[Tom Zinnen, Outreach Specialist, Biotechnology Center, University of Wisconsin-Madison]
Welcome, everyone, to Wednesday Nite @ the Lab. I’m Tom Zinnen. I work here at the U.W.-Madison Biotechnology Center. I also work for U.W.-Extension Cooperative Extension, and on behalf of those folks and our other co-organizers, Wisconsin Public Television, the Wisconsin Alumni Association, and the U.W.-Madison Science Alliance, thanks again for coming to Wednesday Nite @ the Lab. We do this every Wednesday night, 50 times a year.
Tonight, it’s my pleasure to introduce to you Peter Muir. He’s with the Department of Surgical Sciences in the School of Veterinary Medicine. He’s going to talk about man’s best friend, the genome-wide association analysis of complex traits of dogs.
Peter was born in Plymouth in the U.K. and went to high school there. He went to Bristol University for his original PhD and DVM. Then he went to the University of Sydney in Australia for a couple of years, came here to Madison, then went to the University of California-Davis, and then was at the Royal Veterinary College at the University of London. And then, in 1999, he came back here to Madison to be on the faculty, where he’s been ever since. I’m looking forward to tonight’s talk, “We All Have Knees.” Bees have knees; more importantly, dogs have knees, and they have ligaments that get stressed kind of like ours do. It’s one of the great things veterinarians get to do. They get to study more than us, and therefore, they can learn from lots and lots of different animals. Please join me in welcoming Professor Peter Muir to Wednesday Nite @ the Lab.
[applause]
[Peter Muir, Professor, University of Wisconsin-Madison School of Veterinary Medicine]
Okay. Thank you.
Alright. Thank you very much for that kind introduction. Can everybody hear me okay at the back of the lab or back of the room? Okay.
So, I’m just going to start this talk by giving you a brief outline of what I want to speak about. I’ve divided this topic into some sort of distinct sections. So, initially, I was going to just make a few comments about this topic of complex trait G.W.A.S. –
[slide titled – Outline – featuring the following bulleted list – Complex trait GWAS; Dog Breeds; ACL rupture in dogs and humans; ACL rupture GWAS in the dog; Not performed in humans to date; Future directions. The slide also features two photos to the right of the list – the first of a Golden Retriever and the second of a dogs leg bone]
– G.W.A.S. obviously standing for genome-wide association study. And to sort of extend that, I’m going to say something about dog breeds and how they were created over time. There’s this idea that dog breeding is the world’s greatest genetic experiment ever undertaken by humankind. And so, we’ll talk about that. I’m also going to talk about this condition of A.C.L. rupture, which is common in people and common in dogs as well. And then talk a little bit about our work and what we’ve done to study this topic –
[Peter Muir, on-camera]
– and why it might be advantageous to study this in dogs. And one important point is that today there are no published studies looking at A.C.L. rupture with G.W.A.S. in human patients. And so, just to conclude then, I’m going to say something about future – future directions and how we see our work unfolding over time.
So, I apologize if I’m explaining things that you already know, but I just want to start off with a couple of definitions. So, a genome is the entire set of D.N.A. for a person or an animal.
[slide titled – Definitions – featuring the following bulleted list – 1) Genome and the sub list – Entire set of D.N.A. for a person or animal; 99.9% of D.N.A. is identical between individuals of the same species. 2) Single nucleotide polymorphism (SNP) and the sub bullet – Nucleotide variation at a single locus. 3) Allele and the sub bullets – One or more forms of a gene or a genetic locus (group of genes); One copy on each chromosome. The slide also includes and illustration of a SNP between two DNA stands]
And almost all of this is identical between individuals in the same species. But, as you can see in this slide here, every once in a while, there is a base pair difference, which is referred to as a single nucleotide polymorphism. So, a difference or variation at a single locus. The other thing that I want to mention is on the define – what – what’s the definition of an allele. And so, this is one or more forms of a gene or a genetic locus, and there’s one copy on each chromosome.
[Peter Muir, on-camera]
One of the interesting things is that G.W.A.S. is really a 21st century field. So, although the ideas were out there a little bit in the 1990s, it’s really only been a – a very nascent field that’s really grown a lot in the 2000s.
[slide titled – History of GWAS – with the following bulleted timeline – 1996 – Idea first proposed by Risch and Merikangas; 2001 – First draft of the human genome completed; 2005 – First draft of the canine genome; 2005 – First human GWAS published; 2007 – Large complex trait consortium GWAS; 2010 – First canine GWAS published; 2016 – GWAS consortia studies typical]
And so, the first draft of the dog genome was published in 2005. So, certainly for dogs, the field is barely 10 years old.
And what’s occurred over time is the magnitude or the scope of these studies has increased, and now, these days, certainly in –
[Peter Muir, on-camera]
– human – human patients, consortia studies with very, very large numbers of people are now more commonplace.
So, when these studies are designed, there’s different ways the investigators can set up the design. More often than not, a – a – a -case control design is used, and that’s certainly something that we’ve used for our work where subjects are either cases affected with a disease or condition, or they’re controls.
But certain diseases can be – have a marker that is a quantitative trait that can be a continuous – a continuous variable. And so, some associations are quantitative trait associations.
[slide titled – GWAS study design – with the following list – Case control design; Quantitative trait association; Multivariate trait association; Single marker association; Regression models that analyze all maker effects together; Number of markers usually exceeds sample number]
It’s also possible if there are many markers for a disease to undertake a multivariate association, and this can sometimes have some advantages statistically.
When we studied marker associations in G.W.A.S., more often than not on the standard way of approaching this has been to do a single marker association. So, statistically look at one marker at a time. But, more recently, statistical models in this field have become very complex, and now there are regression models that will analyze all of the markers together. And I’m not a statistician, but this is a big challenge –
[Peter Muir, on-camera]
– statistically, both from, I think, designing the statistical algorithm, but also from a computational point of view. And one of the challenges for any of you who might have a statistical background is that the number of markers that are studied in these projects are typically much in excess of the sample number of subjects or patients.
So, I want to say a little bit about complex traits. And so, what I’m trying to illustrate here is that complex traits are on a continuum where, obviously, certain diseases may be considered to be principle –
[slide titled – Complex traits – featuring an illustration of a bar graph that has been divided from the upper left to the lower right to create two triangles within the graph. At the bottom of the graph (or left-hand triangle) is the label – Genetics, at the top of the graph (or on the right-hand upside-down triangle) is the label – Environment (e.g., Infectious Disease). On the y-axis is the label – Complex trait disease. The slide notes that the heritability of ACL ruptures in dogs is from .27 to .51]
– or exclusively an environmental condition. Just – so, one example might be some types of infectious disease. And at the other extreme, there are Mendelian diseases that are thought to be purely – have a pure genetic basis. And so, complex traits reside in the middle of this somewhere, and what we typically do is estimate heritability to try and understand how – what – what –
[Peter Muir, on-camera]
– what proportion of the trait might be explained by genetic background. And so, we’ve done that, and other investigators have done that for dog A.C.L. rupture, and it’s been estimated that heritability is in the range of 0.27 to 0.51. And so, this is a moderate value, which is good from trying to dissect the genetic background of the – of the disease.
One important concept to think about is when we’re undertaking studies of complex traits, is that the alleles that explain the genetic component of the disease are common in the population. So, when we look at a population of cases –
[slide titled – Complex trait alleles are common in the population – featuring two illustrations of circled 5 by 10 dots each representing cases wherein dark blue dots do not have symptoms and light blue dots do have the symptoms. The left-hand illustration is marked as Cases and has 12 symptomatic cases and the right-hand illustration marked Controls only has 6 symptomatic cases. The slide notes that the odds ratio is therefore 1.9. The slide also note that SNPs are used as allelic markers and that odds ratios typically range from 1.2 to 3.5]
– as in this diagram here in controls on the right, we can see that the risk allele, in the light blue in this diagram, is common in both populations. And so, when we calculate an odds ratio, typically the odds ratio for the risk alleles are not very big. So, they’re sort of moderate in size. And when we undertake this type of analysis, more often than not, we’re using Snips (S.N.P.s) as the allelic markers for the association.
