applause]
[Andrew Seltzman]
Gas captain. We call it plasma, but it is merely ionized gas. To understand how to build an electric propulsion system we’ll first need to understand electrostatic repulsion. We can demonstrate such an affect with this device here called a Van de Graaff generator.
[indicates the Van de Graaff generator, a large silver sphere on top of a silver pedestal which is itself on a blue box]
In this Van de Graaff generator charge is moved from the blue box at the bottom up [moving his hands from the box to the top of the sphere] onto this sphere by means of putting it on a rotating belt. Let’s see what happens if we put a Tribble on top.
[places stuffed animal Tribble on top of sphere and turns on machine]
[Tribble squeaks and falls off]
Oh, it’s fur’s standing up. Doesn’t seem to like that at all.
[turns off machine and holds up metal wand and touches Van de Graaff generator which releases static electricity]
Now maybe we’ll need somebody who can stay still. We’ll need a volunteer from the audience.
[wide shot of audience with multiple children with their hands raised]
Let’s have you. [points to a longhaired young girl near the front row of auditorium]
Step right up here on to this plastic stool. And place your right hand on top of this dome.
[girl steps up onto a stool and places her right hand on top of the Van de Graaff sphere]
[Andrew turns on machine]
Now as you may know like charges repel. So, this Van de Graaff generator is putting charge onto your body. That charge is going onto the strands of your hair. Now if you’ll shake your head a little bit.
[girl shakes her head and hair]
Well, you see at the top some of your hair is staying on end. The charges on the strands of your hair are repelling from one another. This causes – this repulsion causes your hair to stand on end. The demonstration of electrostatic forces at work.
[turns off machine]
[young girl returns to the audience]
Thank you for your assistance.
[organ plays Ta Da!]
[applause]
Now let’s see how we can use these electrostatic forces to create motion. If we connect this Van de Graaff generator [connects motor to generator] to an electrostatic motor we can make things move by electrostatic repulsion itself.
[indicates a row of three empty aluminum foil coated 2 Liter soda bottles – the two on the ends are turned upside-down and one in the middle is right side up]
The Van de Graaff generator is connected to this aluminum foil coated soda bottle on the left. This soda bottle on the right is connected to the ground while the soda bottle in the center which has aluminum foil segments on it is free to spin.
Now we’re going to start spraying charge out of fine needle also connected to the Van de Graaff generator onto these aluminum foil segments and see what happens.
[turns on generator]
[the right side up center bottle starts rotating]
The charge placed on the bottle in the center is repelled from the bottle – from the bottle on the left towards the bottle on the right thus causing the electrostatic motor to spin solely from electrostatic forces.
[organ plays Ta Da!]
[Andrew Seltzman]
As you can see here.
[applause]
[Andrew turns off generator, releases static electricity and unhooks motor]
But now let’s see how we can use this to generate a propulsion affect with this electrostatic pinwheel.
[places the end of a pinwheel in the top of the generator- at the end of the pinwheel are two toy rockets – one red and one green]
This electrostatic pinwheel has two rockets on each end. At the back of each rocket there’s a very fine needle which concentrates the electric field generated by the high voltage from the Van de Graaff generator. This ionizes the surrounding gas. Now as you might know like charges repel. So, this ionized gas will be pushed away from the back of the rocket. And as every force has an equal and opposite reaction, this blowing of the ionized gas [moves his hand out from the back of the toy rocket] out the back of the rocket will cause the rockets to move forward.
[turns on generator]
Let’s see what happens.
[the pinwheel starts spinning]
The pinwheel begins to spin. A demonstration of propulsion using ionized gas.
[organ plays Ta Da!]
[applause]
[Andrew Seltzman]
But how will we use it to actually propel a spacecraft? Well, we’ll need to use a state of matter called plasma. Now plasma is a very high temperature ionized gas. If you took an ordinary gas such as air and continued to add energy to it, it would become hotter and hotter. Eventually it would have so much energy that the electrons would be free to move from the atoms. At this point the gas would become ionized. We can gen – we can create a plasma by adding energy by – with an electric arc, such as in this Jacob’s ladder here.
[indicates Jacobs Ladder, a large machine with a transformer on the bottom with two wire rods in a V-shape coming out of the transformer]
[turns on Jacobs Ladder and an electric plasma charge appears between the two rods, starting at the bottom and moving to the top where it breaks and then reforms on the bottom again]
In this Jacob’s ladder a transformer at the bottom places a high voltage between these two rods. This breaks down the air between them into a plasma. Now because the plasma is so hot it’s less dense than the surrounding air and thus begins to rise upward up the Jacob’s ladder until it breaks, and it reforms at the bottom.
Why, that’s so high voltage, if you were to touch it, it would not your Spock’s off.
[laughter]
[turns off Jacobs Ladder]
[Klingon narrator, Peter Weix]
Aw, you Vulcans are so naive. I think you meant to say it could knock your socks off.
