Sarah Corso: Hello, everyone.
Welcome, and thank you for coming to tonight’s Badger Talk, brought to you by UW Connects.
My name is Sarah Corso, and I’m a librarian and access services manager here at the Oak Creek Public Library.
I’m pleased to introduce our guest, Dr. Thomas Beatty, assistant professor in the Astronomy Department for the College of Letters & Science at the University of Wisconsin-Madison.
The presentation he is giving this evening is called “How Can We Find Other Life in the Universe?”
Dr. Beatty is from Riverside, Connecticut, and grew up sailing off the coast of New England.
He has a bachelor’s degree from Harvard University, a master’s degree in physics from MIT, and a PhD in astronomy from Ohio State University.
Before moving to Madison, he worked as an instrument scientist on the James Webb Space Telescope, where he helped build one of Webb’s cameras and helped to operate it once Webb reached space.
His research focuses on measuring the atmospheres of exoplanets– planets around other stars– to figure out what they are made out of and what their climates are like to understand how planets form and to search for life elsewhere in the universe.
He has also discovered nine new exoplanets, none of which he got to name after himself, sadly.
Today, we are asking, “Are we alone in the universe?”
This question has been asked for over 2,000 years, and now we possess the tools and techniques necessary to answer it.
Dr. Beatty will discuss what we want to look for on other planets to see if there is life, as well as how this search has begun at UW using current and future telescopes.
Please join me in welcoming Dr. Beatty.
[audience applauding] – Thomas G. Beatty: Thank you very much.
That was a very nice introduction.
It’s a pleasure to be here.
I actually, coincidentally, am in the universe.
And, really, how can we find life elsewhere, and how are we trying to approach this problem at UW and, in general, in the astronomical community to try and solve this question?
And I like to begin talks like this with just sort of a picture of the night sky and the thought, the idea, that in many ways, astronomy is sort of, you know, the second-oldest profession in the world– being an astronomer, right?
It’s probably the oldest science that we have in the sense of you could imagine people, very early people in Africa, just looking up at the night sky and wondering what is going on up there, what’s going on with the stars, and that, fundamentally, is what my job is.
What we do as astronomers today is, we’re still looking up at the night sky, and we’re still trying to figure out what’s going on with the stars.
And, in particular, I work on exoplanets, so I look for planets around other stars, and we’re trying to measure these planets around other stars and figure out if there’s planets like the Earth elsewhere in the galaxy.
That question, the idea that there might be another planet like the Earth somewhere up there, probably– it’s a little bit fuzzy– was probably first posed by the ancient Greeks about 2,500 years ago, probably the first person to, at least, write that question down.
Maybe people thought of it before that.
So we’re really thinking today very concretely about how do we answer this question: Is there a planet like the Earth somewhere else in the universe?
And it’s a question that people have been wondering about for over two millennia, and we’re very close to actually answering it, and that’s the topic of what I’d like to talk to you about tonight.
So I wanna begin by just talking about what is an exoplanet, right?
What does that mean when I talk about an exoplanet?
What are those?
So in the solar system, we have our planets in our solar system.
We have all the planets that go around the Sun, so the planets go around the Sun, those are planets, and an exoplanet is simply a planet that orbits a star other than the Sun.
So just like the Sun has a solar system of planets around it, there are other exoplanet systems around other stars elsewhere in our galaxy that we know of.
In galactic terms, most of the planets that we are looking at are very close by in the terms of the universe, right?
All the planets that we know of are within the Milky Way galaxy.
This is a nice map produced by Professor Bob Benjamin, who’s at UW, right, showing what the Milky Way looks like, so all the planets that we’re thinking about looking at and know about are within the Milky Way galaxy, and they’re actually very close to the sun, within the Milky Way galaxy, right?
This red circle is about the limit of detection, so where we’ve seen exoplanets, how far out we’ve seen them.
If I zoom back out on the entire galaxy, you can see we’ve actually been probing a very small segment just of our local corner of the universe, right?
We now know of a lot of exoplanets, but all of them are very close by on an astronomical scale.
They’re all within our galaxy, and they’re all close within our galaxy.
All together, over the last 25 years– 30 years now, we’ve now discovered over 5,000 exoplanets.
I think the count as of this morning was 5,761, right?
And if you look at this graph, right, you can see there’s actually a lot of sort of features here you could think about teasing out.
So I’ve marked on this slide, the capital J is where Jupiter is, and the capital E is where the Earth is, and you can see we have a whole bunch of planets that are like Jupiter, we have a whole bunch of planets that are like Earth, and a whole bunch of things in between, and, in particular, we also have a whole bunch of planets that don’t even really exist in our solar system, right?
A lot of the ways that even we astronomers conceptualize what these planets look like is by thinking about how do they compare to a planet in the solar system.
Are they, like, a hot Jupiter?
Are they a super-Earth?
Are they a warm Saturn?
We use words like that, but there is a whole class of planets, like super-Earths or sub-Neptunes, that don’t actually exist in the solar system.
And so, by finding all these new planets, we’re actually uncovering new processes and physics that we didn’t know about before we knew that these planets existed.
And this has been a lot of the work of the last 30 years in this field, is finding new planets, getting this number to go from one– the first one was discovered in 1995– getting it from 1 to 5,761, right?
That’s taken a lot of work, and it’s taken over two decades of people finding new planets around other stars.
Most of these are discovered indirectly, right?
We actually– of those over 5,000 exoplanets, we only have direct images where we can see them in a photograph or an image for only a handful, probably about a dozen.
Most of these, we never actually see the planet directly.
Instead, what we see is, we see the planet’s effect on the star that it’s orbiting, right?
So one way, on the left there, one way that we see the planet affecting its star is that we can look at the star, we can measure the velocity of the star, and we see the star moving back and forth a little bit because the planet as it orbits the star is tugging on the star, and the star itself is moving just a little bit as the planet orbits around it, and so we can see that motion of the star with our telescopes, right, so we see the star moving, and we know it must be a planet based on that motion.
We never actually see the planet itself.