[new slide titled – Complex trait GWAS needs many markers and subjects – featuring a graph titled – Rheumatoid arthritis – with -Log10 (P Value) Expected on the x-axis and -Log10 (P Value) Observed on the y-axis and showing a direct correlation between the two on the graph until there are slight variations in excess of 15. The slide also notes the following data – 500K SNPs; approximately 2,000 cases with approximately 3,000 control cases; QQ plot to examine markers]
Now, as I mentioned earlier, when we’re wanting to study complex traits, these studies typically need many markers and subjects. And so, there’s a very famous study published in 2007 that looked at 500,000 Snip (S.N.P.) markers in their study and had thousands of people. So, in this case for rheumatoid arthritis, approximately 2,000 cases and 3,000 controls. And so, one way of looking at these data is to make this plot on the right, which is referred to as a Q-Q plot, where the negative log of the observed P value of the association for each marker is plotted against the expected value from the chi-squared distribution. And so, each of these little circles represents one of the 500,000 markers, and what we’re interested in obviously focusing on is the markers that deviate from the identity line in this plot and are associated with the disease.
[Peter Muir, on-camera]
When these analyses are done, another important concept is that these large populations of humans and/or dogs can tend to cluster together. And so, we find that certain groups of individuals may be more similar genetically than other sort of subgroups within the sample population. And so –
[slide titled – Population structure – featuring a 3-D graph of clusters with Component 1 on the x-axis, Component 3 on the y-axis, and Component 2 on the z-axis. The clusters aggregate in the lower middle, upper left, and central areas of the graph. The slide also notes – Principle components of analysis (PCA) of a complex trait disease using pooled data from 7,393 osteoarthritis (OA) cases and 5,122 controls; and that it identifies clusters of individuals more closely related to each other]
– this is an important point because this clustering can lead, potentially, to spurious associations, or false positive associations. And so, the clustering of – needs to be studied and potentially accounted for in the statistical models that are used to analyze these data.
[new slide titled – Consortium studies – and featuring a graph with Observed associations on the x-axis and then specific Chromosomes on the y-axis and showing variations in data according to each chromosome. The slide notes that the graph represents – autosomal loci associated with rheumatoid arthritis. Dashed grey line is genome-wide significance; the slide also notes that there were 11,475 rheumatoid arthritis patients and 15,870 controls which were combined with 2,363 cases and 17,872 controls]
And, as I mentioned at the beginning, these days it’s more common to undertake, particularly in humans, consortia studies. And so, in this plot, which is referred to as a Manhattan plot, now we have the chromosomes across the genome and, again, each marker is a little circle. And on the X-axis here, we have the negative log of the associated P value. And so, one thing to notice as the number of patients increases, and obviously you can see that there are large numbers of patients here, you end up potentially with generating very, very significant P values. So, these ones are P is less than 10 to the minus 100. But, obviously, with many markers and so forth, then you need to correct the P value for genome-wide significance. And so, you can see in this study that the gray dotted line here represents genome-wide significance. And so, for this trait of rheumatoid arthritis, there are many markers across the genome that do associate with the trait. And so –
[Peter Muir, on-camera]
– therefore, rheumatoid arthritis is a complex trait with a genetic contribution to it. But the point is that you need very large numbers of subjects in order to gain enough power in your association to really understand what’s going on.
So, another important point is that with many of these complex trait diseases are quite common in human or dog populations. And so, as I mentioned earlier, we’re often trying to estimate heritability to sort of understand how big the genetic contribution to the condition might be. And so, typically when we do that, we’re estimating narrow-sense heritability, and this is either done from
[slide titled Common diseases, common variants featuring this bulleted list Broad sense heritability Ratio of total genetic variance to total phenotypic variance; Narrow sense heritability from SNPs or pedigrees Ratio of additive genetic variance to total phenotypic variance; GWAS SNPs often explain only a small proportion of phenotypic variance; Missing heritability? Structural variation (CNVs), epistasis, maternal/paternal]
– Snips (S.N.P.s) or from pedigree information. And one of the nice things about dogs is it’s actually fairly easy for us to obtain their pedigree information in order to construct a data matrix that can estimate heritability.
And what’s been learned from over time in this field is that when the early GWAS studies were done and they were studying a disease where heritability had already been estimated, it was found that the GWAS Snips (S.N.P.s) often only explained a small proportion of the phenotype. And so that sort of lead to this question of Well, is there – what about this missing heritability? And so, really, there’s sort of two ideas explaining that.
[Peter Muir, on-camera]
One idea is that there are other facets to genetic variation, such as structural variation in the genome, that could explain the missing heritability that therefore wouldn’t necessarily be captured by the Snip (S.N.P.) association. And the other idea is that there’s a tendency in the past for these studies to be underpowered. And so, if there are not enough subjects and not enough markers and you don’t have enough statistical power, then some of the genetic variation that may explain the disease could be missed or not captured in your association study.
So, just to summarize this first section, G.W.A.S. has become a powerful discovery tool for studying complex traits.
[slide titled Key Points with the following list GWAS is a powerful discovery tool for studying complex disease; For human disease, very large numbers of individuals are needed for a robust study; Phenotype is often explained by many genetic variants with small to moderate additive effects]
And we know, for human disease in particular, that large numbers of individuals are needed for a robust study, and obviously that is a huge amount of work and a lot of expense and so it potentially makes a research project really a substantial and very expensive undertaking but potentially very valuable.
And the phenotype in these diseases is often explained by many genetic variants with small to moderate effects that in general are thought to be additive.
[Peter Muir, on-camera]
So, we have this – common diseases have common variants in the population that have, in certain individuals, can come together, and if enough of them are there, disease will happen.
Here’s kind of a fun section now to say something about dog breeds. If you’re interested, there’s this really fabulous paper, which has one of my favorite titles I’ve ever come across in my career: Man’s Best Friend is Biology’s Best in Show.
[slide tilted Dog breeds featuring the quote Mans best friend is Biologys Best in Show]
And this is a review article published in 2010. And it’s well worth looking into, if you want to learn more about the background.
[new slide featuring a five by four grid of photographs of twenty different dog breeds]
So, obviously, dogs, as everybody knows, come in many different shapes and sizes.
[new slide titled Phenotypic diversity and featuring the following bulleted list More than 400 breeds Selected intensively for different physical traits; Selection occurred over approximately 15,000 years Most breeds only a few hundred generations old Unique genomic architecture]
And so, there – currently, there are more than 400 breeds of dogs that have been created by intense selection. And obviously these – these breeds have been selected to perform different functions. That was the purpose for breed creation in general in the past. And this selection process has occurred over about 15,000 years. But most modern dog breeds are not very old. They are only a few hundred generations old, and maybe as little as a hundred or more years old
[Peter Muir, on-camera]
– in some cases. And so, this has led to dogs having a unique genomic architecture as a species.