[Andrew Seltzman]
That – that may be true but in this case it would be one and the same.
[pulls up bottom of his pants legs to reveal Star Trek Captain Spock socks]
[crickets chirping]
[laughter and applause]
But there are other ways to generate a plasma. For example, we can use radio waves.
[indicates a small microwave oven the door of which he opens]
We can demonstrate this by placing a fluorescent light bulb into a microwave oven.
[pulls a circular florescent light bulb from the microwave oven and holds it up]
Normally a fluorescent light bulb is plugged into a light socket. In that case the voltage from the light socket drives an electric current [moves his hands circularly around the light bulb] through a low-pressure gas in the tube causing it to light up. Much in the same way the Jacob’s ladder caused a plasma discharge. But a radio wave also has an electric field in it.
[places circular florescent light back into the microwave oven and shuts the microwave door and turns on the oven]
[the florescent light lights up]
In we place this fluorescent bulb into the microwave oven the electric field in the radio wave will excite the gas into a plasma causing the fluorescent light bulb to light up without being plugged in.
[turns microwave oven off and the florescent light goes out]
[organ plays Ta Da!]
[applause]
Now the thing about plasmas and why they’re so important for plasma propulsion is that a plasma rocket can be much more efficient than a chemical rocket. In a chemical rocket, you would combust a fuel and an oxidizer such as hydrogen and oxygen. That high temperature exhaust would then be expelled out the back of the spacecraft causing thrust. Now the thing is the hotter you can get that gas, the more efficient the rocket engine will become. And a plasma can be taken to much, much higher temperatures than chemical rocket engines thus a plasma thruster can be much more efficient than a chemical rocket engine.
In fact, we have such a plasma thruster right here.
[indicates a vacuum chamber on top of a table]
Within this vacuum chamber we’ll generate a plasma discharge by exciting a gas into a plasma with an electrical arc. Then using electrostatic repulsion we’ll blow out this plasma out of the plasma thruster into the vacuum chamber. Let’s have the lights off and see what this looks like.
[house goes dark, and Andrew turns on the vacuum chamber]
Here is the plasma is being expelled from the plasma thruster –
[glowing plasma brightens up one end of the vacuum tube and one can see it has created a plasma engine]
– this will generate a small but finite amount of force. Now this can add up to extremely high amounts of velocity over a long period of time. Such technically is already in use on a satellite on deep space missions. This is a type of propulsion system we’ll use on our journey to Proxima Centauri.
[house lights are turned back on, and Andrew turns off the vacuum tube]
[organ plays Ta Da!]
[applause]
My scientific expertise is needed on the bridge.
[makes Vulcan V greeting sign]
Live long and prosper.
[applause]
[alarms blaring]
[Klingon narrator, Peter Weix]
Alert! Alert! Alert! Alert! Alert!
Captain Sprott, Captain Sprott, what happens if we crash through an ion storm?
[Clint Sprott]
Relax Mr. Weix, there’s nothing to worry about. Lieutenant Norval can help us.
Lieutenant Zhulu beam him in.
[Dani Zhu, pretending to work the teleporter]
Aye, Captain.
[computerized beeping]
[house goes dark]
[red and green dots of light appear]
[Teaching assistant Ryan Norval appears wearing a yellow Star Trek – The Next Generation Uniform]
[Ryan Norval, walking toward center stage]
An ion storm you say Captain?
That’ll be no problem at all. We can just use some magnetic shielding.
[Clint Sprott]
Well, while you’re doing that perhaps you could explain to us how magnetic fields work.
[Ryan Norval]
Sure, so here I have a simple magnet. [holds up a circular magnet in his hand] And the first thing I need to know about a magnet is that every one of them is surrounded by magnetic fields. You guys might have seen the pictures of magnets with their North and South poles and the little field lines coming out and wrapping around from North to South.
Those magnetic fields are what’s interesting about magnets. They’re always invisible so we can’t readily see them right away, but I’ve got a little way of showing you how with this apparatus. Before I do that can anyone out there name for me the metals that are typically magnetic?
So, we’ll start right here in the front.
[young audience member]
Iron.
[Ryan Norval]
Iron, iron is one of three commonly known magnetic materials. You there?
[teenaged audience member]
Cobalt.
[Ryan Norval]
Cobalt’s another one. Anyone know the last one? How about you right there in the glasses, yep.
[Student]
Tin.
[Ryan Norval]
Tin is not really magnetic. I’ll take one more guess before I tell you. All the way up in the back there.
[teenaged audience member]
Steel.
[Ryan Norval]
Steel is usually magnetic because of the iron in it. Nickel is the last common one.
So, I’ve got something here called a ferrofluid. Now for those of you that are good with your Latin you might know what one of those three metals is in the ferrofluid. Anyone want to shout it out?