Another way we discover planets, that’s on the right, is, we look, and as a planet goes in front of a star, it will block out part of that star, and the light from the star will go down.
So Jupiter going in front of the Sun would make the Sun get dimmer by about 1% for a couple of hours, and then it would go back up again, right?
And we can detect planets that way.
Actually, that technique has gotten– We’re all sufficiently good at doing that that I’m actually with a couple of students looking, trying to detect a new planet– or confirm a new planet from the roof of the Astronomy Department in downtown Madison sometime next week.
So that sort of measurement for a Jupiter in front of a Sun-like star has gotten relatively easy with modern technology.
Planets like the Earth is a lot harder, right?
The Earth is 10,000 times less of a signal that we have to measure, right, but the real point to just take away from this is, we have a lot of ways to detect planets.
We’ve found a lot, and we know a lot from just their masses and their radii and their sizes and where they are relative to their stars, but we still never actually see the planet directly, right?
We just see what it does to the star.
We don’t have a lot of information about what is happening on the planet itself.
And so if we’re thinking about detecting life on an exoplanet, figuring out if it’s habitable, if it’s like the Earth, right, what we will start with is something like this.
This is a simulated light curve, so measurements of the brightness of a star, as a planet like the Earth goes in front of it.
So if we were looking at the Sun and the Earth passed in front of it, this is what we would see.
It’s a very small signal.
We’d have to measure it from space.
And what we learn if we were looking at the Sun is, we could, you know, learn that the planet was about the size of the Earth.
We maybe could get a mass by looking at the motion of the Sun, so we knew it was the mass and the radius of the Earth, and we know how far away it is using things like Kepler’s laws and the orbital period.
But the important thing is, if we were doing this on the solar system, we wouldn’t just get one planet like the Earth.
We’d get two, right?
If we were looking at the solar system with our current technology, we would say the solar system has two habitable planets because we have the Earth and we also have Venus, and Venus is nearly the same mass and radius as the Earth, and it’s closer to the Sun, but it’s not that much closer, and the theory we have about habitability says that Venus probably should be habitable.
There’s a lot of other factors, of course, that have made Venus not habitable, but we don’t fully understand them yet, right?
So one of the tasks that we have, and this is what I do, is trying to figure out, right, if we have a planet like this, how do we determine if it’s like the Earth– that is, a nice beach vacation– or if it’s like Venus and it’s gonna melt your spacecraft 30 minutes after you land, right?
This is actually true.
The only landers that we have that have ever reached the surface of Venus are a couple of Soviet landers that landed in the 1980s, and they only lasted on the surface for about 30 minutes before they got squished by some combination of crushed by the pressure and dissolved by the sulfuric acid that’s present in Venus’s atmosphere.
And actually, a really fun story is, we don’t actually have a very good measurement.
This isn’t that funny, this isn’t that fun a– It’s a fun story for us.
It’s not a fun story for the scientists involved.
So we don’t really have a good measurement of the surface of Venus, like, what the, like, dirt is in that picture, because one of the– So the first Soviet lander that landed on Venus, that had a camera, but the lens cap didn’t come off, so they didn’t get any pictures, and so they said, “Okay, for the next one, we’re definitely making sure the lens cap is coming off,” right, and it did.
This is where this picture comes from, right, so the lens cap comes off as it’s drifting down, and we get these nice pictures.
But one of the next experiments they had– and, remember, they only have about 30 minutes, and then the whole thing is done because the spacecraft has melted.
One of the experiments they were gonna do was, there was a spring-loaded arm inside the spacecraft that was gonna, you know, a catch-release.
As the spring shoots the arm out, it goes into the dirt, and then they retract it back, and they can get some dirt, and they can analyze it, but it’s a one-shot thing.
You can’t retract the spring, you know, once it’s fired, right?
You fire it, you’re done.
And so they’re down, they’re all ready, they’ve released the spring.
The arm shoots out, and it shoots directly into the lens cap that, even though it popped off about 500 feet up in the air, has landed directly next to the spacecraft and directly where this arm was about to shoot out into, and so that is why we don’t actually have a good measurement of what Venus’s surface is like.
But okay, so we can’t do that with exoplanets, right?
We can’t go there.
We can’t try and dodge lens cap covers to measure what these planets are like.
We can’t even see them most of the time, right, so we need to come up with other ways of figuring out what they’re like.
And so the main way in which we do this is, we look at their atmospheres by watching as transiting exoplanets– Those are the exoplanets that pass in front of their stars.
As they pass in front of their star, some of that light, some of that starlight that’s getting blocked by the planet, it isn’t entirely blocked, right?
It filters through the outer edges of the planetary atmosphere, and that outer edges imprints on that starlight.
The starlight keeps coming to us on Earth from the outer edges of the atmosphere imprint on it, and that is what allows us to measure the atmospheric composition of these planets, right?
We never actually see the planet.
We just see it pass in front of the star, but because of that little fringe of atmosphere, we can measure what’s going on in the exoplanet atmosphere.
And this was first done about just under 20 years ago for a planet called HD 219–209458.
Most planet names are complete telephone numbers.
They’re named after star catalog names.
Like I said, you never get to name an exoplanet after yourself.
You get to name it after the star catalog that it’s in.
But if you do this long enough, you get to remember the telephone numbers, as well, so it was first done on a planet called HD 209458, it’s well-known in the community, and now we’ve done it for a whole bunch of exoplanets using telescopes like Hubble and things from the ground.
But the really exciting thing is to do this with the James Webb Space Telescope, and this is the new telescope that was just launched two years ago, and is really– You know, saying it’s gonna usher in a new era of, you know, exoplanet atmosphere studies is probably understating what’s about to happen over the next couple of years.
So launch for James Webb was Christmas Day of 2020 from French Guiana.
As was mentioned in the introduction, I worked on one of the Webb instrument teams, one of the JWST instrument teams, specifically on a camera called NIRCam.
That’s one of the 4 instruments on the spacecraft, and so all of us were online to watch the launch.
Actually, what happened is, I was at my in-laws in Chicago, and I have two young children, and so we did presents for about an hour, and then launch was at 6:15, so we woke up at 5:00 A.M., did presents for about an hour, and then we all went and watched the launch for– you know, in the middle of Christmas morning.