So, dog breeds are phenotypically very diverse. And as you can see in these images, there are dog breeds
[slide titled Phenotypic diversity featuring eight photos of dogs at work a dog herding sheep, a greyhound racing, sled dogs, a dog jumping to a lake rescue, two photos of dogs used for hunting, and a photo of a dog pulling a cart as well as a photo with two veterinarians holding a Chihuahua and a Great Dane. Additionally, at the bottom right corner of the slide is a graph of Dog Evolutionary History featuring time on the left-hand side and grey wolves on the far right who early in the timeline were domesticated and cross-bred with other dogs to create Village Dogs to create breed formations that lead ultimately to Purebred Dogs]
– used for herding, for hunting and coursing, for retrieving in water. Dogs obviously can be sled dogs. They can be for transportation. Down here we have a pointer, that’s also hunting, and some foxhounds. And so, one of the motivations for breed creation was to, when dogs had more of a working function in life
[Peter Muir, on-camera]
– was to have breeds that were optimized for different tasks. And so, in current times as a veterinarian, we may have this tiny little dog
[return to the Phenotypic diversity slide described above]
– like this Chihuahua, and then a really big dog, like this Great Dane. And so, theyre, phenotypically, they’re very, very different, but they are still members of the same species. And so, from an evolutionary point of view down here, dogs are domesticated from gray wolves. And so, we have this ancient bottleneck associated with domestication of the wolf. And then, up here, we have the more modern bottleneck associated with modern breed creation. So, there are two population bottlenecks to that
[Peter Muir, on-camera]
– evolutionary history of dogs.
And the genetic architecture of different breeds of dog has been studied, particularly with regards to this idea of ancient breeds of dog versus modern and how they may be related to the species of origin, the the gray wolf.
[slide titled Ancient and Modern Breeds featuring an illustration on the left-hand side of the slide of a Cluster Analysis of 43,000 Dog S.N.P.s leading to a half circle graph of the genetic relation of these breeds relations to the gray wolf with several wolf-like breeds on the top third of the illustration in blue and then the more distant relations in the middle and lower third of the illustration in red. The middle third of the illustration indicates the breeds that could be considered ancient breeds and the bottom of the half circle are considered modern breeds. On the right-hand side of the slide are photographs of two dogs who are closely related one at the top the Ancient Dyrehund and one at the bottom the Modern Australian Shepard]
And so, in medieval Europe, this breed of dog here would be referred to as a Dyrehund.
[the slide animates on the name of Modern equivalent of the Dyrehund Norwegian Elkhound – underneath the name Dyrehund]
And today we know this breed as a Norwegian Elkhound. And so, this would be a good example of a breed of dog that’s existed for a long time, going back probably at least a thousand years. This breed of dog down here, the Australian Shepherd, which was largely created in the U.S., is a modern breed that’s barely more than a hundred years old.
[new slide titled Linkage disequilibrium featuring the following points Non-random association of alleles at two or more loci that may or may not be on the same chromosome Stretches of DNA inherited together Longer LD in newer populations Broken down by generations of random mating; Dogs are not bred randomly to maintain breeds Lifetime of over 400 matings/liters for stud dogs]
So, before I move further into this section, I just want to say something about linkage disequilibrium cause this is an important concept. And linkage disequilibrium is the non-random association of alleles at two or more loci. And so, essentially what this means is that stretches of D.N.A. can be inherited together. And so, in newer populations, such as these modern breeds, long stretches of the D.N.A. are inherited together. And this is broken down by random mating. But, of course, dogs are not bred randomly. Gray wolves are or do, but not dogs. And so, just to give you a sense of that, popular stud dogs are are breeders that are highly valued or prized may sire more than 400 litters of puppies in their lifetime. So, dogs are pretty
[Peter Muir, on-camera]
– selectively line bred.
Now, when we’re interested in studying LD, we can typically do that with what’s called a triangle plot. And so, in this plot, you can see at the top here are some Snip (S.N.P.) markers, and then there’s a box that
[slide titled LD triangle plot featuring a graph of genetic markers across the top that are divided into Blocks where each marker is compared to other marker to form an inverted triangle shape. The marker comparisons are graded in color from red to pink to white to show how closely these markers are correlated red markers indicate strong correlations and white markers indicate no correlation. Therefore, red equals S.N.P.s in the LD. This process enables haplotype blocks to be defined]
– matches one marker with adjacent markers. And in each of these boxes, I think you can see that there’s a number, which is the correlation coefficient between the two. And so, this triangle plot is essentially a heat map where white is very little to no correlation and the bright red is a very strong correlation. And so, from the heat map of the triangle plot, we can determine that there are segments of D.N.A., such as this block here, that are inherited together. And so, this is referred to as a haplotype block.
[new slide titled LD in human populations featuring three LD triangle plots for (on the left, top to bottom) Caucasians, Chinese/Japanese, and Yoruba. In the middle of the slide is a graph with an arrow pointing down with Newer at the top and Older at the bottom. To the right is a small section of the top of the Caucasian LD triangle plot where there is a small triangle of red high correlation markers. At the top of this red inverted triangle a black mark is ticked at the top indicating a disease locus marker and to its right is another blue tick indicating the location of a S.N.P.]
And so, if we look in a human population, Caucasian population is a relatively modern population, and we can see quite a lot of red in this triangle plot. And then if we go down to the bottom here, the the Yoruba population is an ancient African population, and we can see that there’s a lot less red. And so, from a G.W.A.S. point of view, when these haplotype blocks exist in the genome, we just really need one marker in each block and obviously to test that block for association with a disease.
[Peter Muir, on-camera]
Now, what about the dog? Well, here’s the Caucasian plot at the top here, and here’s a chromosome
[slide titled LD in dog breeds and featuring two LP triangle plots at the top the same Caucasian human plot from the previous slide labelled Average LD block in Caucasians 23kb – and at the bottom the LP triangle plot for dogs labelled Average LD block in a dog breed is 1Mb]
– for a dog. And you can see, of course, that the dogs have a lot more red, and the blocks are much bigger. And so, within a breed of dogs, the average LD block is about a Megabase. Whereas, in human populations, it’s more in the, like, tens of thousands to 20,000 bases. So, big difference.
[new slide still under the title LD in dog breeds now featuring a graph with Distance in kb on the x-axis and Mean R-squared on the y-axis for several different breeds of dogs as well as grey wolves and village dogs at the bottom. The slide notes that R-squared is the coefficient of association of allele frequencies. Additionally, the slide notes that the LD extends approximately 10-100kb in human beings]
And this plot here just shows these data or or this concept in a different way, where we have distance across the genome here on the X-axis and the correlation R-squared on the Y-axis. And so, we can see that the gray wolves are – have fairly short LD and their correlation or their R-squared decays very quickly across the genome. Whereas, within breeds, and particularly some of these modern breeds, we can see that we can move a long way across the genome and the Snip (S.N.P.) markers are still very associated.
[Peter Muir, on-camera]
So, as I say, the contrast between dogs and people means that G.W.A.S. in dogs needs fewer markers to undertake the association because the LD blocks in the genome are much bigger.
And here we are again talking a little bit about the genomic
[slide titled Haplotype structure in the dog featuring four illustrations and two photos. The first illustration is another representation of the bottlenecks for dog breeds with a large arrow on the left labelled Wolf going down to an illustration of a wolf; around the middle of the arrow are three smaller arrows marked as Old bottlenecks for interbreeding of wolves and dogs and then these smaller arrows combine into a larger arrow labelled Dog. At the end of the Dog arrow the arrow again separates into four smaller arrows representing different dog breeds with an illustration of four dogs at the bottom of the arrow. To the right of the bottleneck illustration are three graphs from top to bottom labelled Pre-breed domestic dogs, breed creation, and Modern dogs and each show colorized genetic markers that differ from pre-breed to modern dogs. To their right are two photos stacked on top of one another the top one of two gray wolves labelled Short range LD, and the bottom one of a black Labrador labelled Long range LD]
– architecture of the dog. So, on this diagram, we can see the ancient bottleneck that I mentioned with domestication of the wolf. And then the modern bottleneck that I mentioned associated with breed creation. And so, modern breeds here have this add mixture of short linkage disequilibrium from ancient times and long linkage disequilibrium from modern breed creation. And this add mixture has not yet really been broken down by random mating. And so, that is what I mean by the unique genomic architecture that dog
[Peter Muir, on-camera]
– breeds have.