[audience member]
Iron.
[Ryan Norval]
Iron.
Iron’s in the ferrofluid. So, let’s see what happens then when we bring magnet next to ferrofluid.
[turns on light next to beaker full of ferrofluid and holds magnet to the bottom of the beaker]
[magnet attaches to the bottom of the beaker and sends up a hill shaped mass off iron shards]
[audience members]
Whoa!
[Ryan Norval]
So, I mentioned that the magnet’s got magnetic field lines. And each one of the spikes that you see on that hedgehog like structure there is a different magnetic field line. The iron in that fluid bubbles up and attaches itself to those field lines.
Now I want to show you guys that there’s two poles to the magnet so I can turn it around here.
[Ryan turns the magnet around and as he does the iron shards dissipate and return again when the other end of the magnet is arrived at]
The smooth section in the middle is where the field lines run parallel to the surface and then the South pole over here looks just like the North pole, pretty indistinguishable. And back around smooth section, magnet.
Now one of the interesting things about magnets is that they don’t actually do any work when they’re just standing there. I could leave this setup as it is, and nothing would change until forever basically. Magnets only do work when they change in time or space. So, the only time this magnet actually moves some material around is when I’m moving the magnet, it won’t do it on its own, something neat about magnetic fields.
So, let’s take a look over here at how we can make time changing or spatially changing magnetic fields do some work.
[holds up an aluminum ring and places it inside a magnet and nothing happens]
Here I have an aluminum ring. It’s usually not very magnetic at all. But we’ll do some interesting things here and I’ll make it jump.
[places aluminum ring on top of large electromagnet]
So, the aluminum ring is next to this electromagnet. The electromagnet takes electricity and generates a brief, powerful magnetic field. That magnetic field’s going to induce a current in this aluminum ring and it’s that current that will feel a magnetic force causing the ring to jump.
Let’s take a look.
Three. Two. One.
[turns on electromagnet and the aluminum ring jumps of the surface and Ryan catches it]
So, that wasn’t all too impressive by itself. But we can engineer this system to make it better. Better in this case means jumps higher.
[pulls a stuffed Tribble from a large iron rod]
These Tribbles getting in everyone’s way.
So, I have here just an iron rod.
[places large iron rod through the iron ring on top of the electromagnet]
Now what this iron rod’s going to do is it’s going to reshape my magnetic fields. So, I told you guys that while in the magnetic field the ring will feel a magnetic force when it’s got a current in it. The time-changing field makes a current. So, currently before the rod, we just had the field bubbling up here and the North pole came back down to the South pole. Now with the rod, the magnetic field’s going to extend the entire length of it. So, we’re going to accelerate the ring for longer.
Let’s see how high we can get it now.
Three. Two. One.
[turns on electromagnet and the aluminum ring flies all the way to near the ceiling]
[organ plays Ta Da!]
[Ryan catches ring, removes iron rod and places ring in an open circular Pyrex bowl]
[applause]
[grabs large container of liquid Nitrogen from on top of the table]
Now there’s one other thing that we can do to change the ring, I said that it’s the current in the ring that feels the force. So, if we put more current into that ring we can get more height out of it.
One way to change the current in it is to lower the resistance of the ring. And we can do that by dropping its temperature –
[pours liquid Nitrogen into bowl with aluminum ring in it]
– with this liquid nitrogen here.
[places cap back on liquid Nitrogen as bowl begins steaming]
Liquid nitrogen is very cold.
[picks up a new full container of liquid nitrogen takes the cap off an sets it down]
It’s minus 321 degrees. That’s 321 degrees below zero Fahrenheit. Now I want to show you guys that liquid nitrogen’s really quite cold.
[grabs a long plastic tube from the top of the table and holds it up]
So, here I have a plastic tube.
[wraps tube around his hand]
I can bend it [bends it], I can stretch it. [stretches it] You know, it’s very flexible.
Let’s dip it into liquid nitrogen.
[places end of the hollow tube into the liquid nitrogen and the non-submerged end releases gas]
So, the first thing you notice is that the liquid nitrogen spewed out this other end [tube falls out of liquid Nitrogen] and that’s because it was boiling [returns tube into liquid nitrogen] much like a teapot at home. Which actually, I should keep it in there. So, all that’s just nitrogen gas coming out. So, now, you know, it’s only like 10 seconds in there.
[takes out tube end that was in the liquid nitrogen and hits it with a mallet]
[tube end shatters into shards]
[organ plays Ta Da!]
[Ryan Norval]
Shatters like glass, it’s pretty darn hard. Can’t really bend or break it anymore. So, liquid nitrogen is cold quickly froze that.
[picks up container of liquid nitrogen]
Now, I like to get a little crazy sometimes. What if I told you guys I wanted to pour this liquid nitrogen over my hands?
[audience members]
Whoa!