And I will tell you, my mother-in-law, who’s very nice, she’s a wonderful woman, and you guys would love her, but she also, you know, she thought, she understood how important this was to me professionally, right?
Like, if this exploded on launch, it was unclear I was gonna have a job the next day, right?
There was a lot riding on this, but also her conception of this was, if the rocket ignited and we were going, we were done, right?
And in reality, you know, the rocket can blow up at any time, right?
And once you get off– actually, the real sticking point was as soon as JWST came off the top of the rocket, it was on battery power, and the batteries last for about 20 minutes, and so it has to deploy a solar panel and start generating electricity as soon as it can, and if that solar panel didn’t deploy, you had 20 minutes to figure out what was going on, and then you were done, right?
There was no way to recover it.
And one of the best things I ever saw in my life was, there’s video feed from the launch of, you know, Webb detaching from the upper stage, and you could immediately see the solar panel coming out, and we were all on the internal comms, and you could hear them reporting that power was starting to be generated, and everybody started cheering because we knew that, you know, the first hurdle was overcome.
But my mother-in-law, who’s a wonderful woman, thought as we were, like, 30 seconds in and the rocket was firing that, you know, everything was great, and so we’re 30 seconds in and the rocket’s firing, and she comes over, and she’s like, “Thomas, you did it.
Like, it’s all happening, congratulations,” and I just was like, “Not now, Cindy.”
[audience laughing] But she’s very nice.
So there’s a couple of exciting things about Webb.
One of them– and this still boggles my mind, even having known this for, you know, years, right– is that Webb is about three times as large as Hubble, but the mirror weighs half as much, right?
And the collecting area you get from a mirror is the area, right, so if it’s three times larger, that means you get almost 10 times as much light in the same amount of time, so it’s about 10 times more powerful than Hubble.
It weighs half as much.
It’s really an incredible machine, and the amount of attention to detail and design that went into it really just boggles the mind.
The other difference from Hubble is that Webb is an infrared telescope.
So Hubble observes a little bit into the infrared, but fundamentally is an optical telescope, but observed very close to the wavelengths– to the kind of light that we see with our eyes.
And Webb’s observes very different wavelengths and longer wavelengths, the infrared, you know, closer to the heat that you see in images of heat.
So one thing this allows us to do is it allows us to probe deeper into the atmospheres of exoplanets.
So this is a picture of Jupiter taken in the infrared.
You can see the sort of bands and clouds where, you know, you maybe are used to seeing in optical images of Jupiter.
Here’s the Great Red Spot, just for reference, right, that sort of dark, circle-ish thing.
The bright parts here are really hot parts of Jupiter, right?
What that means is, those bright parts are you are seeing through the clouds into the interior of the planet, where it is hotter.
So the darker parts are the upper clouds that usually that’s what we see when we look in visible light and hotter and deeper, we can actually see with these infrared images, and we usually can’t see that in the optical.
We also see different molecules in the infrared.
We get to see a lot of methane.
We get to see a lot of ozone and water and carbon dioxide, which we couldn’t really see easily, particularly in exoplanet observations.
As a little bit of color, so launch for Webb was Christmas morning, and then we all spent the next six months traveling out to Baltimore one week– a little over a week a month for six months straight to operate the telescope during commissioning, because it launches and then it needs to be checked out before NASA will, you know, accept it as an operating observatory.
So, as some local color, here’s what my office looked like for most of those six months during commissioning, helping to run the telescope.
The really helpful thing, right, is that most of this is color-coded, right?
It turns out that there are actually very few people that know what all those numbers mean, right, not even me.
I knew my part, but most of these, what was happening, like, other people understood.
It was sort of a fascinating project that no one person can really hold it all in their head, except there was one guy, right?
The real job that we all had was to look at these screens and if anything turned red, we were gonna call Carl, right, ’cause Carl knows what all these numbers mean and knows if they turn red, what to do about it, or at least he knows who to talk to, right?
I’ll also say that we– most of the shifts, they were eight-hour shifts, and we were there most of the day.
We did have to observe.
We had to be there overnight some weeks, which I felt was particularly unjust for a space telescope where you don’t actually usually have to be up overnight.
The one nice thing is, we actually ended up watching a fair amount of movies, and this is a photograph of– So we were all in a room called Science– the Science Instrument Room, the SI Room, and right next to us in a separate room was Flight Operations, Flight Ops.
This is a photograph of all the Flight Ops desks.
They were the people who actually sent commands to the spacecraft.
And you will notice, if you look very carefully, right up here at that image, Flight Ops is currently watching Olympic curling on the big screens at the front of the room, right?
So most of the movies we watched were actually stuff that Flight Ops set up and put on, and they also get to choose the movies, and they had a very interesting– I really wanna know who was in charge of this ’cause we spent about a week working through all the Princess Diaries movies.
Then we did really trashy sci-fi movies.
Have you guys ever seen Moonfall?
Like, the moon comes alive and crashes into the Earth.
Yeah, that’s what we watched.
It was not what I would have expected for a bunch of NASA engineers in the other room.
Okay, so that’s a little bit of color from commissioning, but to get back to the serious part of the talk, right, how can we use JWST to search for life, right?
Now that we have this machine, this observatory in space, we want to use it to search for life with its giant mirror.
How do we do that?
So one thing is that often when we think about other life in the universe, right, a lot of what we think about is other intelligent life, right?
Like, we’re thinking about some sort of, like, face-sucking alien that’s gonna, like, read your mind or, like, you know, the alien from Aliens, right?
But there’s other kinds of life out in the universe, right?
And when we’re talking about in the astronomical context searching for life, we’re not just meaning intelligent life.
We also mean plants and bacteria and animals and, really, any kind of life, any kind of life out there that would be something like life on Earth.
So what that means is that a lot of people spend a lot of time thinking about what, exactly, do we mean by life, right?
Like, what does that actually mean?
Like, what is that?
And I’ll say it’s a topic of a lot of debate.