So, when we’re thinking about a disease and the genetic bases of a complex trait disease, the disease associated alleles likely proceed modern breed creation. And so, it’s pretty common as a veterinarian to recognize that there are common phenotypes between breeds. So, for example, A.C.L. rupture is common in different breeds of dog. And so, that suggests that there are probably common causal variants in the different breeds that contribute to the genetic risk.
[slide titled Disease-associated alleles with the following bulleted list Disease-associated alleles likely precede modern breed creation; Common phenotype between breeds suggests common causal variants; Need a SNP marker density to detect 5-10 kb ancestral types Two-phase study as a research approach Capitalizes on genomic architecture of dogs]
And so, when we’re thinking about G.W.A.S., we need a marker density that hopefully can capture the ancestral haplotype blocks, and therefore be of an appropriate density to sort of map the disease to the genome. And so, it’s been proposed as the field has evolved to do this in a two-phase way.
[new slide titled Two-stage discovery GWAS featuring an illustration on the left of two groups of four dogs (one white, the other black) at the top and the four rows of genetic markers for each of those four dogs. For the white dog group there is an area in all four rows of markers that is circled as an area of similarity for all four dogs in that breed. On the right, this illustration is labelled Stage One and it is noted that it is Within-breed GWAS, and the Discover loci is of approximately 1 Megabase]
So, in the first discovery phase, we take a single breed of dog. So the – obviously, here we have black and white dogs, but they’re the same breed so they’re the same shape. And we do our association and then find a locus that associates with, in this case, the color and expect that it’ll be a region of about a Megabase. And then
[new slide still under the title of Two-stage discovery GWAS labelled as Stage 2 and featuring an illustration on the left wherein the genetic markers that match from the first white dogs are compared to the genetic markers from different breeds. This is represented in the illustration with the original white and black dog illustration from the previous slide at the top of the illustration and then underneath those dogs and markers is another set of four dogs (both black and white) from a different breed and showing that within that different breed the same markers exist as the first breed in the case of the eight white dogs. Again, a dotted line is circled around all the markers that match both within the first breed and between the first breed and second breed. The slide notes that this stage is between-breed GWAS, and the Discover loci is of approximately 10-100 kb. The slide also asks the question of How best to do this?]
– if we have a second breed, or potentially several breeds, that have the same disease, we can take our loc – area of interest, our candidate locus from the first phase, and then we’ll finally map that or study that in more detail and take advantage of the short range, ancient, short range LD
[Peter Muir, on-camera]
– and, by studying it between breeds, potentially then narrow down the locus to a much smaller interval.
And so, this theory has been used to some extent in the field, but some of the evolution in technology, which I’ll say something about at the end of my talk, will – has sort of overtaken some of these ideas. And so, it’s still a bit up for discussion about how useful this type of approach is.
To give you a couple of examples, I don’t know if any of you are familiar with this breed, the Rhodesian Ridgeback, but maybe what you didn’t know is that some Rhodesian Ridgebacks don’t have
[slide titled Mapping the ridge featuring two photos and a graph followed by a bulleted list. The two photos are of the two variants of the Rhodesian Ridgeback breed of dog one with a prominent ridge on its back and the other without a ridge on its back. The graph titled Rhodesian Ridgebacks – maps the position of five chromosomes on the x-axis and the Log P of 1 million permutations on the y-axis and showing spikes in the graph in the chromosomes responsible for the ridged attribute in a study of 12 ridged and 9 ridgeless Rhodesian Ridgebacks. The bulleted list notes a 27K SNP array; a 750kb haplotype block on CFA18; 133kb duplication associated with the ridged phenotype]
– a ridge. [laughs] And so, that’s one example of a of a genetic phenotype that’s been studied and shown to you know, the genetic basis of the ridgeless dog was discovered by G.W.A.S.
[new slide titled Obsessive compulsive behavior featuring a screenshot of the top of an article from the research journal Genome Biology with the headline Candidate genes and functional noncoding variants identified in a canine model of obsessive-compulsive disorder and the following bulleted points Doberman Pinschers 87 cases, 63 controls (2014); 119 variants associated with OCD; Discovery of novel gene associations in humans (2016)]
In this – another example would be this – some breeds of dogs are affected with obsessive compulsive disorder. And there’s this nice paper that was published in 2014 that had sort of moderate numbers of dogs down here and found quite a large number of numbers of variants associated with the disease. And what’s interesting in this area is this is one example where initial discoveries in dogs were then led on to discovery of novel disease association in humans
[Peter Muir, on-camera]
– human patients.
So, just to summarize this section, there are different genomic animal models that geneticists have studied, but the dog, if you look down these column here
[slide titled Genomic animal models featuring a five column, nine row table that maps considerations of traits of interest; similarity of human disease; shared environment with humans; pedigree; population type; LD; SNP map; and mapping strategy between four genomic animals mouse; dog; horse; cattle. The dog stands out because it has complex traits, many similarities to human disease, a shared environment with humans, pedigree, breeds, long and short LD, a SNP map, and has two-stage mapping]
– is unique and very advantageous, particularly for complex traits, because there are many dog diseases that are similar to humans. Dogs have a shared environment with people, which is potentially important. It’s relatively easy to get pedigree information about dogs. There’s this very strong breed portfolio of different breeds that exist and this unique genetic architecture that exists in dogs too.
[new slide titled Key Points featuring the following bulleted list Dogs have a unique genomic architecture; LD within dog breeds is much greater than in human populations; Short range LD has not been broken down by recombination in modern dog breeds; Advantageous for studies of complex disease in particular]
So, the key points here is dogs have a a unique genomic architecture where the linkage disequilibrium within a breed is much greater than in humans. And in modern modern breeds, short range L.D. has not been broken down by recombination. So, this is thought to be very advantageous for studies of complex trait disease.
[Peter Muir, on-camera]
Okay, so the next section I want to do is say something about the disease of interest that we’ve been particularly focused on for quite some years now, which is this condition of A.C.L. rupture.
So, the cruciate ligaments, there are two cruciate ligaments, the anterior and posterior cruciate ligament that exist
[slide titled Cruciate ligament anatomy featuring a photo of the top of a knee bone together with an anatomical illustration of the knee joint below it showing the locations of the anterior cruciate ligament, the posterior cruciate ligament, the lateral collateral ligament, the medial collateral ligament, the lateral meniscus, and the medial meniscus. The slide also includes the following list Two intra-articular extra-synovial ligaments Anterior cruciate ligament, Posterior cruciate ligament; Together with the LCL and MCL stabilize the knee joint. The slide also shows an illustration of a normal ACL/PCL knee and one whose ACL has been torn]
– inside the knee joint. And we can see here, this one here is the posterior, and this one here is the anterior. And you can see that they cross over each other and sort of twist around each other, and this crossing over is obviously what gave them the name. And together with the collateral ligaments, so the lateral and medial collateral ligaments, these are important stabilizers of the knee joint. In the anatomy of these ligaments is very similar in dogs and people.