[Ryan starts pouring liquid nitrogen over his hand and then switches hands and pours it over his other hand]
[pours liquid nitrogen on the floor of the lecture hall]
[Ryan Norval]
So, some of you up front can see this effect on the ground similarly. And those of you in the back might have seen this at home when you’re cooking. It’s a very similar affect if you take some water and put it onto a hot skillet. In this case my hand is acting like a hot skillet to liquid nitrogen which is relatively much, much colder. It’s called the Leiden frost effect. When you put that water into a hot skillet or the liquid nitrogen on my hand what happens? Well, it beads up and it pools off the edge. Why does it do that? Well because the little bit of nitrogen that first touched my hand evaporated into a gas. And that gas carried the rest of it right on over my hand and so, whatever I poured on me almost none of it make contact with me and it just fell on the ground.
Neat little trick.
[audience member]
What would happen if you put your hand in?
[Ryan Norval]
Oh, it would last until about my hand got cold and then it would get frostbite; it’d be horrible, absolutely horrible.
[grabs forceps and removes aluminum ring from liquid nitrogen and places it on top of the electromagnet]
So, let’s take a look at the cold ring now. So, I want you guys to make a bet with yourselves. Will the cold ring jump higher or less high than the iron tube?
[audience member]
Higher.
[Ryan Norval]
Three. Two. One.
[turns on electromagnet and the ring jumps a distance of half way between the first instance [with just the electromagnet] and the second instance [with the iron rod])
[Ryan catches the ring and places it back in the bowl]
A little less high, right?
[turns off electromagnet]
But now here’s the fun part.
Do you guys want to see what happens when I combine the two effects, the cold ring and the iron tube?
[young audience members]
Yes! Oh yeah!
[laughter]
[Ryan uses forceps to place the cold ring back on top of electromagnet and replaces the iron rod through the ring and on top of the electromagnet]
So then, I don’t know what’s funny about this.
I want you guys to make a bet with yourself now. Do we hit the ceiling?
Okay, give me a countdown, we’ll start at three.
[Ryan Norval and audience]
Three. Two. One.
[turns on electromagnet, and the ring almost touches the ceiling and lands one of the tables making loud pinging sounds]
[applause]
So, in all those experiments I never changed the strength of my electromagnet. I just changed how we use it.
Pretty neat.
But I want I really came up here to tell you about is how we can use these magnetic fields to protect ourselves from ion storms and other space weather, solar flares and what not. So, the magnetic field is doing work on the current in that ring. Now, ion storms are just charged particles. Moving charged particles are currents basically. Which means we should be able to use magnetic fields to affect them.
[lights of the hall are dimmed]
[indicates a cathode ray tube on the table that has a green colored electric current running through it]
Here I have a cathode ray tube, one that might have been found in an old TV like from the 60’s or 70’s. And here I have a standard bar magnet. [holds up bar magnet] Let’s take a look at what happens when we put the bar magnet near the cathode ray tube’s beam of electrons.
[wherever Ryan places the magnet along the tube the green current goes right to the magnet]
You guys can see the magnet bends the electron beam around. And depending on how I orient my magnet I can bend it in different ways. So, if we’re flying through an ion storm all we have to do is put a magnetic bubble around our spaceship and the particles will zip right around us without interacting with us or our ship, pretty neat trick.
[organ plays Ta Da!]
[house lights return to full]
[applause]
Now where that was a pulsed electromagnet this one’s an electromagnet that when I turn it on it’s going to flip it’s North and South poles back [moves his hands in a top to bottom movement alternately] and forth very fast. So, why might this be interesting? Well, other than ion storms and solar flares and charged particles in space, there’s the big rock and metal objects that float out in space. You guys know what they’re called?
[young audience members]
Asteroids!
[Ryan Norval]
Asteroids! Asteroids and comets. They’re mostly made of metal and magnetic fields like we say with the ring can affect metal, you know.
[indicates a steel rod with several large steel coils attached to the top]
So, here I have steel rod and it’s attached to these coils, these coils just serve to amplify the affect for the demonstration. And what we’re going to see here is that when we place the metal into the magnetic field –
[turns on electromagnet and then places metal rod with coils over the top of the electromagnet]
– which is changing back and forth rapidly it’s going to do something interesting. After about 20 seconds what happens?
[the metal rod starts to glow red]
The metal rods starting to glow.
And if I leave this in here, you know, for about another 30 seconds, I could liquefy it into slag, and it would just crack.
[removes coiled rod from the top of the electromagnet]
So, you guys can imagine on our star ship if there’s an asteroid coming at us if we had a sufficiently powerful power-supplied nuclear reactor or something and strong enough magnets, we could just pulse a magnetic field, liquefy an asteroid coming at our spaceship and be perfectly protected.
[organ plays Ta Da!]
[applause]
Now there’s one last interesting thing about magnetism I want to show you guys. And that’s the notion of a superconductor.