I’ve gone to a lot of conferences where people spend a lot of time discussing exactly what it means.
My personal favorite definition– Well, I’ve written it on the slide, right?
It’s, life is some sort of self-organized chemical structure that alters its environment and can reproduce.
To me, that seems like a pretty general, basic statement that tries to be pretty agnostic about what, exactly, life is, right?
So we’re not just looking for intelligent life.
We’re looking for just life as a general category of objects.
On Earth, all of the life on Earth is based fundamentally around DNA, right, which is some assembly of hydrogen, oxygen, nitrogen, carbon, and phosphorus, right?
The backbone of most of these molecules are carbon molecules.
That’s why we say that life on Earth is carbon-based.
It’s why organic chemistry exists as a subfield.
Organic chemistry is just carbon molecules and carbon chemistry, right, because it’s a fundamental part of all of our lives of everything on Earth.
And life on Earth also requires liquid water, right?
The reason for that is that all the chemical reactions that happen in your body need some sort of liquid suspension for it to occur in, right?
It’s hard to get chemistry to happen just dry on the floor.
We need some liquid to allow things to mix, and you can dissolve a lot of things in water, right?
Water is sometimes referred to as the universal solvent.
So life on Earth, we need liquid water.
We need a lot of carbon, oxygen, nitrogen, maybe a little bit of phosphorus.
Okay, so what about life on other planets, right?
If we think about what life looks like on Earth, then what does that tell us about where do we look for life on other planets?
Well, first thing is, we need an ocean, right?
If we want to find life like the Earth, we need to have liquid water present on the surface, enough liquid water for it to be a fundamental part of the biology, just like it is for us.
It’s actually an interesting point about the Earth’s oceans, right?
And it’s actually a very fine point that a lot of people are thinking about as we think about oceans on other worlds.
‘Cause if you think about it, if Earth had a little bit more water, just a little bit more water, the surface would be entirely covered by ocean, right?
There’d be no land.
It would just be water all the way down.
Well, until you hit the sea floor.
And if we had a little bit less water, there wouldn’t be any oceans.
We’d be something like Mars, where there is water, but it’s frozen into the polar icecaps, or it’s in ice underground.
It’s not just standing water on the surface.
So Earth, at least right now, seems to be a pretty finely balanced midpoint between those two extremes, and it seems very convenient that we happen to live on the one planet that just happens to hit that exact point.
Now, maybe that’s reading too much into it, right?
We only have one example.
Maybe we’re gonna go out there, and we’re gonna discover tons of ocean worlds, and it’s gonna be great, or maybe we’re gonna discover tons of life that lives on ice and melts it– or I don’t know what, right?
But just something to think about.
But the fact that we have oceans on Earth is actually very coincidental, seems to be very finely tuned.
Another thing we also need is, we need the right temperature, right?
We need the right amount of water.
We need the right temperature, right?
If Earth gets too hot, if it moves in a little bit closer to the Sun, all the water boils off into steam.
If it moves too far out, it all freezes, it turns into ice, and we’re a giant snowball.
So one of the fundamental things we wanna look for when we’re looking for potentially habitable planets is we wanna look for planets that are at the right distance from their stars, that are in the habitable zone, or the Goldilocks zone, the right temperature so that liquid water can exist on the surface of these planets.
And we actually have a bunch of these, right?
These are actual real planets.
The images are not real, right?
These are all artists’ conceptions, but these are all actual planets that we have detected and are about the size of the Earth and are at the right distance from their stars to support liquid water on their surfaces, right?
We actually know about a whole bunch of potentially habitable planets that we could go and search for life on them.
So, okay, so we’ve detected a whole bunch of planets.
We have this nice light curve, right?
What happens next?
How do we figure out if we have a beach vacation or if we have Venus, right?
Now that we know these planets are out there, how do we look at their atmospheres and figure out if they actually might have life?
Well, we primarily wanna look for three things in the atmosphere.
So first, we wanna look for water, right?
We wanna confirm that there’s some sort of water in the system.
Well, it’s the right temperature for liquid water, but we don’t know if liquid water is there until we see it.
We also want to look for oxygen, right?
20% of the air you’re breathing right now is oxygen.
It’s a major component of our atmosphere on Earth.
And we also wanna look for methane, but probably not too much, right?
It’s one of the major biological products from life on Earth.
The main biosignature, right, thing you would want to look for in an atmosphere, is oxygen.
It’s often considered a primary biosignature, and the reason for that is that without life on Earth, the level of oxygen in our air would be about a million times lower, right?
So when the Earth first formed, there was almost zero oxygen in the atmosphere of the planet, and the only reason why the air you are breathing right now is 20% oxygen is because life evolved and started photosynthesizing, so the fact that 1/5 of our air is composed out of oxygen molecules is solely due to life on Earth.
If all the life on Earth suddenly blinked out of existence tomorrow, all the oxygen would be gone from our atmosphere within probably a couple thousand years, very quick on an astronomical time scale.
So if we were looking at an exoplanet and we saw some water in the atmosphere and we saw oxygen, I mean, there’s probably a couple more steps, but you could probably think about writing your Nobel Prize acceptance speech at that point, right?
It’s a really strong signal that we’ve got a good, promising candidate.
One of the best candidates to look for this, a lot of people are spending a lot of time looking at this, is a system called TRAPPIST-1.
These are a whole bunch of planets, and a bunch of them are in the habitable zone, so it’s a very small star.
They’re all very close in, but they’re all in the habitable zone.
Two of these have already been looked at with James Webb.
I was actually involved with looking at– the team that looked at the innermost one, TRAPPIST-1b, where we measured what it looked like for the first time, and, unfortunately, the answer was, it looks like a bare rock in space, that the atmosphere has been completely blasted off.
I was actually sitting with the guy who was analyzing the data.
The whole observation was designed to take, like, five separate transits, and we were gonna have to add them all together before we thought we were gonna get anything, and I was sitting next to him when he first downloaded the first data from the first observation, and you could just see it, like, in the first one.
And we both looked at each other, and we’re like, “Well, that is not really the answer we wanted,” because it means it was a very hot rock, the fact that we could see it the first time.