[Peter Muir, on-camera]
So, in human patients, this is an important condition. It has a gender predisposition and obviously is very common in athletic and sporting people
[slide titled Human ACL rupture featuring an illustration of how the ligament tears at the top of the slide and underneath a photo of Green Bay Packer Jordy Nelson laying on the field after suffering an ACL tear. The slide also notes the following statistics Common condition, female predisposition annual incidence of 68.6 per 100,000 person-years; Non-contact mechanism landing and pivoting; Life-changing health problem 78% risk of knee arthritis after ACL rupture; 11.8% risk of contralateral rupture; 80,000 graft repair surgeries annually at a cost of 1 billion dollars]
– but it’s also common in sedentary people too. And if you follow the Green Bay Packers, then this is an important player here who unfortunately experienced this injury. And it is a life changing health problem. If you are a human patient with A.C.L. rupture, you’re very likely to get knee arthritis as a result of the injury. And you’re also at some risk, actually, of developing a second A.C.L. rupture. And most ruptures occur not from contact injury but from landing or twisting and that type of movement. And it is sufficiently common in surgical treatments done a lot to essentially
[Peter Muir, on-camera]
– mean that it’s an economically important disease to the human population, and certainly large amounts of money are spent on the healthcare for both human patients and for canine patients as well.
There have been some genetic studies in humans. And so, some candidate gene studies have shown significant associations. And the focus of interest for the candidate gene studies has been to look at genes that regulate ligament matrix homeostasis and
[slide titled Human ACL rupture genetics featuring a microscopic image of a ruptured tendon as well as an illustration of the different parts of the fiber bundles that make up the tendon including the fiber, the fibril, the sub-fibril, and the micro-fibril. The slide also includes the following bulleted list Candidate gene studies significant associations ligament matrix proteins collagen gene polymorphisms; no heritability estimate; GWAS has not been performed to date]
– competition – composition. And this has typically been the collagen genes were obviously been the initial focus in this way because the collagen protein has extremely important role in the hierarchical architecture of tendons and ligaments and is very important for mechanical properties. But today, as far as I’m aware, there’s not heritability estimate for A.C.L. rupture in people, and there are no published G.W.A.S. discovery studies.
[Peter Muir, on-camera]
So, what about dogs? Well, this condition is very common in dogs. It’s the most common reason that dogs have orthopedic surgery at the veterinarian. And in high-risk breeds, it actually affects about 10% of the breed, which is huge if you actually think about, like, how many breed how many Labradors or Rottweilers or Newfoundlands might there be in the whole of the U.S. So, this incidence
[slide titled Dog ACL rupture featuring the following bulleted list Common up to 10% breed prevalence approximately 100 times higher incidence versus humans; Risk factors for disease initiation breed; Risk factors for disease progression Synovitis and OA knee morphology?]
– comparing the species means that in the high-risk breeds it’s about 100 times higher incidence than human patients. And so, the biggest known factor for initiation of the disease is breed. And I’ll say – comment on that in a minute. For disease progression, other factors have been implicated, and without going into a lot of detail, inflammation within the knee joint and the morphology or the anatomy of the knee are two things that have been studied quite a lot in
[Peter Muir, on-camera]
– that regard.
This – there was a nice paper published in 2008 that looked at the epidemiology of the disease and essentially created a nice list of
[slide titled Breed association with ACL rupture noting that it is the only important factor for disease initiation and containing a table of dog breeds that are most likely to suffer from ACL injury including breed, number of dogs, percentage with injury, OR, and P value. The top five dog breeds to suffer from this injury are Newfoundland, Rottweiler, Labrador Retriever, Bulldog, and Boxer]
– many common breeds of dog with their breed prevalences. You can see here, up at this list, the top is a Newfoundland with 8.9% prevalence. And the next breed is the Rottweiler, 8.29%. So, very common in certain breeds of dog. And, obviously, third on the list here is the Labrador Retriever.
[slide titled Discovery of GWAS in the Labrador Retriever featuring a photo of five golden retriever puppies at the top of the slide and a photo of a chocolate retriever underneath the photo of the puppies. The slide also has the following list Most common pure-bred dog in the USA 5.79% rupture incidence approximately 100 fold higher incidence than in human beings; Heritability in dogs estimated at .27 to .51 for ACL rupture no estimate in humans]
We chose the Labrador Retriever for our studies, and you may say, Well, why did you do that if it’s not most commonly affected? But it is the most common breed of dog in the U.S. And so, if you put the prevalence and the numbers of dogs together, it means that there are lots and lots and lots of Labradors out there that experience this disease.
So – and as I mentioned earlier, we – there are heritability estimates in the dog that certainly suggest that this is a disease with moderate heritability.
[new slide titled Dog age and ACL rupture featuring a bar graph with age of onset (bar one) versus age of presentation (bar two) on the x-axis and number of dogs on the y-axis. The graph has a curve that leans left for the onset of the disease more prevalent in early years and a curve that has more of a normal bell curve for the presentation of the disease more prevalent in middle age. The slide also notes that this is for the Labrador Retriever 6% is more than 8 years]
So, I just want to say a little bit more about the sort of epidemiology of the background of the disease in dogs. And so, we know a fair bit about its age at presentation. And this is important when we’re thinking about, like, the controls for our G.W.A.S. And so, we can we know that once the Labrador Retriever gets to about eight years of age, it’s pretty unlikely to experience cruciate rupture. And so, it’s very interesting. I’ve talked to owners of quite young Labradors that might only be a few years old, and they have disease, and the dog’s sort of got
[Peter Muir, on-camera]
– reduced mobility. And I’ve talked to other owners that might have a 15-year-old Labrador that goes hunting with them for pheasant hunting, and the dog’s fine and it doesn’t have the disease. So, it – this disease does have some impacts on dog mobility.
This table just summarizes a little bit of information about sex steroids and the risk of the disease. Now, obviously in dogs
[slide titled Reproductive status noting that ACL rupture risk increases with neutering in both males and females some variation between studies. The slide also has a five row by three column table of group of dogs female, spayed female, male, and castrated male and the percentage of population and OR both spayed females and castrated males have a percentage above 4 as opposed to percentages of about 1.5 for dogs that have not been spayed or neutered]
– it’s pretty routine in in the U.S. to either spay female dogs or to neuter the male dogs. And so, whether it’s a male or a female dog, neutering and – does an influence on the disease, and the risk of the disease goes up.
And so, this little table just summarizes that. We can see here for the spayed females and males that the odds ratio for ACL rupture is higher.
[new slide titled Bilateral ACL rupture featuring two graphs comparing contralateral effusion to contralateral osteophytosis both with days on the x-axis and percent survival on the y-axis for Grades 0 3. The slide also includes the following bulleted list Bilateral ruptures at initial presentation 11-17%; Subsequent contralateral rupture greater than 50% at one year; Synovitis influences disease progressive]
We published a paper from our lab some time ago. Were very interested in studying second cruciate ruptures in dogs. And this paper was published in 2014. And it’s in Plus One, so it’s freely accessible, and I’d say it’s an interesting one to read if you’re interested. So, what the what we did is look at dogs that had diagnosed with A.C.L. rupture in one knee, and then what we did is actually studied the second knee. And so, we made made radiographs of the knees and graded them for radiographic change, for either the volume of joint fluid, or essentially a marker of inflammation in the joint, and also for the amount of arthritis that we could see on the radiographs.
And these curves are what are referred to as Kaplan-Meier curves. So, they’re a survival curve where the terminal event, either A.C.L. rupture, causes the curve to drop down. And so, obviously, if you can see at the top here, the red curve obviously looks different from the other two, and it is. And so, the dogs with more severe effusion or inflammation radiographically in their joint at diagnosis quite quickly went on to get a second rupture. Whereas the dogs with less severe change were less likely to get a second rupture over time. And you can kind of see the same pattern to the data when we grade the radiographs for osteophyte inflammation.
[Peter Muir, on-camera]
So, in the vet school these days, when we have a lame dog and we make the diagnosis, we often take radiographs, and we actually take radiographs of both knees to sort of stage the dog and warn the owner and say something from a predictive point of view of how likely it is for the dog to be developing a second A.C.L. rupture.