Superconductors are certain materials, typically rare Earth element that –
[tries to use forceps to remove liquid nitrogen cooled circular puck from the bowl, but fails and instead tries to lift it out with his hands]
Ah.
Get this out of here.
[removes puck and sets it down then picks it up with forceps]
Exhibit this very interesting property that when they get really cold, like liquid nitrogen temperatures –
[removes magnetic puck from forceps and places it on a large tray with a magnetic track whereupon it floats over and moves around the track, floating the entire time]
[audience members]
Whoa!
[Ryan Norval]
– they become superconducting, so they have no resistance which gives them very high electroconductivity properties and it’ll try to exclude magnetic fields. So, this track is made of just ordinary bar magnets. And when I put the puck there. You guys can see –
[moves forceps around the puck to show it is floating]
– no trickery here it’s truly floating. The puck has a magnetic field so strong that it keeps itself up above gravity. Now I’m going to put it a little lower.
[pushes the magnetic puck down and sends it zooming around the track]
You guys can see that this is a pretty frictionless track. And you can spin right around.
[applause]
So, how might this be useful on our journey? Well, if we have a really big ship like we want to go colonize Proxima Centauri, you know, and we want to get from one end to other in a ship we could make a little magnetic tube superconductor and –
[stops magnetic puck and zips it around the track in the other direction]
– zip from one end of our ship to the other while in space. The other thing is this is defying gravity here.
[stops puck]
We don’t know with the gravity’s going to be like on Proxima Centauri. So, why even bother dealing with it at all? When we land there and we start unloading colonial supplies and scientific equipment we could just lay out some magnetic track and use superconductors to levitate it all, not even being bothered by gravity.
[sends puck zipping around the track again]
Pretty neat trick.
[organ plays Ta Da!]
[applause]
So, you see Mr. Weix magnets will make our lives easier and protect us on the journey. You got nothing to worry about.
[Ryan Norvel exits stage right and Klingon narrator, Peter Weix moves to center stage]
[Peter Weix, dressed as Klingon]
Well, he had some very attractive ideas.
[groans and laughter]
But Captain Sprott, I’m still not sure that this is all going to work.
[Clint Sprott]
Well, we can erect a large sail and can use the pressure of the sunlight to push our ship along. Or maybe we could aim very powerful lasers at it and push it away from the Earth. Or we could build a nuclear reactor and carry it along with us. In fact, Lieutenant Ehlerding has been studying this. Lieutenant Zhulu beam her in.
[house goes dark]
[Dani Zhu]
Aye, Captain.
[computerized beeping]
[small red and green circular lights light up]
[Teaching assistant, Emily Ehlerding, dressing in a red original Star Trek uniform]
You know you’re exactly right. To get all the way out to Proxima Centauri we’re really going to have to plan it out.
[crickets chirping]
Oh, but really though, we have so many more power options available to us all using different types of radiation. Now when I say radiation, I’m not talking just about the heat radiation that we learned about earlier. I’m talking about light. Radiation’s just a fancy word for light. Now the visible light, the stuff that you can actually see makes up a teeny, teeny tiny part of the electromagnetic spectrum. But it’s an important part ’cause, you know, it kind of gets dark out there in space, right?
Did – have you guys ever noticed that when you see a star in sky it kind of looks like it twinkles? Yeah, let me show you why. So, I have my laser here, not a star but a laser and it’s hitting the wall up there.
[turns on green laser and points it at the wall]
You guys see that? Now when light comes to us from a star in outer space it’s has to go through, you know, space, there’s nothing there. Kind of like how this laser is going through –
[picks up a can of liquid smoke and starts shaking it]
– going through the air to hit the wall.
[house lights go to half and Emily sprays the liquid smoke into the path of the laser beam whereupon the green laser beam becomes visible]
If I spray some smoke, can you guys see the beam there? Yeah, so the laser is going all the way up and hitting the wall up there.
[Emily sets down the liquid smoke and house lights return to full]
So, it doesn’t really, you know, have to go through anything. But when light comes to us from outer space it has to go through our atmosphere which is a bunch of hot gasses and things like that. Kind of like the hot gasses –
[picks up a box of wooden matches, grabs a match, strikes it and lights a Bunsen burner that is elevated to just underneath the green laser beam]
-that are caused by my burner here if I can get it to light.
And my light twinkles.
[laser light on the wall starts twinkling]
[piano plays “Twinkle, Twinkle Little Star”]
And then I turn off my atmosphere –
[turns off Bunsen burner]
– and it goes back to being a boring dot.
[laser stops twinkling]
[organ plays Ta Da!]
[applause]
So, while I’ve got my laser here –
[grabs two cups and a beaker full of water]
– I’m going to show you one other cool thing here. So, I’ve got two cups here, right?