So actually, it’s telling us a lot about how planets lose atmospheres if they’re very close to their stars, and then the second one, TRAPPIST-1c, is more Venus-like, it turns out, from other Webb observations, but there are a lot of people looking at E and F and G to try and get much finer observations of their atmospheres.
So there’s a lot of people looking for Earth-like life.
Another thing we can do is look for life, but maybe not quite as we know it, right, not quite like the Earth.
And so I mentioned that the Earth– We seem to be very finely tuned in terms of having land and an ocean, right?
What if we looked not at planets like the Earth, but what if we looked at water worlds, giant water worlds that are somewhere in between the Earth and Neptune?
So these are called hycean planets.
This was sort of a category of planets that was suggested a couple of years ago, and the idea is that it’s not a planet like the Earth.
It’s probably about five or even ten times more massive than the Earth, much larger, but there’s a lot of water, and there’s a hydrogen envelope, a hydrogen atmosphere that insulates that water, and so you can have a liquid water ocean at the right temperature, at about room temperature, and you could maybe have volcanism or something going on underneath, and so you could have a liquid water ocean at the right temperature with energy being put into it, and you could have life present on these planets, right?
That wouldn’t be life like the Earth.
It may not even be intelligent life, but it would be life, right, and that would be very exciting to find, and these are much bigger planets, and so they’re much easier to measure the atmospheres for.
And so actually, at University of Wisconsin, we actually have time on a telescope out in Arizona.
We get about 20 nights a year on the WIYN telescope, and one of the things that I do is I use some of that time to look for planets like these, and I actually have a student working with me right now who’s working on this.
He’s actually– Pretty excitingly, he’s actually found two of these this spring where we’re starting to write up that paper right now, right?
Two new planets in this category that this fall, we’re gonna apply for James Webb time to try and look at what the atmospheres are like, right?
We’re actually– Well, Arizona is two hours behind us, so they’re probably opening up probably in about an hour from now to start observing tonight, and they’re gonna be observing again for us tonight, as well, right?
So we’re actually doing this search tonight to look for planets like these.
And the exciting thing is, is that, because these are so large, they’re actually pretty– the atmospheres are pretty detectable with James Webb observations, so JWST in one to four transit observations, which is a pretty reasonable request to ask for time on the telescope, could look at these planets and could detect signatures of life in the atmosphere, right?
And this is actually something that’s happening, right?
These two have both been observed, right?
This one, the results were announced last fall.
This one, the results were announced last spring.
Top one hasn’t been looked at yet, but there’s a lot of people putting in proposals.
We’re putting in proposals and getting time to look at these planets, and we’re finding new ones to try and find life on these planets, and we think we have a pretty good shot if it’s there.
The really fun part is gonna be once we get something that we think might be life because I’ve been describing to you how we need to understand the biosignatures in the atmosphere: We need to look for water; we need to look for oxygen, maybe a little bit of methane– and what’s gonna happen if we do this successfully is, we’re gonna get something that looks like an atmosphere like Earth, and then we’re gonna have to figure out if all those gases and things we see in the atmosphere are produced by life or if they’re produced by things like volcanoes or geologic processes.
And figuring that out is gonna require not just astronomers and people who know how to operate JWST and know how to measure exoplanet atmospheres.
We’re gonna need planetary scientists.
We’re gonna need geologists, biologists, ocean scientists, everybody, right?
All these other bits of the puzzle that we all need to get together and figure out what’s going on, and that’s the idea behind this thing called the Wisconsin Center for Origins Research, WiCOR, that is, hopefully, starting this fall.
We’re provisionally starting– We’re provisionally a center right now, but we’re hopefully officially gonna be a center this coming fall that is specifically designed to do just that, to get all these different people together from all these different departments at UW-Madison to start talking and figure out how can we plan for detecting life and how can we design observations that are gonna do it?
And once we’ve done it, how do we prove that it’s life in a way that’s convincing to the rest of the scientific community?
And it’s a project that’s gonna be extremely difficult, and it’s gonna require a lot of different people all working together, sharing their own specialized knowledge, but we think it can be done.
Okay.
I like this flowchart.
Usually, actually, when I show this slide, I don’t really have much to say here.
I just sort of like the way it schematically lays out what’s going on.
It actually reflects a lot of my thinking on this as well.
I will say, my particular favorite is that the successful detection of life is just labeled by “champagne,” right?
Right, this is the basic outline of what we need to do.
We need to find planets.
We need to figure out if they’re like the Earth.
We need to run through a bunch of tests in the atmosphere to see what’s in it, and at the end of the day, hopefully, we’ll work our way down to champagne and then have to start talking to the geologists and the oceanography people to figure out if this is actually feasible.
Okay, so I’m gonna end with this picture again, right?
So, as I mentioned at the start of the talk, you know, people have been thinking about is there life elsewhere in the universe, right?
The question “Is there a planet like the Earth somewhere else?”
for at least 2,500 years, right, over 2,000 years, probably more than that, right?
That’s a pretty– I can imagine somebody asked that question a very long time ago.
And even in the astronomical community, in exoplanets, people have spent the last three decades, even since we knew about exoplanets, looking for planets like the Earth, and we’re starting to find them.
And one of the big revolutions in astronomy is, the search for biosignatures and the search for life has progressed from something that, you know, nobody really takes seriously to something that a lot of people are trying very hard to do successfully, and it’s an incredibly exciting time to be a part of astronomy, right, ’cause, like, the stuff that we are doing at UW, you know, we’re assembling this team that’s gonna hopefully do this.
We’re observing tonight, like I said, to try and find new planets that we can look at with instruments like the James Webb to try and find life elsewhere.
Like, the hunt is on, and it’s happening now as we speak, right?
Like, people are doing it.
This isn’t some future thing maybe in a couple of years, we could think about, you know, looking for life on other planets.
People are actively searching right now, and if it’s out there and if it’s on some of these big, sort of, hycean planets, I think we have a pretty good shot of doing it in the next 10 years.
Probably earlier than that– by 2030, I bet, right?