And so, from a mechanism point of view, what does this data say? Well, it certainly suggests is one piece of data that suggests that synovitis, or inflammation inside the knee joint, does influence the progression of the disease over time as more and more fibers rupture in the ligament.
So, here are some arthroscopic images. Just like in human surgery, in the vet school it’s pretty routine these
[slide titled knee arthritis in ACL rupture dogs featuring four arthroscopic images of dogs knee joints (clockwise) a normal knee joint with Femoral condyle indicated, a knee with a partial ACL rupture, a knee with a partial ACL rupture with the ACL and the PCL noted, and a knee with an ACL rupture]
– days to do arthroscopic surgery. And so, in the normal joint, we – the – the synovial lining of the joint should be almost white with very small number of blood vessels. But as the ligament starts to rupture, the synovium becomes fronded and proliferates and much more inflamed. And down here on the right you can see that the synovium’s intimately associated with the cruciate ligaments. And so that inflammatory change sort of makes sense as to how that inflammatory change could then influence the homeostasis of the ligaments that reside inside the knee joint.
[Peter Muir, on-camera]
And I have a little video to play.
[slide also titled – Knee arthritis in ACL rupture dogs – and featuring a video of an ACL surgery with an arthroscopic probe which Peter describes below]
So, here, we have this little arthroscopic probe, and what it’s doing now is showing you that the splits and damage to the fibers are actually the posterior cruciate ligament. So, it’s very common that both ligaments are damaged, but the rupture event is typically in the A.C.L., and that’s really from a biomechanical reason. And so, now we can start to see some of the inflammation in the joint. And down here is the torn A.C.L. And if we look carefully at the end, you’ll see it in a moment, the end of it has a little blood areas. So, the ligament is trying to heal. The end of the torn ligament is trying to heal, but because it resides in this intra-articular environment, once the ruptures –
[Peter Muir, on-camera]
– occurred normal wound healing just can’t occur. And so, once the rupture has occurred, the ligament won’t heal.
Okay. So, other factors that have been studied include body condition. So, higher body weight increases rupture risk.
[slide titled – Knee morphology – featuring a photo of the top of the knee joint and the following list – Ligament complex ACL & PCL – Located within the intercondylar notch – wrap around each other; Intra-articular but extrasynovial; Little blood supply from attachments to bone]
And knee morphology can influence this too. So, these ligaments, as I mentioned, are intra-articular but extrasynovial, and they wrap around each other inside this notch, the intercondylar notch in the – in the – in the femur or the thigh bone. And an important point is that little blood supply to the ligaments is derived from the bony attachments. It all comes from the overlying soft tissue.
[new slide still under the heading – Knee morphology – featuring five photos and a graph. The first two photos are an x-ray view looking at the top of a normal knee and a knee with ligament damage. The second set of three photos are x-ray photos from the side of the knee showing a normal knee and two photos of knee ligament repair. The graph plots tibial plateau angle in degrees on the x-axis and ligament survival time on the y-axis with most data points laying in the middle of the graph. The slide notes two outcomes of rupture – narrowed intercondylar notch and increased posterior tibial slope of TPA]
A couple of things that have been studied from a morphology point of view is, as the arthritis develops, you can see that here in this image, that the notch of the femur can narrow, and that notch is thought to impinge the cruciate ligament and promote rupture. And, also, dogs and people have a posterior or a slope to the tibial plateau, as you can see here, relative to the long axis of the bone. And that slope has maybe a small effect on risk of rupture. And so, in – in this data here, we’ve plotted that time to contralateral rupture we talked about a couple slides ago against the TPA or the posterior slope angle. And we can see that is a slight drop-off to the regression line. And over here on the right, interestingly, in this model, biomechanical model from these images here, we can see that when the cruciate ligament is cut in a cadaver knee that the tibia sublux – subluxates in an anterior direction. And when we level the plateau surgical, as per this cut here, we can actually mechanically stabilize the joint without actually repairing the ligament. And so, there’s been great interest in this morphology, particularly the posterior slope, or the T.P.A., tibia plateau angle, because it’s become incorporated into a very common –
[Peter Muir, on-camera]
– surgical treatment that’s used clinically to treat dogs. In the vet school, we operate on dogs almost every week with this surgery.
So, clients come in and we do this surgery called the tibia plateau leveling osteotomy for the client and dogs. It usually works really well. Helps the dogs with mobility. But it’s a very common surgery and done commonly in specialty practice and other vet schools throughout the U.S.
So, just to summarize, dog A.C.L. rupture is an excellent model for human A.C.L. rupture. And this topic is, like, an important example of One Health research.
[slide titled – Key points – with the following bulleted list – Dog ACL rupture is an excellent model for human ACL rupture; Important example of One Health research; Bilateral rupture common in both species; Disease risk influenced by sex steroids; Dogs and humans have a shared environment]
Bilateral A.C.L. rupture is common in both species. The disease risk is influenced by sex steroids. And, importantly as well, for some diseases, dogs and humans have a shared environment.
[Peter Muir, on-camera]
So, now I’m just going to talk a little bit, having given you this background about some of the work that we’ve been interested in doing. So, as I mentioned, I think, briefly, we conducted a within breed association study, and hopefully it makes sense as to why we did that. And it was a case control study.
[slide titled – Within-breed GWAS – featuring two x-ray photos – one of a damaged dog knee and one of a repaired dog knee. Additionally, there are the following notes – Study was in Labrador Retrievers – Case control phenotyping and n was greater than or equal to 100 per group for robust power. The Phenotype-negative controls were greater than or equal to 8 years, and having a normal orthopaedic exam and stifle radiographs]
And when we started recruiting, our aim was to recruit more than a hundred dogs, a hundred Labrador Retrievers in each group. And because of this age effect, we only used dogs more than eight years of age for our controls. And we also wanted to confirm that they had normal knee radiographs. And obviously, when diseases have a prevalence of 5% to 10%, if you just get some healthy dogs, then obviously, statistically –
[Peter Muir, on-camera]
– it’s quite likely you could accidentally have some disease dogs in your control group. And so, this phenotyping piece is actually very important.
There is a standard Snip (S.N.P.) genotyping array that’s available – been available for some time for dogs. And so, one of the very commonly –
[slide titled – SNP genotyping – with the following list – Blood or salvia; Illumina Canine HD Bead Chip – 173,662 SNP markers – more than 70 markers per megabase]
– used ones is from this company called Alumina, and that’s what we used for our work. And so, the Alumina array has over 170,000 markers on it.
[new slide with an output of data for the array which consists of a long string of letters representing genes – lots of As, Gs, Ts, an Os]
This is what the data looks like. I mean, I think from computational things, people are always like, Well, what does the data look like? Well, this is what it looks like.
[laughter]
So, essentially, those 170-odd-thousand markers are in a row. So, each dog is a row of data. And we have the first few columns are the phenotyping and clinical information, and then the rest of the data are the genotypes. And so, the – the – the data is in two files. There’s this file and then map file, which is –
[Peter Muir, on-camera]
– a map of how the order of the Snips (S.N.P.s) relates to the genetic information in the data – in the genotype file. So, these are large files, obviously, and there’s quite a lot of learning needed to understand how to run the programs to handle these large data sets, which is definitely something my labs grown up a lot with over the last few years.