[holds up the two cups, one in each hand]
And I’m just going to take some ordinary water –
[holds up beaker of water]
and pour it into my cups –
[pours water into each of the cups]
– so, we got right there. Pour a little bit of water there.
Okay and now, I’m going to hold this in front of my laser.
[holds one of the cups in front of the laser]
[theme music from Jeopardy plays]
Now, we all know that lasers are powerful. You shouldn’t shine them in your little brother’s eye no matter how annoying he is. And we’ll let this here. Okay and I believe that’s good enough.
Alright, so this one –
[holds up cup of water that was not in front of the laser]
– didn’t go in the laser, right? So, I’m just going to pour the water back.
[pours water from the cup that didnt go in front of the laser back into the beaker]
And –
[picks up the cup that went in front of the laser and tries to pour it back into the beaker]
– then the one that went in the laser.
[cup that was in front of the laser is empty, Emily tries to still shake water out of it, but it is still empty]
How many of you guys knew that was going to happen? Alright?
Okay, well actually let me let you in on – on a little secret. So, when you’re doing a scientific experiment, you need a control for all your variables, right? You need to make sure everything’s the exact same between all your situations.
[holds up cup that went in front of the laser and shows that there was a sponge in the bottom of the cup]
Well, there was a sponge in one of them so.
[audience groans]
[Emily Ehlerding]
You didn’t see anything at all.
Sorry, guys.
[laughter]
[Emily Ehlerding]
Actually, its kind of is like magic, right? Do you guys know the difference between magicians and scientists? Magicians don’t tell you their secrets and scientists won’t shut up about them, right?
[lifts cover from a scientific instrument that has a glowing rod in it]
Now as a scientist myself I have another contraption that I would like to show you. I actually have a machine here that can change the color of a balloon using the power of light. Right? I know it’s really cool.
[lifts up a blue balloon and holds it]
You know you’re at your birthday party and your like, “I don’t really think I like blue balloons anymore. Maybe I want a yellow one,” or something like that., right? Well, that’s where my handy-dandy contraption will come in and change the color of your balloon. So, I have a light here, not a laser. But I do have a nice bright blue laser.
[turns on blue laser that shoots its light horizontally, put perpendicular to the scientific instrument with the glowing rod]
Can you guys see that?
Alright, so I got my color changing blue laser here.
[turns off blue laser]
So, I’m going to put my balloon into the box.
[places blue balloon into box with the glowing rod]
And we’re going to count down and we’re going to change the color. Sound good?
Alright! So, three, two, one.
[turns on blue laser and blue balloon in box pops, leaving a clear balloon in its place]
[organ plays Ta Da!]
Looky there. We changed the color of our balloon –
[opens top of box and lifts out and holds clear balloon]
– from blue to clear. I know, well.
[amazement and laughter]
So, since I’m a scientist, I’m going to let you in on the secret. I didn’t actually change the color of the balloon.
[young audience member]
What?!
[Emily Ehlerding, holding clear balloon]
I know, so it turns out there was a clear balloon inside of my colored balloon.
Yeah, so.
[young audience member]
Thats not really fun.
[Emily Ehlerding]
It’s really fun.
[laughter]
So, since that blue balloon is colored – right – my laser hits that balloon and the blue balloon absorbs a little bit of that energy, and it heats up a tiny little spot on the balloon there. When that balloon spot heats up it makes the balloon pop. But since my inside balloon was clear –
[holds up clear balloon]
– the laser just goes right through it. It doesn’t absorb any of the energy, so it doesn’t pop.
It’s actually a lot easier if we do it in reverse to see what’s going on.
[puts down clear balloon and holds up a new set of balloons with a blue balloon inside a clear balloon]
So, here I have a clear balloon on the outside of a colored balloon. So, we’ll do the same thing again and I’ll show you how it works.
[places balloons in the box and shuts lid]
I put that in there.
A little three, two, one.
[turns on blue laser and the inner blue balloon pops leaving the clear balloon]
[organ plays Ta Da!]
So, you can see it, yeah. So, lasers are really powerful. We could use a laser like that to get us out of our solar system. N.A.S.A. has actually said that if we could build powerful enough lasers and we could build sails that we could hit them with we could get to Mars in three days, right? So, we could use that to get out of the solar system.
Come to think of it, do you know how the solar system holds up its pants?
[young audience members]
No. Nope.
[Emily Ehlerding, lifting up her shirt to reveal a belt with an asteroid for a belt buckle]
With an asteroid belt.
[rimshot plays]
[groaning and laughter]
[Emily Ehlerding]
So, we could get out of our solar system using light and things like that but once we get out there, we’re going to have to keep going, right? And that’s where we’re going to turn to my favorite power, nuclear power.
Now, nuclear power just harnesses the energy of atoms as they fall apart or undergo fission.
I’ve actually got some radioactive sources here. And have taken entirely too many classes on how to handle these things safely but when we get out there in space, we’re going to want to protect ourselves. Well, that’s what we’re going to do here.