That means that we are right on the edge of answering a question that people have thought of for 2,500 years and wondered about the answer for.
And so it’s a very exciting time to be in astronomy, and it’s a very exciting time to think about finally knowing the answer to that question after over two millennia of people wondering about it, and it’s, you know, it’s why I get excited about going in to work in the morning.
It’s also why I like giving these talks, right, to tell you guys about that and hopefully get you excited about how really close we are to actually doing this.
You know, I said it’s gonna be very hard, right?
It’s very difficult observations.
It’s a very complicated analysis problem, and it’s gonna be a pretty subtle chain of logic that’s gonna get us there, but, you know, I think it’s gonna be probably one of the most complicated and difficult but probably greatest, you know, scientific endeavor that astronomy has done for the last couple hundred years.
So, hopefully, we’re gonna get to champagne in the next couple of years, and if we do, I will come back and tell you guys all about it in another Badger Talk.
So thank you, and I’ll take questions.
[audience applauding] Uh, yeah?
– Audience Member 1: Is it possible that some of the moons, both within our solar system and outside, could contain life?
– Right, so is it possible that some of the moons in our solar system or outside could contain life?
Yes, and, actually, the planetary science community is very excited about going out to the moons of Jupiter and Saturn, as well.
So there’s one mission called Europa Clipper that is launching in six months that’s gonna travel to Jupiter’s moon Europa, and Europa is very exciting ’cause it’s covered in a surface of ice, right?
It’s cold enough that ice on Europa is like rock on Earth, so the surface is solid ice, solid water, and then the mantle, what we have for lava, is just liquid water, so it’s liquid water ocean, and there seems to be volcanoes underneath.
We know that ’cause we can see ice volcanoes on the surface, so it seems like it’s a liquid water ocean and there’s volcanoes adding energy into that system, and so it’s very possible there’s life there, and so there’s Europa, there’s Enceladus, which is around Saturn, is another exciting one, and Titan around Saturn is in many ways actually pretty similar to early Earth before life evolved, or Archean Earth, actually.
That’s very early life on Earth, when we had a lot of methane in our atmosphere, and so all three of those, people are spending a lot of time thinking about and developing missions for to try and find life, and people have been searching for moons outside the solar system, moons around exoplanets, and there’s been a couple of tentative detections, but nothing quite yet.
But people are thinking about that as well.
So, yeah, moons in the solar system, people are– the planetary science community is thinking about that a lot and actually getting stuff out there ’cause Europa Clipper will launch soon.
Uh, yeah?
– Audience Member 2: What future telescopes are being planned that you can get a closer look at these?
Is there any in the works right now?
– Yeah, so what future telescopes are being planned?
So the next big NASA telescope mission will be something called Roman, which is launching in ’26 currently.
That’s more of a exoplanet detection and population survey than a characterization mission.
Probably, there are a couple of other missions that are gonna launch that will do exoplanet atmospheric characterization, but they’re not gonna greatly exceed JWST’s capability.
The next big thing that everybody is thinking about is a mission that right now is called the Habitable Worlds Observatory that, you know, is currently scheduled for 2035 for launch, but put your own handicap on that.
It’s probably later than that, but that is the idea.
We’re gonna put a very large, you know, eight-meter mirror in space, and that will be, in principle, able to directly image planets like the Earth and actually measure the atmospheres directly.
We don’t need to do it indirectly by waiting for planets to pass in front of a star.
So Habitable Worlds Observatory, HabWorlds, if it launches, should be able to do this.
But that’s another good point, right?
HabWorlds, like, is just– It’s just an engineering problem right now, right?
It’s an engineering, and it’s a “convince the Congress to give NASA enough money to build it” problem, right?
We could do this very quickly if we wanted to.
It’s just, we need to do a couple of engineering checks and stuff, right?
This is no longer science fiction.
This is like, “Let’s go to Congress and ask for money to do it.”
– Audience Member 3: You said if all life on Earth died… – Mm-hmm.
– That within a couple thousand years, the oxygen and the atmosphere would be gone.
– Thomas: Yeah.
– Why?
What would it make it go away?
– Right, so why does the oxygen disappear if life disappears?
So oxygen, I mean, it’s sort of the reason why it’s so fundamental to life, ’cause oxygen is incredibly reactive with stuff.
So oxygen, it will disappear into rocks through rust.
It will, you know, go into– Sort of like acid rain, it will go into the water.
And it’ll go into the seafloor in, like, lime or something.
It just gets absorbed into rocks and water and geologic processes very quickly.
– Audience Member 3: So it’s not that it would be– like, just drift away or be blown away by the solar wind.
– Oh, no, yeah, so it doesn’t escape from the Earth.
It doesn’t– It still stays here.
Just, it’s gonna go into the rocks.
We’re gonna turn into Mars, effectively.
We’re gonna turn into a rusty red planet if all the life disappeared, yeah.
Yeah?
– Audience Member 4: So how many times do you have to observe an exoplanet before you actually confirm that you think it’s an exoplanet, and how do you track its location so that in the future, you can have the Webb check back?
– Right, so how many times do you have to look at an exoplanet to confirm it, and then how do we track it so we can look at it later?
So confirmation observations are– So actually, the planets that I’m working on with a student right now are probably a good way to explain what the process is.
So there’s a NASA mission up right now called TESS, which is just scanning the sky for new exoplanets, and so it finds something that looks like a transiting exoplanet, like a planet going in front of its star.
It’s measuring the brightness of all the stars, but one of the problems is that there’s, for lack of a better word, a lot of things that go bump in the night that look like planets but are not.
There’s a lot of things like stars and other, you know, things that aren’t planets that can mimic those transit signals.
So we get a lot of candidates and then we have to refine that, so we look at it with telescopes like WIYN, right, that we have in Kitt Peak to look for the star moving, and if we see the star moving, that tells us the mass of the object, and so we get a good mass.
Usually, we’ll look at it with bigger telescopes on the ground to make sure it’s happening on the star we think it’s happening on.
And so that’s most of the confirmation process.