When we attain the genotyping data, we need to do some quality control to make sure that we have good quality data. And without going into a lot of information or a lot of detail, if we have samples with a low genotyping
[slide titled Data Quality Control with the following bulleted list PLINK; Sample genotyping rate greater than .95; SNP genotyping rate greater than .95; Minor allele frequency less than .05 Genotyping arrays use common SNPs; Hardy Weinberg equilibrium – HWE less than 1.0E-7. The slide also notes approximately 120,000 SNPs for analysis]
– rate, that infers, suggests bad data, and so they would be filtered out. If we have Snips (S.N.P.s), individuals Snips (S.N.P.s), with a low genotyping rate, then we’ll filter those out. And if we have Snips (S.N.P.s) that don’t vary very much in terms of the genotypes in the population – so they have a minor allele frequency of less than 5%, then they’re typically filtered out because they’re not really gonna inform the association. And then we also look for Snips (S.N.P.s), which is usually not very many, that might deviate from Hardy-Weinberg equilibrium. So, when we’re done with this filtering, the 170-odd-thousand –
[Peter Muir, on-camera]
– usually goes down to something like 100,000 to 120,000. Something like that. Which we have then left to perform our association analysis.
So, as I mentioned at the beginning, there’s a number of different statistical models that have been described as to how you can do this. But, basically, many of them are a variant of a linear mix model. And these –
[slide titled – Statistical models – featuring the following list – Linear mixed model – Single marker analysis – PLINK, GEMMA, GCTA; Regression models that analyze all markers together – PUMA, BayesR]
– algorithms essentially look at the markers one at a time. So, a single marker analysis. And these programs here are acronyms that we’ve used for some widely adopted programs in the field. We’ve also begun to study more – more advanced or sophisticated regression models that analyze all the Snip – Snips (S.N.P.s) together, which is computationally more intensive, but as desktop workstations have become more powerful, this type of analysis has become more routine.
[new slide titled – Genome-wide significance – featuring this bulleted list – Bonferroni correction too conservative; Permutation testing – Rerun GWAS 1,000 – 10,000 times, randomly permuted genotypes; Candidate regions at P<5E-04 for pathway analysis; Room for improvement!! The slide also contains a sample graph for the Irish Wolfhound with expected LogP on the x-axis and actual LogP on the y-axis and showing a direct correlation that deviates about halfway up the graph]One interesting topic here is to think about genome-wide significance, where, obviously, we set an alpha level of 0.05%, 5%, 0.05, usually in scientific experiments. But we need to correct that for - in these association studies. And so, it's a big topic as to how do you do that. Bonferroni correction is too conservative cause, as we've discussed, the markers are not independent cause they're linked together in haplotype blocks. So, other approaches that have been considered is to do some sort of permutation testing with random phenotypes to estimate genome-wide significance, or sometimes just empirically you can set a lower, less stringent threshold for association which is often used for pathway analysis to look - [Peter Muir, on-camera]- for enrichment of a biologic signaling pathway. But there's definitely room for improvement statistically on this topic. And this is something that we've been discussing a lot amongst the lab, my lab, and people that - statisticians that we collaborate with here on campus. And a recent paper did propose a more sophisticated way of trying to create a breed specific genome-wide significance because the LD can vary between breeds, where permutation testing data was used to draw a Q-Q plot with empirical confidence intervals from the data. And then, obviously, the - where the Snips (S.N.P.s) leave the upper 95% confidence - [return to the previous slide labelled - Genome-wide significance - described above]- window, if you draw that across, in theory, that should set what a robust genome-wide significance is. And so, that's one new idea that's been out there as to how to do this. [slide titled - Discovery GWAS results - featuring two graphs. The first graph is a Q-Q graph using GEMMA with expected Log -10P on the x-axis and actual Log-10P on the y-axis and showing a direct relationship with a few outliers on the end of the graph line. The second graph is a Manhattan Plot using GEMMA with the Chromosomes on the x-axis and the Log-10P on the y-axis and showing a forest of spikes for the empirical data. The slide also notes that the number of dogs involved in the study was 237]In our initial discovery G.W.A.S., this was our Q-Q plot. And so, we did find a locus that met genome-wide significance. And when we set a less stringent cutoff for pathway analysis, we found, as you can see here, many loci across the genome that looked like they contributed to the disease. [new slide still under the heading GWAS Discovery results - featuring the following list - Single SNP on CFA24 met genome-wide significance - 10 genes in this locus (500kb flanking regions) - DNA repair, angiogenesis, and immune response pathways; 129 ACL rupture candidate SNPs; LD clumping = 99 candidate loci]In the most significant locus that we found, there are about 10 genes that reside in this locus that regulate a number of different biological phenomena. And so, obviously as we move forward, that's one area, the canine genome that we're interested in studying a lot more detail. And overall, we identified 129 associated Snips (S.N.P.s). And when we did our LD clumping, some of them clump together, which left us with 99 - [Peter Muir, on-camera]- candidate loci. This graph here is actually a fairly recent product of some of the work that we've done. And it may not look like much but - [slide titled - Bayesian mixture model (Bayes R) - featuring a graph titled - Genetic Architecture of ACL Rupture in the Labrador Retriever - with the various chromosomes on the x-axis and the Variance Explained on the y-axis for three different sets consisting of 41 (in grey), 338 (in orange), and 3228 (in blue) SNPs, respectively. The slide notes a total of 3607 SNPs with non-null effects fitted in the model]- the people in our lab put huge amount of effort to compute this graph, which is actually quite - took quite a lot of effort to compute it. But this output is from this Bayesian mixture model called a program called Bayes R, which analyzes all the Snips (S.N.P.s) together. And the idea with that type of analysis is more - better able to capture weaker associations between certain Snips (S.N.P.s) and the trait. And so, when we fitted our data to Bayes - using the Bayes R model, Bayes R said that there are 30 - just about 3,600 Snips (S.N.P.s) that associate with the disease of A.C.L. rupture in the dog. And the nature of the mixture model then is that Bayes R partion - partitions the Snips (S.N.P.s) with non-zero effect. So, Snips (S.N.P.s) that have some effects on the trait into essentially Snips (S.N.P.s) with large and gray medium, in orange, or small, in blue, effects on the disease. So, when you look at the gray bars here across the genome, and the X-axis obviously plots the percent variants explained, you can see that the gray sort of maybe explains about sort of two-fifths of the genetic background of the disease. And so, obviously, those Snips (S.N.P.s) are relatively small number at 41. But obviously, the orange and blue also make a big contribution to the genetic background to the disease. And, obviously, the medium and small effect Snips (S.N.P.s) are much larger in number. So, what this analysis does is tell you quite a lot of information about the genetic architecture of A.C.L. rupture in much - [Peter Muir, on-camera]- more detail. And it also tells you that it's highly polygenic. So, again, just to summarize. Dog A.C.L. rupture is highly polygenic. [slide labelled - Key Points - featuring the following bulleted list - Dog ACL rupture is highly polygenic; A large proportion of phenotypic variance is explained by a few SNPs; Many common variants with small to moderate additive effects also explain a large proportion of phenotypic variation]Quite a large