Now I’ve got three different sources here.
[motions towards a Geiger counter]
I have an alpha source –
[indicates an element on the far side of the Geiger counter]
a beta source –
[indicates an element in the middle of the Geiger counter]
and a gamma source.
[indicates the near end of the Geiger counter]
Now alpha particles are really big. Beta particles are kind of medium sized. And gamma particles are, you know, really light. So, if something has a lot of mass, is it going to go faster or slower than something that’s smaller?
[audience members]
Slower.
[Emily Ehlerding]
Slower, right we learned that earlier. So, these alpha particles, I’m going to show you with my vintage Geiger counter here, you’re going to hear this buzzing sound whenever I’m picking up the particles.
[holds Geiger counter end to side with alpha particles and Geiger counter buzzes]
You guys hear that? So, it turns out these alpha particles are so big I can stop them with a piece of paper.
Watch this, you can hear it.
[holds a post-It note between the Geiger counter end and the alpha particles, the Geiger counter buzzing slows. Then takes away the post-It note and the Geiger counter buzzes again]
[Emily Ehlerding]
Cut it right out, right?
Now, these beta particles since they’re a little bit smaller. Do you think they can go faster or slower than alpha particles?
[audience members]
Faster!
[Emily Ehlerding]
Faster, so what do you think the paper’s going to do?
[audience member]
Slow it down?
[Emily Ehlerding]
Well, let’s find out okay.
[places Geiger counter end on the beta particles and Geiger counter buzzes]
So, you hear the beta.
[places a post-it note in-between the Geiger counter end and the beta particles and the Geiger counter keeps buzzing]
Nothing, right? We don’t need that.
[throws post-it note to the ground]
Turns out we need – we need this –
[holds up a post-it sized piece of lead]
– big piece of lead to stop these beta particles so if you listen –
[holds up Geiger counter end to beta particles and Geiger counter buzzes]
– you go like this.
[places post-it shaped piece of lead between Geiger counter end and beta particles and the Geiger counter stops buzzing]
Cuts it right out, right?
[removes piece of lead and the Geiger counter starts buzzing again]
Now gamma particles are even smaller. So, what do you think the lead’s going to do?
[audience members]
Nothing!
[Emily Ehlerding]
Nothing. Let’s find out.
You hear that?
[holds up Geiger counter end to gamma particles and the Geiger counter buzzes]
[places post-it shaped piece of lead between Geiger counter end and gamma particles and the Geiger counter keeps buzzing]
[Emily Ehlerding]
You’re right it doesn’t do anything, so if you guys have ideas about how we’re going to shield ourselves from these gamma particles come talk to me after class ’cause we’re going to need to figure that out, right?
Well anyway, so nuclear reactors use really, really big atoms like uranium or plutonium, things like that. They’re so big they’re just, kind of, unstable. Turns out if we give them a neutron which is a teeny tiny particle usually found in the nucleus of an atom, these atoms will fall apart. When they fall apart, they give off more neutrons which are absorbed by more atoms which, you know, it just kind of keeps going –
[moves her arms in a circular motion]
– and going and going, right? It’s called a nuclear chain reaction.
Now I have a chain reactor over here –
[indicates a large rectangular chamber filled with small orange ping-pong balls]
– not a nuclear chain reactor, but a chain reaction. And I’m going to need somebody to help me set it off.
[holds up her hand indicating that she is looking for a volunteer from the audience]
[points to audience member]
In the red and white there, come on down.
[young volunteer runs down from the lecture hall]
[Emily Ehlerding]
Whats your name?
[young audience member]
Arie.
[Emily Ehlerding]
Arie?
[Arie]
Yeah.
[Emily Ehlerding]
Lets give him a round of applause, alright.
[applause]
[Emily Ehlerding]
Okay Arie, so we have our neutrons at the top of our reactor here.
You can step right here and face the audience.
We have our neutrons –
[indicates two orange ping-pong balls in a tube at the top of the container being held up by a small door]
– and we have all of our atoms down here.
[indicates the large number of ping-pong balls at the bottom of the container]
And you’re going to put the neutrons into our reactor and set it off, okay?
[hands Arie a handle that is attached to the end of a string that holds the top door closed]
Let me give you this and you’re going to pull it when we count down, okay?
Let’s go, three, two, one – give it a tug.
[Arie pulls on the string opening the door at the top of the container that releases the two orange ping-pong balls from the top of the container into the container proper. Arie lets go of string closing the door, whereupon the orange ping-pong balls all start bouncing around in the container]
[Emily Ehlerding]
Good job Arie! Thank you, you can have a seat.
[organ plays Ta Da!]
[Emily Ehlerding]
Alright, and it just keeps going, right?
It’s got so much power. You so see Mr. Weix, we have so many ways to get out there to outer space, don’t you think so?