If you can see the planet go in front of the star, if you can measure the mass from the star wobbling back and forth, and if you can demonstrate that there’s no nearby star that you’re getting confused with, then you’re pretty much there, and you know there’s a planet around that star.
And as a part of that, we generate what’s called, you know, an ephemeris for the planet, you know, a prediction for when transits will occur.
And for the first couple of years, those are probably good to a couple of minutes, but over time, those will drift, and some of the planets that were discovered 10 years ago, we don’t actually know the transits to better than half an hour or maybe even hours.
So for JWST observations, you need to know it to minutes, and so there’s actually efforts that a lot of people work on, that a fair number of people work on to go back to older planets and look at them again to get more, sort of reset that drift on the clock and figure out when it’s gonna happen to refine the ephemerides so we don’t lose ’em and we still know exactly when they’re going to transit.
So it’s a lot of people working to do both parts of that.
Uh, yeah?
– Audience Member 5: In the red ring on the map of the Milky Way, like, where you found the planets, how far away are we talking about as far as, like, light-years, and how far back in time are we looking?
– Right, so how big is that red ring in light-years, and how far away are we looking?
So most of the planets that we know about are probably– I’m doing a conversion in my head ’cause most of us, a lot of astronomers use parsecs instead of light-years.
Yeah, if you guys ever wanna be cool at an astronomy party– I don’t know why you’d be going to an astronomy party, but if you want an invite, I think Mary has my email.
Yeah, talk about things in parsecs.
That’s how you know you’re on the in-crowd.
So probably out to about 500 light-years, I’d say, most of the detections, which means that most of the planets we’re seeing only about 500 years back in time, which, on an astronomical scale, is pretty small.
Most of them are actually even closer than that, probably within 50-ish light years, so not very far away.
The time scales here, there’s one planet we know of, actually, that’s decaying onto its star.
Like, the orbit is shrinking and it’s falling onto its star, and it’s happening incredibly fast, which means in 3 million years, it’s gonna hit the surface of the star.
So 3 million years is incredibly fast for astronomers.
Yeah?
– Audience Member 6: So how big is it in parsecs?
– It’s probably about 200 parsecs, 300 parsecs.
I guess that’s a little bit more than 500 light-years.
Yeah, about 200 parsecs, I’d say.
There’s stuff much further out, but most of them are centered closer than that.
Yeah?
– Audience Member 7: Is AI going to be able to help you in your search?
– Is AI gonna be helpful in this search?
It’s funny you ask that ’cause I actually was just part of a very big proposal to do just that.
So there’s a bunch of ways.
I think the thing that got me really excited– and this maybe is a little inside baseball– but one of the fundamental limitations we have is that we have to compute– We get the observations and then we need to compute atmosphere models, right?
We get the data, but to actually figure out what’s in the atmosphere, we need to compute models.
We need to run these complicated– We effectively need to run a weather model on a computer, and we need to do that a million times to try and run all these different parameters.
And so the sorts of systems I’m working on typically take one to two to three weeks, two to three weeks to run on a computer.
And that’s on, like, a supercomputer– it’s actually at Arizona State– but it takes a long time to run, and so one of the things we’re particularly looking at is how can we use AI to speed up that process ’cause that’s only a one-dimensional model.
We’re not doing any sort of latitude/longitude modeling.
We’re just doing straight up and down, and running it out to a 3-D model, like we would have on the Earth, would take– you know, it would take months to run one of these things, and we’d need to run 10,000.
So one thing that I’m interested in looking at is how do we use AI machine learning to try and speed that up and get it to run faster, but right now, that’s just an idea.
Nobody’s actually done it yet.
Yeah?
– Audience Member 8: Do you anticipate getting beyond the infrared look and closer to the microwave look as far as the radio waves?
– Yeah, so are we thinking about moving into, like, microwave or radio waves?
So there are people, actually, who do that now, but they don’t– They use radio and microwave and millimeter waves to– They don’t look at planets, but they actually look at protoplanetary disks, so the stuff that forms planets, and there are beautiful images taken by an observatory called ALMA which just started operating a couple years ago in northern Chile to observe protoplanetary disks, and you can see– Actually, it’s amazing.
Guys, just Google search, like, “ALMA protoplanetary disk.”
Remember that and Google search it ’cause it’s, there are these disks of material, and then you can see there are rings.
There are gaps in the disks.
Those are planets that are forming that have sucked all that material and that ring onto them.
Like, that’s Jupiter that’s pulled all that material onto it.
And you can’t see the planet, but you can see all the gas missing where the planet must be forming.
And it’s these amazing images– There’s a woman, actually here at UW, Coco Zhang, who does this, so that’s a big part of understanding planet-formation processes.
That’s a whole ‘nother thing that I could talk forever about, is trying to figure that out, but, yeah, that’s a big part of it as well.
All right, oh, we got one more, yeah?
– Audience Member 9: I was wondering, on the original red disk that it said, out of the 5,761 exoplanets that have been discovered, what is the percentage of systems that you scanned that actually come up back and have an exoplanet within there?
– Oh, so, yeah, so how many stars do we need to look at before we find a planet?
Yeah, so that was my PhD thesis, was finding exoplanets and running a planet-detection survey.
That’s why I’m particularly bitter about not being able to name them after myself, because it was specifically my PhD– I actually asked my PhD advisor if we could do this, and he just was, like, “Under no circumstances can you name this after yourself.”
So the answer is, we probably looked at… We looked at about 10,000 stars before we found one.
But, right, but there’s a lot of what are called observational biases that go into that, right?
So that doesn’t mean that one star out of 10,000 has a planet.
It just means it’s very hard to find transiting planets ’cause we were looking for very big ones, which are pretty rare, very close-in ones, which are pretty rare, and they needed to go right across the star, which is pretty rare, right, so there’s a lot of things working against us when we do that search.
When people have tried to correct for that and say, “Okay, we saw one out of 10,000, but, you know, “we’re only gonna see one out of ten just because of the angle probabilities,” what’s the actual rate?
Our actual guess for, say, how many habitable, Earth-like planets are around an average star, the current best guess is about one-half to two, meaning that we think that, on average, every single star like the Sun has a planet like the Earth pretty close to its habitable zone, right?