proportion of the phenotypic variants is explained by only a relatively small number of Snips (S.N.P.s). And so, obviously, going forward, the genetic loci that those Snips (S.N.P.s) reside in are definitely going to be of focus for more investigation as the discovery work moves forward. But we also have this sort of problem or phenomenon that a large proportion of variation is also associated with Snips (S.N.P.s) or genetic variants that have just very - [Peter Muir, on-camera]- small or moderate size effects on the disease. So, just to finish up, I'm going to say a few words about future work. What about things that we would like to do going forward? Well, we would like to do a replication study. So, it's very - with discovery G.W.A.S., we talked about the idea that false associations can occur. It's diff - its statistically a difficult sort of field. And so, one thing that it's a good idea to do is to see if you can replicate your results in a different, second population. [slide titled - Replication and validation GWAS - featuring two photos - one of a chocolate Labrador Retriever and underneath it a photo of a Rottweiler. The slide also included the following bulleted list - Replication GWAS - Second Labrador Retriever population (with-in breed); Validation GWAS - Rottweiler - Expect to discover common risk loci for ACL rupture; Control breed (Greyhound) GWAS?; Consortium GWAS project]Obviously, replication in a second population of Labrador Retrievers. But it's also a good idea to try and validate your discovery results in a different population with a different genetic background. So, our goal right now, and we're right in the middle of doing more recruiting, actually, for both breeds, is to look at the Rottweiler. And I mentioned Rottweilers actually have a much higher breed prevalence than Labrador Retrievers. Although, Rottweilers are not as common or popular breed of dog. We've also been thinking about the idea, and still thinking about it right now, is should we really try and study some sort of a control breed? And there an iconic control breed with a very, very low prevalence of the disease would be the Greyhound. And Greyhounds have largely been selected to race. And obviously if they're racing, they need to be orthopedically fit, and so that sort of makes sense that the alleles - [Peter Muir, on-camera]- that might associate with A.C.L. rupture or other orthopedic diseases are not very prevalent in that breed. And we're also trying to explore this idea of doing some sort of consortium project with other vet school investigators or other research groups to try and address this piece about statistical power and get really large numbers of dogs into an association analysis. Why is this work important? Well, obviously, this is discovery research. So, we expect to make discoveries about novel genetic variants that contribute to A.C.L. rupture. We need to sort of then obviously -[slide titled - Impact of the work - featuring the previous photo of the chocolate Labrador Retriever as well as a photo of a litter of Labrador puppies below it. Additionally, the slide contains the following bulleted list - Causal variant discovery - confirm causal relationships experimentally - develop new drug treatment from a target; Genetic testing informs clinical management - selective breeding (dogs) - risk management]- confirm those relationships experimentally. But as that work unfolds, there's the potential that we could identify a drug target that could come up with a better treatment for the early phase of the disease perhaps. And in veterinary medicine, obviously dogs are bred selectively so, clearly, genetic testing could potentially have a - a role in breed improvement and also in risk management. This is an acquired disease, so if we knew a priori that a particular individual patient had a very high genetic risk - [Peter Muir, on-camera]- for the disease, then we could - we - veterinarians could provide advice to those owners about how to try and mitigate that risk. And, finally, I think that what I'd like to highlight is that in veterinary medicine One Health Research is a very important topic. And, certainly, it's a topic that's capturing more attention in terms of sort of human medicine and - and human -[slide titled - Comparative and clinical value - featuring the logo for One Health Research and a photo of a group of three dogs and a photo of a crowd of people and two arrows pointing back and forth between the two photos. At the bottom of the slide are the precepts of One Health Research - Discovery of susceptible loci, identification of biological pathways, identification of biomarkers, and clinical care all leading to diagnosis, prediction, prognosis, novel treatments, and response to treatments of disease]- health sciences research. And so, I think what I've hopefully shown you today is this is a good example of One Health Research where work in dogs can benefit people and vice versa. And so, G.W.A.S., as susceptibility loci are discovered and we can learn more about biological pathways, then we can potentially identify better markers for disease, and that could therefore improve clinical care. And, obviously, clinical care can be at the level of diagnosis, prediction of disease, and so probably for these complex traits, very common both in human populations and dogs, genomic prediction may well be actually a more important test - [Peter Muir, on-camera]- clinical test in medicine. Because these diseases are highly polygenic, it may well take genomic prediction but with a large panel of markers to actually predict the risk well. And, obviously, those types of - that type of knowledge could inform prognosis about disease, it could lead to development of new treatments, and also provide perhaps better ways of monitoring response to treatment. So, I'd definitely like to leave you with this sort of thought as you walk out of the room this evening to think about, you know, the overall value of One Health Research. So, obviously I haven't done this work on my own, and there's quite a long list of people here that I'd like to acknowledge. [slide titled - Acknowledgements - listing the people and organizations that have helped the Vet School in their research]Various individuals that worked in - in the lab in the vet school with me. Faculty collaborators on campus, and one of the great things about U.W.-Madison is it's a very rich academic environment where there's lots of opportunity to talk to interesting people about your work. [Peter Muir, on-camera]And I've also been lucky enough to have support from a number of organizations across the country. I'd also like to acknowledge some of the financial support that my lab's - [slide still under the heading of - Acknowledgements - listing the financial organizations that have helped with the research]- had to support this work. [new slide titled - Thank you - featuring the photos of four researcher that Peter would like to thank - Susannah Sample - NIH K01 Scholar, Emily Binversie - MS Student, DVM Student, Lauren Baker, MS - DVM Student, CIBM Scholar, and Alex Piazza DVM, Research Fellow]And, in particular, I'd like to thank these individuals who have made substantial contributions to the work over the last year. And Lauren Baker has just recently graduated from vet school in May and is now studying for a PhD in my lab. And so, she's going to be a - a big player in this work that I've described over the next year or so. [Peter Muir, on-camera]Alright, that's all I have. So, I think there may be time for some questions. [applause]
Search University Place Episodes
Related Stories from PBS Wisconsin's Blog

Donate to sign up. Activate and sign in to Passport. It's that easy to help PBS Wisconsin serve your community through media that educates, inspires, and entertains.
Make your membership gift today
Only for new users: Activate Passport using your code or email address
Already a member?
Look up my account
Need some help? Go to FAQ or visit PBS Passport Help
Need help accessing PBS Wisconsin anywhere?
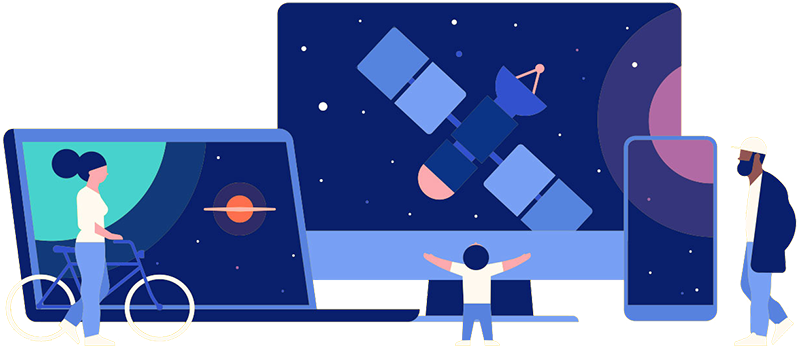
Online Access | Platform & Device Access | Cable or Satellite Access | Over-The-Air Access
Visit Access Guide
Need help accessing PBS Wisconsin anywhere?
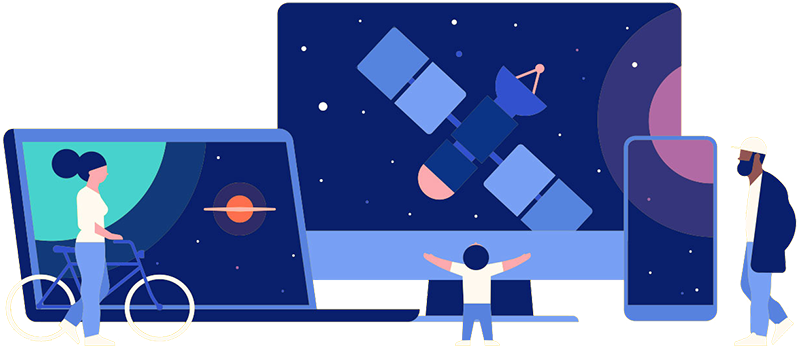
Visit Our
Live TV Access Guide
Online AccessPlatform & Device Access
Cable or Satellite Access
Over-The-Air Access
Visit Access Guide
Follow Us