[Klingon narrator, Peter Weix]
Yes, you have some very illuminating ideas.
[Emily Ehlerding]
I know I do but it’s time for me to make like uranium and split.
[Emily exits stage right and Peter Weix comes to center stage]
[laughter and applause]
[Peter Weix]
All right Captain Sprott, maybe this is going to work. But how long is all of this going to take?
[Clint Sprott]
Well, I was hoping that we would have this spaceship built by today but Steve Narf who sets out all our demonstrations was too busy playing with all the apparatus –
[Steve Narf appears behind Professor Sprott shooting large smoke puffs from a smoke machine]
– and didn’t get around to making it. But we do owe him a debt of gratitude for all the work he’s done.
[applause]
[Steve Narf bows]
[Clint Sprott]
But seriously I think we know enough physics to mount an expedition like this. But there’s a lot of technology that needs to be developed to carry humans on such a long voyage. And that may take another 100 years, who knows.
Furthermore, Proxima Centauri is very far away. And even if we could travel at 20% the speed of light it would take us 20 years to get there. But I’m quite confident that someday this will be done. And maybe one of you will be on that first voyage. And so, I’d better get back to making the starship that will eventually take us there.
And I would like to thank you all for coming.
[upbeat music]
[cast comes out and takes bows]
[credits]
Executive Producer and Host – Clint Sprott
Coordinator – Peter Weix
Lt. Zhulu – Dani Zhu
Chief Engineer – Mike Randall
Dr. I. KraNee – Terry Craney
Lt. Seltzman – Andrew Seltzman
Lt. Norval – Ryan Norval
Lt. Ehlerding – Emily Ehlerding
Technical Support – Tara Keenan, Steve Narf, Paul Nonn, Bill Zimmerman
Theme Music – Jim Latimer, Frank Ferriano
Cameras – Jaimie Doering, Brandon Duerst, Leigh Drogemuller, Steve Narf, Kelsey OHara
Audio – Steve Narf, Jaimie Doering
Editing – Leigh Drogemuller, Brandon Duerst
Copyright 2017 U.W. System Board of Regents, Department of Physics, University of Wisconsin-Madison.
Search University Place Episodes
Related Stories from PBS Wisconsin's Blog

Donate to sign up. Activate and sign in to Passport. It's that easy to help PBS Wisconsin serve your community through media that educates, inspires, and entertains.
Make your membership gift today
Only for new users: Activate Passport using your code or email address
Already a member?
Look up my account
Need some help? Go to FAQ or visit PBS Passport Help
Need help accessing PBS Wisconsin anywhere?
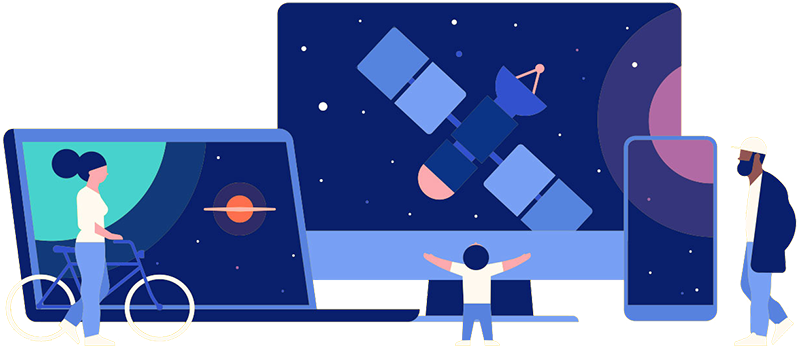
Online Access | Platform & Device Access | Cable or Satellite Access | Over-The-Air Access
Visit Access Guide
Need help accessing PBS Wisconsin anywhere?
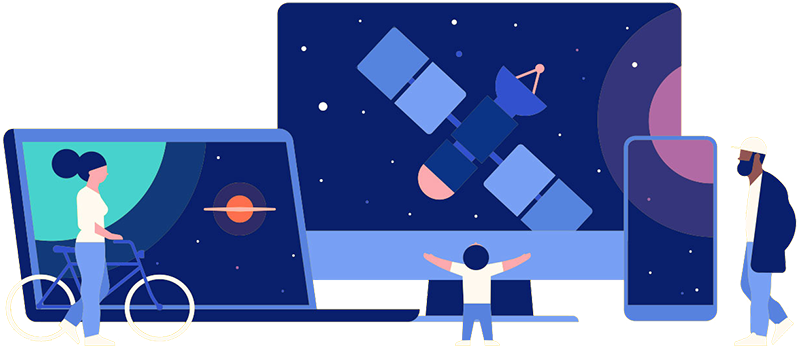
Visit Our
Live TV Access Guide
Online AccessPlatform & Device Access
Cable or Satellite Access
Over-The-Air Access
Visit Access Guide
Follow Us