It seems like planet formation happens, and it happens all the time, and it goes, and it makes a lot of planets around most stars.
So it’s hard to find them, but that’s sort of because they’re hard to find, not because they’re rare.
– Audience Member 10: What would make that job easier?
– What would make that job easier?
Uh… bigger telescopes.
Well, no, actually, you know what would make the jobs easier?
If the stars cooperated, ’cause, man, let me tell you, like, the Sun, so to detect the Earth around the Sun, the velocity you’re measuring is about this fast, right?
I am walking at about two meters per second, one meter per second.
That’s about how much the Earth makes the Sun move in speed, but you’re measuring this speed over the course of a year, right?
The surface of the Sun is burbling all the time.
It’s just sort of flexing a little bit from solar flares and all sorts of things, right, and the Sun is doing its own, like, processes inside it.
And so the surface is moving, and you can imagine that measuring the whole Sun moving at this speed is pretty difficult if the entire surface is just flexing and wringing and bending underneath you.
So if I could have anything in the world, I would tell the stars to just stand still for, like, two years so we can measure them exactly, and then if we could figure that out, then we could do it, and, actually, the sort of less joking answer to that is, there’s actually a lot of people working on ways to try and, like, separate those two signals and get down to the level where we can measure a planet like the Earth, but it’s a difficult problem to try and separate that out.
Yeah?
– Audience Member 11: When you actually designed the camera… – Mm-hmm, yeah.
Well, helped design, yeah.
– Audience Member 11: Did it have a lens cap on it?
– Did the camera have a lens cap?
It did not, no.
Actually, the thing that was gonna really get us was the sun shield on Webb because the whole thing, it needs to deploy out.
Well, I can’t have– If you look at it, a picture, there’s the mirrors, and underneath that, there’s this big– it’s almost a tennis court-size thing, and that’s what shields it.
All the cameras need to be kept very cool, like -200 degrees.
And so if the Sun is shining on it, you can’t keep it that way, so the sun shield is there to block out all the sunlight, but the problem is, the sun shield is very thin Mylar that rips very easily.
It’s designed to be deployed.
It’s, like, six layers that all pull out and then separate, and it’s designed to be deployed in zero G, so it’s very thin Mylar that’s designed to be deployed in zero G. It’s very hard to test on Earth, and I remember being in meetings where they were trying to test it in Long Beach, California, where the telescope was assembled, and they’d say, “Oh, yeah, they tried another test, “and just all the cables, like, “jumped out of their cables trays, and it stopped, like, halfway through,” and you could just see people around the table be like, “What are we doing,” right?
And the woman in charge of NIRCam, when I worked for her, I still remember her saying– She saw this, right, and was like, “Look.
“Nothing that any of us do right here “is gonna affect what happens with the sun shield, right?
“There’s a lot of very smart people “who know exactly how important this is, “who are working very hard to make sure it works right “the first time because we only get one shot at it, “and it’s not worth worrying about.
None of your worrying is gonna affect what they do,” right?
And one of the nice things about Webb was, over time, it was pretty delayed, but one of the nice things was that one of the reasons it was delayed is ’cause everybody wanted to make sure it worked the first time, and it did.
It was pretty amazing.
There was pretty much no problems during commissioning.
Sun shield deployed the first time, no problem.
It still sort of amazes me that we didn’t actually hit some sort of major problem during that whole process, but it all worked the first time just like it should’ve.
All right, well, thanks, guys.
This was fun.
[audience applauding]
Search Episodes

Donate to sign up. Activate and sign in to Passport. It's that easy to help PBS Wisconsin serve your community through media that educates, inspires, and entertains.
Make your membership gift today
Only for new users: Activate Passport using your code or email address
Already a member?
Look up my account
Need some help? Go to FAQ or visit PBS Passport Help
Need help accessing PBS Wisconsin anywhere?
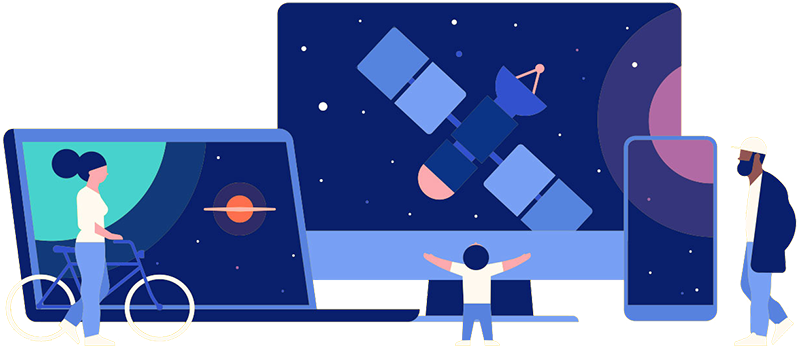
Online Access | Platform & Device Access | Cable or Satellite Access | Over-The-Air Access
Visit Access Guide
Need help accessing PBS Wisconsin anywhere?
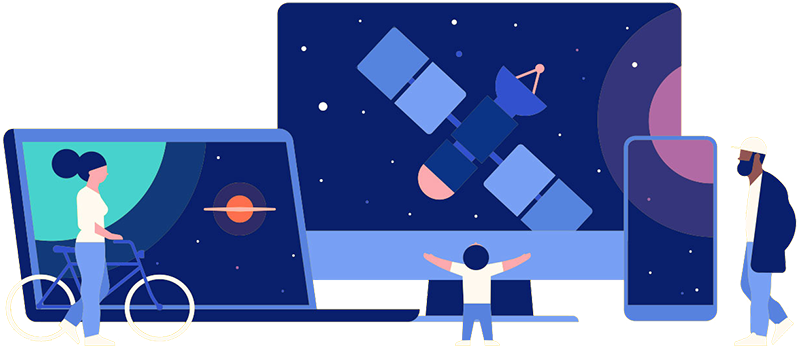
Visit Our
Live TV Access Guide
Online AccessPlatform & Device Access
Cable or Satellite Access
Over-The-Air Access
Visit Access Guide
Follow Us